INTRODUCTION
Winter rye is an important cold- and drought-tolerant cereal crop that can remain productive in less fertile lands with a wide range of soil pH. Moreover, winter rye has several ecosystem benefits such as prevention of soil erosion and elevation of soil microorganism activities [1–4]. As a silage crop, winter rye can be harvested earlier to achieve higher quality forage (like early heading stage); early harvest also allows for another crop to be planted in a double-cropping system [5]. However, the high moisture concentration of forage rye at the vegetative stage is unsuitable for ensiling because of excessive silage effluent, soluble nutrient loss, and potential clostridial spoilage. Those factors sometimes affect the rye silage quality [6,7]. Field wilting has been recommended to optimize the conditions for silage fermentation [8]. Wilting reduces moisture concentration in forage, minimizing protease activity, and seepage from ensiled forage [6]. Recently, Zhao et al. [9] noted that the population of lactic acid bacteria (LAB) increased when forage rye cut at the heading stage was wilted for 24 h, resulting in more desirable fermentation patterns during ensiling.
Despite the high concentration of soluble sugars in small-grain forages, the likelihood of producing high-quality silage can be increased by using biological and chemical additives, particularly when the forage dry matter (DM) concentration at ensiling is suboptimal [5,10,11]. Moreover, the hollow stems of winter forage rye may provide air space during ensiling and create aerobic environment in silo [12]. As a result, the use of additives is recommended to promote rapid acidification, resulting in suppression of unwanted microorganism growth at the early ensiling stages.
Sodium diacetate (SDA) is an FDA-certified preservative capable of inhibiting microbial growth and, thus improving silage fermentation quality [13,14]. Organic acid-based additives, such as SDA, are readily ionized to acetic acid and immediately acidify forage biomass, thereby suppressing the growth and activity of unwanted organisms during the early phase of fermentation and minimizing the loss of nutrients during ensiling [6,15,16]. In their undissociated form, organic acid salts can readily permeate the cells of yeast and mold, and release hydrogen ions within the intracellular region. This increases adenosine triphosphate (ATP) expenditure of unwanted organisms for maintenance of intracellular homeostasis, and thus disrupts their cellular metabolism [17–19].
Although the presence of epiphytic LAB on forage biomass surfaces can naturally initiate ensiling fermentation, microbial inoculants are usually recommended to support rapid acidification, which improves fermentative quality, and minimizes nutrient degradation during fermentation [20,21]. Previous studies identified that microbial inoculants such as Lactobacillus plantarum (LP) and L. buchneri (LB), individually or in combination, enhanced the fermentative quality of cereal grain and grass silages [22–24]. However, inoculation with both homofermentative and heterofermentative LAB has provided inconsistent results when tested at variable moisture levels [25]. A meta-analysis also concluded that the improvement in fermentation quality was achieved by crop-specific application of homofermentative or heterofermentative LAB [26]. These results indicate the necessity of additional information to clarify the individual or combined effects of the LAB inoculants on the fermentation dynamics of wilted forage rye at the vegetative stage.
Information is limited comparing the usefulness of chemical- and biological-based additives for enhancing the silage fermentation quality of wilted forage rye. Therefore, this experiment was designed to determine the effects of microbial inoculants, including LP, LB, and their combination, and an organic acid preservative (SDA) on the dynamics of the anaerobic fermentation and nutrient conservation in wilted forage rye through monitoring fermentation process.
MATERIALS AND METHODS
Rye (Secale cereale L.) was seeded in October 2019 at the experimental field of Pyeongchang Campus, Seoul National University (37°32ʹ46.1ʺ N, 128°26ʹ17.9ʺ E) in Republic of Korea. The temperature and rainfall during the growing season are presented in Fig. 1. Forage rye was harvested at the early heading stage (May 13, 2020) with a hand clipper. Five randomly chosen spots (1 m × 1 m) were harvested to estimate forage production. The forage rye was harvested at 6-cm stubble height and wilted on the field for 24 h, tedded in 12-h interval.
