INTRODUCTION
As a hardy grain, rye (Secale cereale L.) is grown extensively as a cover crop and forage crop throughout the world. It can be consumed by dairy cows and beef cattle in the forms of green chop, pasture, silage, or hay. In the livestock industry, forage quality is a critical factor affecting animal productivity. Ideally, an increase in the quality of the forage fed to an animal will improve animal performance and potential economic profit for farmers. Forage quality is commonly determined by various factors. Among these, forage species and cultivar are the most fundamental. Forage harvested at different stages of maturity also has different quality. Generally, forage quality decreases and dry matter (DM) yield increases as the plant mature, so the proper stage of maturity for harvesting should be considered carefully. The best harvest time is recommended for most forage crops in consideration of quality and productivity, but the actual harvesting is done according to weather and farm conditions [1].
Ensiling and haying are the two most common forage preservation methods for farmers. Hay is more marketable than silage, but the process of haying is more difficult to mechanize [2]. Farmers select a preservation method according to their requirements and the weather conditions. For example, in Korea, silage is preferred because haying is difficult to manage due to the predominantly wet climate during the hay making period [2].
The functional material, β-carotene, the most active biological form among the carotenoids, plays a significant role in forage nutrition as a precursor of vitamin A, a critical fat-soluble vitamin [3]. It has great benefits to both human health and livestock. Vitamin A cannot be synthesized by an animal and must be obtained from cleavage of pro-vitamin carotenoids from the diet [3]. Additional supplements of β-carotene can help meet the vitamin requirement. As many studies have claimed, β-carotene and vitamin A are necessary for the reproduction capacity and immune function of dairy cows [3–5]. For beef cattle, a study has shown that levels of β-carotene and vitamin A influence beef quality through effects on lipid stores and adipose tissue hardness [5].
Many studies have conducted on the appropriate harvest time of small cereal crops. As a result, forage crops are the recommended harvesting times from boot to soft dough stage [6–8]. Therefore, this study was conducted to determine the effect of harvesting time on the β-carotene content and the quality of silage and hay of rye.
MATERIALS AND METHODS
The experiment was carried out in an experimental field of Seoul National University, Pyeongchang Campus (located at 37°32ʹ46.1ʺN, 128°26ʹ17.9ʺE, 600 m absolute sea level [ASL]) from September 30, 2017, to May 31, 2018. The annual mean temperature is 11.5°C, average annual precipitation 113.4 mm, average annual wind speed 1.1 m/s, and the average annual humidity is 68.0% (Sin-ri, Pyeongchang, Korea).
After being harvested on April 30 (early boot), May 9 (heading), and May 15 (blooming), rye herbage was chopped into lengths of approximately 2–3 cm. Subsequently, the rye was exposed to different wilting time treatments: 0 h (no wilting), 6 h of wilting, or 24 h of wilting. Then, the chopped rye materials were ensiled into 20-L mini-silo (Plastic Jar, Samwon Chemical, Busan, Korea) without additives and sealed tightly with lids. Three replications were performed for each treatment. The 27 mini-silos were preserved in a dark, dry, ambient-temperature environment for 60 days before opening. To measure DM loss, the wet and dry weights of the silages before and after ensiling were determined using an electronic scale.
Rye hay was made in triplicate after harvesting on April 30 (early boot), May 9 (heading), and May 15 (blooming). The cut down rye was tedded twice each day to shorten the drying process. During the drying process, DM content was checked and recorded twice each day (9:00 and 17:00). After DM content increased more than 80%, the hay was packed into a nylon-net bag (40 × 80 cm, ~2 kg, Doo-il Chemical, Incheon, Korea) and preserved at ambient temperature for 70 days. During storage, variations in the rye hay and air temperatures were recorded at 17:00 every five days using a dial probe thermometer (Daewon Instrument, Seoul, Korea) with a 50-mm diameter dial and 300-mm length stainless probe. After storage, DM loss was calculated.
Approximately 300 g of each sample was collected and then dried in a 65°C forced-air drying oven for 72 h for determination of DM content. The dried samples were subsequently milled using a Willey mill with a 1-mm screen into screw-top plastic bottles and preserved at 4°C in a dark, dry storage room until analysis. Acid detergent fiber (ADF) and neutral detergent fiber (NDF) were measured by the method of Van Soest [9]. Crude protein (CP) was determined via the Dumas method, as described by Jean-Baptiste Dumas [10]. Total digestible nutrient (TDN) and relative feed value (RFV) were calculated by the formulae described by Holland et al. [11]. TDN was calculated from the ADF value (TDN% = 88.9 − 0.79 × ADF%), and RFV was estimated through digestible dry matter (DDM) and dry matter intake (DMI) as RFV = (DMI% × DDM%) / 1.29. The two-stage technique [12] for in vitro dry matter digestibility (IVDMD) analysis was used.
All silages were opened after 60 days of fermentation. Two subsamples were retained for further analysis. One subsample (about 500 g) was dried at 65°C in a forced-air drying oven for 72 h and then used to determine DM content and other chemical compositions, including ADF, NDF, CP, IVDMD, and water-soluble carbohydrate (WSC). Another subsample (about 700 g) was stored at −80°C in a refrigerator and subsequently used for sequential determination of silage acidity (pH), organic acids, microorganisms, and ammonia nitrogen. The Flieg’s score of the silages was calculated by the formula presented by Zhang et al. [13]. Silage pH was analyzed using a pH meter (AB 150, Fisher Scientific International, Pittsburgh, PA, USA). Lactic acid, acetic acid, propionic acid, and butyric acid were determined by high performance liquid chromatography (HPLC; Agilent Technologies, Santa Clara, CA, USA). Ten grams of fresh chopped silage sample mixed with 100 mL distilled water into 250 mL conical flask. Shaking for one hour on shaker and then stored at 4°C for 24 hours. The extracts were filtered through filter paper (Whatman No. 6, Whatman International, Maidstone, UK) and retained in −20°C refrigerator. Before analyzing, thaw the sample. Take 1.5 mL of filtrated material and centrifuged at 1,008×g, 4°C for 15 minutes using Centrifuge Smart 15 (Hanil Science, Dajeon, Korea). The supernatant (700 uL) was taken from sample solution with a syringe (KOVAX-SYRINGE 1 mL) and syringe filter (13 mm Syringe Filter, w/0.45 μm PVDF Membrane). The condition of instrument was shown in Table 1. For the silage, lactic acid bacteria (LAB), total microorganisms (TM), and viable fungi and yeast were counted by the spread-plate method [14]. Only fungi and yeast were counted for hay.
For β-carotene analysis, 2 g of the ground samples were used. The pretreatment is as follows [15]: put 10 mL of 6 % pyrogallol and sonicate in the samples, reacted for 10 minutes. After sonication, 7 mL of 60 % KOH was added in and mixed evenly by vortexing. Then the mixture was incubated at 80°C water bath for 1 hour and followed by 10 mL of 2 % NaCl and 15 ml of hexane-acetate, the ratio of which was 85: 15. Sequentially, the mixture was centrifuged at 2,800×g for 15 minutes. After centrifuging, added 1 ml of chloroform and the β-carotene concentration was detected by HPLC using Agilent 1260 series (Agilent Technologies, Santa Clara, CA, USA). The instrumental conditions were shown in Table 2.
Data were analyzed using the general linear model (GLM) procedure in SAS 2002 (version 9.1). The completely random design was used for the effect of harvest date on hay quality, and the split plot design was used for the effect of harvest date (main plot) and wilting period (sub plot) on silage. Differences were considered significant when p < 0.05.
RESULTS AND DISCUSSION
The effects of ensiling date and wilting on the chemical composition and feed value of the raw material and silage of rye are shown in Table 3 and 4. Chemical compositions were affected by the maturity stage at harvest of the grass silage within each growth cycle [16]. Advancing maturity of ensiling decreased CP from 137.41 g/kg DM to 86.92 g/kg DM (p < 0.05), similar to the result observed by Muck et al. [16]. The effects of harvest date on ADF and NDF were increased significantly (p < 0.05) with delayed harvesting date. The lowest ADF and NDF contents were harvested on April 30 (boot) with wilting for 24 h, which ware 267.37 and 476.50 g/kg of DM, respectively, and the highest content were 449.27 and 678.73 g/kg of DM, harvested on May 15 (blooming).
For IVDMD, significant increases (p < 0.001) were found on April 30 (boot) and May 9 (heading). Delay of the ensiling date decreased the digestibility of the rye silage, as shown by the values of 807.26 g/kg on April 30 (boot) and 633.91 g/kg on May 15 (blooming) in this experiment. A similar result for Italian ryegrass (IRG) silage wilted for 5 h was found by Kim et al. [17].
The content of TDN was reduced significantly (p < 0.001) by the delay of ensiling date; the TDN on April 30 (boot) was the highest, and that on May 15 (blooming) was the lowest. RFV was affected by the different wilting treatments (p < 0.01) in this experiment, and the effect of ensiling date on RFV was also significant (p < 0.001), as delay of harvest decreased RFV.