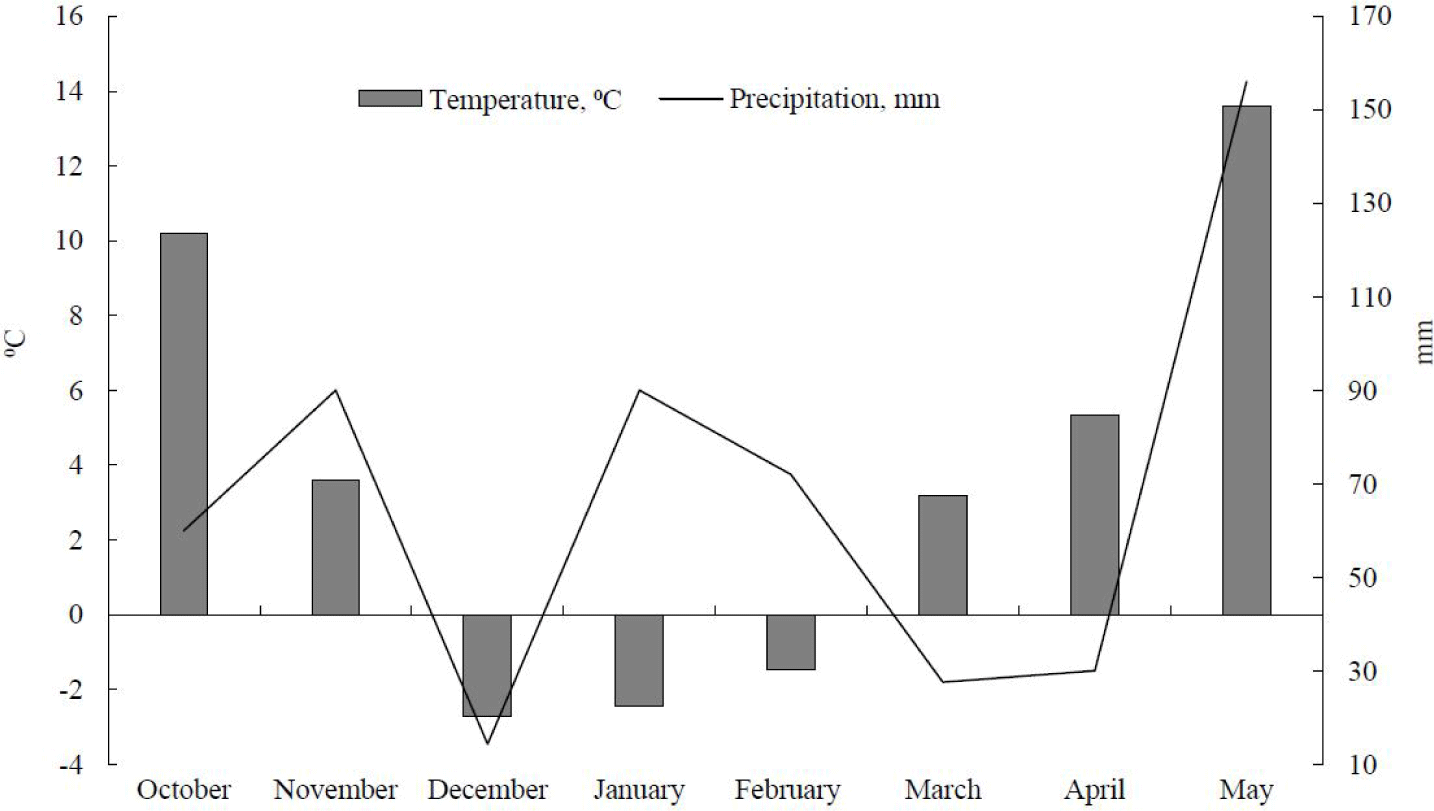
The wilted whole-plant rye was chopped into a theoretical cutting length of 20–30-mm using a forage cutter (Richi Machinery, Henan, China) and thoroughly mixed. The forage mass was divided into six equal portions, and randomly allocated to one of the following treatments: SDA3, SDA6, LP, LB, and LP + LB. The SDA (99%; Shanghai Rhawn Chemical Technology, Shanghai, China) was applied at rate of 3 (SDA3) and 6 g/kg wilted weight (SDA6). The microbial inoculants were LP (NLRI-101, LB (ATCC4005), and the combination (LP + LB; 1:1 ratio) of the two lactobacilli. The application rate was 1 × 106 colonial forming unit (CFU) per g wilted mass. A control treatment (without any additives) received 10 mL distilled water per kg wilted biomass. Other five additives were sprayed uniformly on forage mass with dispensing the same volume of distilled water as the control. After manually mixing, a 400-g mass of forage was packed in a plastic vacuum bag (Food grade, 28 cm × 36 cm, Korea), sealed by a vacuum packer (FM-06, Aostar, Seoul, Korea). All the silage bags were stored at 20°C–22°C. The 0-day ensiling was sampled immediately after the preparation of all treatment silages. The stored silage treatments were opened at 1, 2, 3, 5, 10, 20, 30, or 45 days of ensiling. The sampling days were set according to Santos et al. [27]. At each sampling day, the whole ensiled biomass in a bag was mixed thoroughly and split into three equal amounts for later analyses. The first portion was immediately frozen (–80°C) for later analysis of ammonia-nitrogen (NH3-N), lactic acid, and acetic acid. The second portion was kept fresh for immediate measurement of pH, LAB, mold, and total microorganisms. The third portion was weighed fresh, dried in an oven at 65°C for 72 h for determination of DM and feed values.
Silage extract was prepared to determine the fermentation profile of rye silage [28]. The acidity of the extract was determined immediately after opening the silo bags with an AB 150 pH meter (Fisher Scientific International, Hampton, NH, USA). Quantification of NH3-N was undertaken using a UVIDEC-610 spectrophotometer (Jasco, Tokyo, Japan) [29]. Lactic acid and acetic acid were analyzed by HPLC (Detector, RI; Column, Agilent Hi-Plex H; Agilent Technologies 1260 Infinity, Santa Clara, CA, USA), according to the procedure specified before [30]. LAB, mold, and total microorganisms were counted by streaking agar plate method [31] with a detection limit of 2 Log10 CFU/g wet weight, as described before [28].
The dried samples were ground into 1-mm particle size using a cutting mill (Thomas Scientific, New Jersey, USA) to determine nutrient value of rye silage. Neutral detergent fiber (NDF) and acid detergent fiber (ADF) were analyzed sequentially [32] by adding sodium sulfite and α-amylase. Analysis of total N was performed using an elemental analyzer (Euro Vector EA3000, EVISA, Milan, Italy) according to the Dumas combustion method [33]. Acid detergent-insoluble crude protein (ADICP) was determined according to Licitra et al. [34]. The anthrone method was used for analysis of water-soluble carbohydrates (WSC) [35]. In vitro dry matter digestibility (IVDMD) was determined according to Goering and Van Soest [36] using ANKOM DaisyII incubator (ANKOM Technologies, Fairport, NY, USA) [37]. Total digestible nutrients were calculated using the equation [88.9 – (0.79 × ADF %)] [38].
Data were analyzed by one-way analysis of variance (ANOVA), performed with the general linear model procedure of SPSS Statistics for Windows (version 24.0, IBM, Armonk, NY, USA). The model used for analysis was Yij = μ + Ti + Dj + (T × D)ij + εij, where Yij = observation, µ = mean, Ti = effect of treatments (silage additives), Dj = day of ensiling, (T × D)ij = interaction effect of treatment × day of ensiling, and εij = error term. The experimental unit was the individual ensiling bag. Before analysis, all data were tested for normality and equal variance (t-test); no outliers were identified and the data were normally distributed. If treatment effect was significant at p < 0.05, the mean differences between treatment pairs were determined by Duncan’s multiple range test.
RESULTS AND DISCUSSION
Heading stage is the optimum stage to forage rye because cude protein (CP) and other nutritive values decline rapidly after this stage [7,39]. Wilting forage rye for 24 h resulted in an increase of DM concentration to 239 g/kg (Table 1). Zhao et al. [9] also noted that the DM concentration was only 165 g/kg when rye was harvested at the heading stage. Therefore, 24-hour wilting could increase DM concentration at a rate of 0.5 percent unit per hour to around 284 g DM/kg. Kim et al. [7] also reported an increase in DM concentration from 178 to 418 g/kg when wilted for 24 h. The wide variations in forage wilting from the other research indicated climate-related factors, namely precipitation, temperature, and wind speed, can significantly influence wilting [40]. Therefore, wilting the rye harvested around this growth stage is advantageous, considering the dry spring weather conditions in Korea.
1) Treatments were untreated silage (Control); SDA3, sodium diacetate applied at 3 g/kg fresh weight; SDA6, sodium diacetate applied at 6 g/kg fresh weight; LP, L. plantarum; LB, L. buchneri; LP + LB, L. plantarum + L. buchneri (1:1 ratio). Application rate of inoculants was 1 × 106 CFU/g fresh weight.
Table 1 presents nutrient compositions and microbiological properties of the wilted rye forage treatments before ensiling. The concentrations of NDF and ADF in this experiment demonstrated substantial variation from the other study conducted with forage rye at the heading stage [41], possibly because of the differences in production management, such as seeding rate and harvesting time, as well as climate [42,43].
Consistent with previous reports on early-cut forage rye [5], the wilted forage rye in the present experiment had a substantial WSC concentration (143 g/kg DM). This concentration was greater than the recommended minimum range (60–80 g/kg DM) for lactic acid fermentation [44]. The epiphytic LAB count in the untreated wilted rye before ensiling exceeded the recommended count of 5 Log10 CFU/g to initiate normal silage fermentation [45].