The fermentation characteristics of the rye silage are shown in Table 5. Extending the wilting time elevated the silage pH. This was consistent with previous studies [18], which indicated that wilting resulted in a significant increase in the pH of grass silage (p < 0.001). In the current study, the lowest pH value was found on May 9 (heading) when ensiling was done immediately without wilting. Silage ensiled on May 15 (blooming) showed higher pH values than the earlier stages. However, McDonald [19] reviewed that with DM content increase, silage fermentation is restricted, so the pH value is not always a reliable parameter for determining silage quality because with higher pH, stable conditions may be achieved at a much higher pH value.
1) 0 h, no wilting before ensiling; 6 h, wilted for 6 hours before ensiling; 24 h, wilted for 24 hours before ensiling.
The DM content of silage was closely related to the DM of the raw herbage, as wilting produced a significant (p <0.0001) increase in DM after ensiling. In addition to the effect of harvesting date, the silage that was ensiled on May 15 (blooming)with 24 hours wilting was highest at 308.12 g/kg DM, and the lowest DM content, 153.43 g/kg, occurred for silage ensiled on April 30 (boot) without wilting. The ensiling stage, wilting treatment, and interaction of both influenced the DM content of the silage significantly (p <0.05).
For April 30 and May 9, wilting significantly decreased the DM loss of silage after ensiling for 60 days. For the blooming stage, the DM loss of silage wilted for 24 h was significantly lower (p < 0.0001) than those for 0 h and 6 h. Among the three ensiled stages, the heading stage showed the lowest average DM loss, 39.14 g/kg, followed by 83 g/kg for April 30 (boot) and 93.31 g/kg for May 15 (blooming).
Wilting prior to ensiling increased the residual WSC content in silage on April 30 (boot) (p < 0.001), similar to the conclusions of Gordon [20], but was contrary to the results of Kim [2]. Silage wilted for 24 h contained more WSC than that wilted for 0 h or 6 h at the heading stage, but there was no significant difference among the wilting treatments at the blooming stage (p > 0.05).
The NH3-N proportion of TN is an important factor that can reflect the extent of proteolysis after fermentation. NH3-N is produced through the decomposition of protein by Clostridium spp. in fresh materials [21]. To some extent, the NH3-N content in silage may reflect the silage fermentative quality, as the silage fermentation was poor when more of it was produced. Wilting decreased the NH3-N/TN ratio for the April 30 (boot) and May 9 (heading) dates. Gordon et al. [18] also reported that wilting prior to ensiling resulted in reductions in NH3-N (g/kg total N). In this study, the highest content occurred for May 15 (blooming) without wilting.
The organic acid composition of the silage is shown in Table 5. Lactic acid was the main acid product during silage fermentation. Higher lactic acid content indicates a more efficient conversion from WSC to acid. In this experiment, the highest lactic acid concentration was detected on May 9 (heading). This result differs from the conclusion reported by Kim et al. [22] that the lactic acid content of whole crop barley silage ensiled at the milking stage showed the highest value, perhaps due to the forage species and different experimental location. Another reason for the high lactic acid content in the May 9 harvest is the WSC content. According to this experiment (Table 4), the rye harvested at the boot stage had the highest WSC content and the lowest at the harvested May 15th. Although the WSC content of the rye harvested at the boot stage was high, poor fermentation occurred due to the high moisture content, and it is believed that the fermentation quality of the rye silage harvested on May 9 was better.
The lowest acetic acid content in rye silage was on April 30 (boot), and the highest was on May 15 (blooming) (p <0.001). Enterobacteria and heterofermentative LAB produce a weaker acid (acetic acid) and increase DM loss [23]. Silages dominated by acetic acid have higher DM loss and might be less acceptable for cattle than silages dominated by lactic acid [24].
The lowest propionic acid appeared in the silage on May 9 and the highest was the silage sealed on May 15 (p > 0.001). In addition, wilting and the interaction of date and wilting had no significant effect (p > 0.05) on propionic acid.
Butyric acid reflects the extent of clostridial activity during ensiling and is considered to cause poor fermentation. Silages with a high concentration of butyric acid may not be readily acceptable by livestock [24]. Wieringa [25] claimed that grass silage containing less than 5–10 g/kg DM butyric acid can be considered good–medium-quality silage. In this study, the butyric acid content of the silage ensiled on May 9 (heading) was the lowest, and that ensiled on May 15 (blooming stage) produced the highest relative to others (p > 0.001). No butyric acid was detected in any of the wilting treatment groups from May 9 (heading). Rye harvested on April 30 is considered to have produced a lot of butyric acid fermentation due to its high moisture content (p > 0.001). According to a study by Leibensperger and Pitt [26], the higher the moisture content, the lower the pH, in order to inhibit the butyric acid fermentation.
In the current study, with progress maturity at ensiling, the ratio of lactic acid to acetic acid (LA/AA) decreased (p > 0.001). As demonstrated by Jones et al. [27], the LA/AA ratio indicates the relationship bewteen homolactic and heterolactic fermentation.
According to Flieg’s score, all silage made on May 9 (heading) was considered as “good” grade; the silage wilted for 24 h showed the highest score. The silage made on April 30 (boot) was determined to be of the average grade. The only poor silage was the silage of May 15 (blooming) that was wilted for 24 h, which was considered to have poor fermentation quality. This may be due to poor weather conditions, such as rainfall, high humidity, and short insolation duration, during wilting.
The viable count of microbes in this experiment is shown in Table 6. LAB dominate fermentation during the ensiling process as soon as anaerobic conditions are established. Some strains of LAB produce bacteriocins, which can inhibit the growth of other microbes [28]. LAB was increased by wilting time for all the stages examined in this study. The heading stage showed more viable LAB than the other stages (p <0.0001). Only 3.85 Log CFU/g of FM was observed at the April 30 (boot) stage without wilting.
Fungi and yeast are aerobic microorganisms present in silage that can lead to spoilage during fermentation [29]. The average number of fungi & yeast was low in the harvest on May 9 and high in the harvest on May 15 (p <0.0001). Overall, the number of fungi & yeast was the lowest in the silage harvested on May 9 with 6 h wilting, and the highest in the wilting for 24 h silage harvested on May 15 (p <0.001). This might due to less proteolytic activity in silage at the heading stage, so even the LAB number was very acceptable. The silage qualities of May 15 were poor because of high fungi and yeast populations, which could lead to undesirable fermentation. The population of TM can reflect fermentation quality directly. In this study, the silage of May 9 (heading) produced the most microbes, and only 5.63 Log CFU/g of FM were produced in the silage of April 30 (boot).
As shown in Fig. 1, the ensiling date and wilting period had effects on β-carotene concentration. With the advance of ensiling maturity stage, β-carotene decreased from April 30 (early boot stage) to May 15 (blooming stage) (p < 0.001). Within the same ensiling stage, the extension of wilting time also decreased the β-carotene content (p < 0.001).
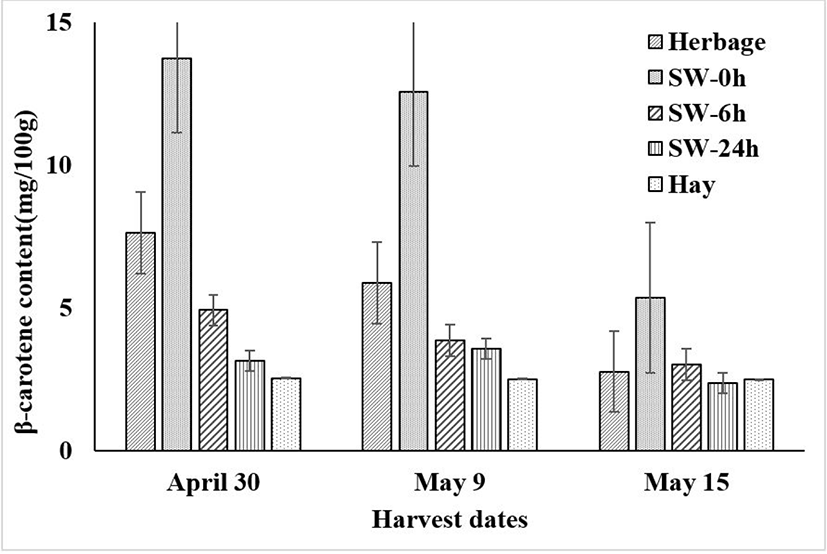
Generally, β-carotene content decreased after silage fermentation [30], and less than 20% of β-carotene might be lost in well-fermented silage [31]. However, in the present study, opposite results were observed, as the β-carotene content of the early boot and heading stages following ensiling increased by 80.08% and 114.51%, respectively, compared with that of the raw materials (Fig. 1). Similarly, increases in β-carotene content after ensiling were found in broccoli by-product silage in a previous study [32]. Therefore, it may be possible to synthesize β-carotene during fermentation, but the detailed dynamics remain unclear, and additional studies are required.
The temperature fluctuations of rye hay and air are shown in Fig. 2. Hay is usually baled at a moisture content < 200 g/kg, so the activity of plant enzymes is low. Thus, the spontaneous heat and DM loss during preservation are caused by microbial respiration [33]. The temperature of all of the hay made from three stages fluctuated slightly during preservation, and the air temperature mostly remained higher than the temperature of the three hays. Among the hays made on the three harvest dates, the hay from May 9 (heading) showed the lowest temperature throughout preservation; thus, it can be inferred that the lowest microbial activities occurred in hay from the heading stage, as confirmed by the fungi and yeast count (Table 7).
The chemical composition and feed value of the rye hay are shown in Table 5. DM loss of hay during preservation is due to microbial respiration. In this study, DM content increased with harvest date delay, as the highest DM occurred for the May 9 (blooming) date. The delay of harvesting for hay-making decreased DM loss markedly, from 167.68 g/kg (April 30) to 17.13 g/kg (May 15). In this experiment, the reduction of DM loss is determined as a result of low nutrient content and reduced incidence of fungi and yeast as the harvest is delayed.
The May 9 (heading) hay contained higher CP content than that of other stages after preservation (p < 0.001). The ADF and NDF contents showed similar trends, as their contents increased with plant maturity at hay-making (p < 0.001). The highest and lowest values were for May 15 (blooming) and April 30 (boot), respectively. Conversely, IVDMD content was decreased by the delayed harvest date of rye. The May 15 hay showed the lowest IVDMD, as well as the lowest TDN (p < 0.001). Stone et al. [34] concluded that, with plant maturity, the digestibility of cool-season grass hays decreased from 63.1% at the vegetative to 51.5% at the late bloom stage. The trend of IVDMD in the present study was consistent with that conclusion, but the numerical value was higher, possibly due to the different physiological conditions of the experimental cattle. Among the three harvest dates, the RFV values of the hay were significantly different (p < 0.05). Advancement of maturity at rye hay-making decreased the RFV. Stokes and Prostko [35] found that RFV decreased sharply with the advancement maturity in alfalfa hay.
Generally, microbial activity is inhibited and is low if hay is well dried. Under conditions of humidity higher than 70% and temperature higher than 20°C, significant fungal growth occurs in moist hays [36]. As demonstrated by Hlödversson and Kaspersson [33], bacterial populations remain stable within the first 2 or 3 weeks of storage, but the populations of several fungi increase in some moist hays. In this experiment, the population of fungi and yeast was larger, 4.91 Log CFU/g of FM, for boot stage (April 30) than for the later stages, although there was no statistically significant difference (p > 0.05) in viable fungi and yeast number between the rye hay of May 9 and the hay of May 15.
Fig. 1 shows changes in β-carotene content. With delay of harvesting, β-carotene content in the rye hay decreased from 2.53 to 2.48 mg/100 g. The levels of β-carotene in the grasses are very high in the young stages and reduce as the plant matures [37].
Compared with the raw materials, the β-carotene content of the hay made from the three stages decreased by 66.84%, 57.34%, and 10.14% after preservation, respectively. Carter [38] indicated that about 80%–90% of β-carotene can be lost in hay, which much higher than the values in the current study. The difference may attributable to the different plant species and experimental location. As shown in Fig. 1, hay-making resulted in more β-carotene loss than ensiling, indicating that ensiling is superior to hay-making for preservation of β-carotene, as mentioned by Ballet et al. [39]. For hay-making, harvesting of forage plants at late stages is superior to harvesting at earlier stages for β-carotene preservation.
CONCLUSION
When the harvesting date was delayed for ensiling, DM, ADF, and NDF contents increased, whereas CP, IVDMD, TDN, RFV, and DM loss decreased. Wilting increased DM content and pH values. Silage made on May 9 (heading) showed the lowest pH value, PA concentration, BA concentration, and fungi and yeast population, and also showed the highest LA content, LAB, TM population, and Flieg’s score, indicating higher fermentative quality compared with silage of the other stages. Wilting elevated LAB and TM populations, but it had no systematic effect on other fermentation products. Both advanced harvest date and wilting decreased β-carotene concentration. Taking all of the fermentation characteristics above into consideration, it can be concluded that ensiling around May 9 (heading) with 24 h of wilting can result in the highest quality of silage for rye.
For hay, delayed harvest date decreased DM loss, IVDMD, TDN, and RFV, but it increased DM, ADF, and NDF. Furthermore, CP content was highest for the May 9 (heading) date, and the fungi and yeast populations were significantly lower for May 9 and May 15 than for April 25 (boot). β-carotene decreased with plant maturity at the time of hay-making. Consequently, to achieve lower DM loss and higher quality of rye hay, making hay around May 9 (heading) is recommended.