The effects of additives and days of ensiling on silage pH, WSC, and NH3-N concentrations of the wilted rye are presented Fig. 2. Fast pH drop occurred at the early stage of ensiling (within five days), and then pH changes flattened throughout the rest of ensiling period (Fig. 2A). The pH of SDA6- and SDA3-treated silages showed steeper drops than LP, LB, and LP + LB. Previous studies on LB inoculation made pH of grass or small-grain silages higher than those of no additives [46], mainly because LB converts lactic acid to other metabolites such as acetic acid, ethanol, and 1, 2-propanediol, which cause the slight rise of the silage pH [47]. Contrarily, we could not confirm this pattern because the pH of the untreated silage was consistently higher than LB-inoculated silages throughout the monitoring period.
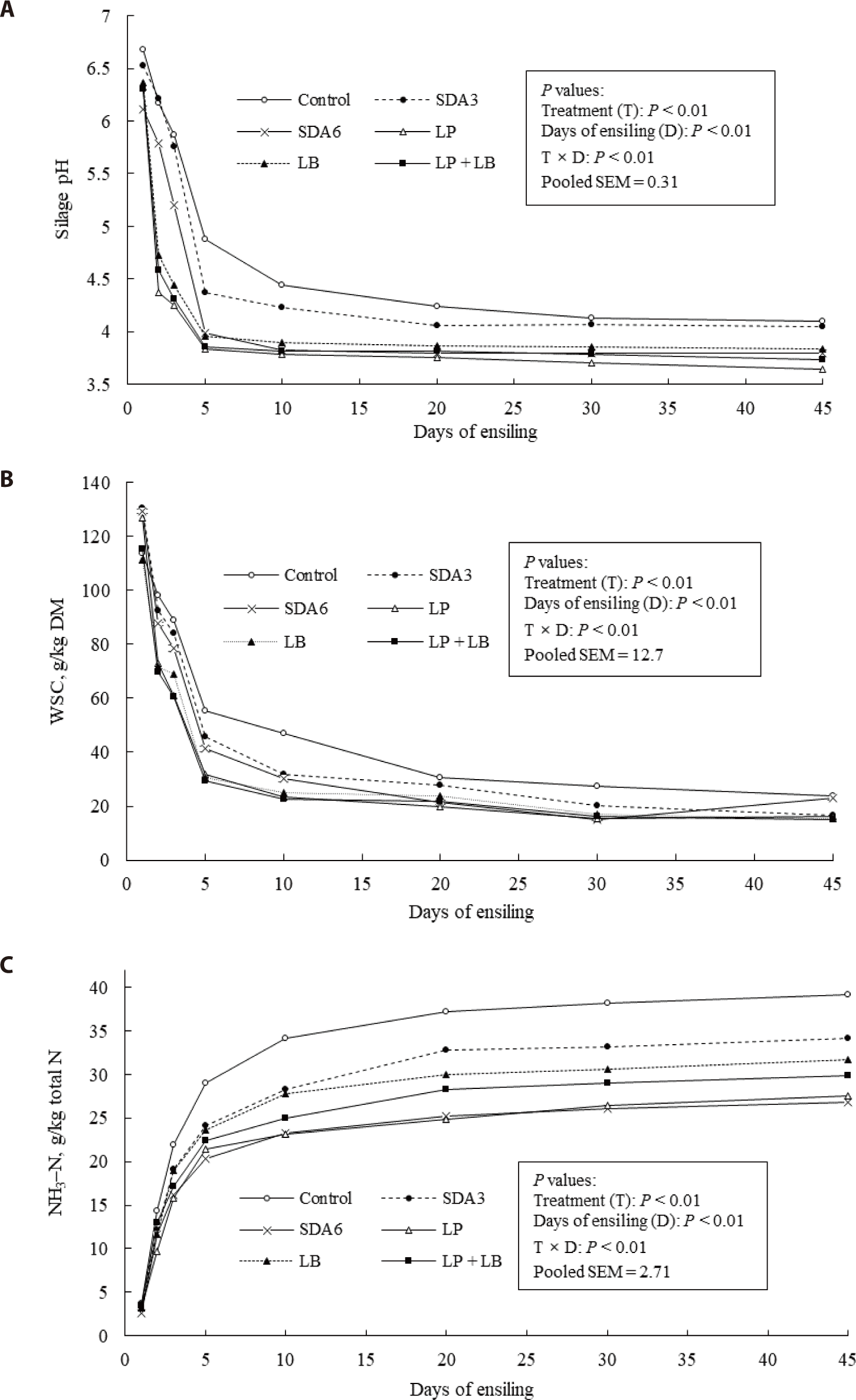
The final pH of silage with no additives was 4.10, which is much lower than the value of 5.72 reported by Paradhipta et al. [42] with the untreated forage rye harvested at the dough stage. This emphasizes the importance of harvest maturity for silage fermentation of forage rye. Changes in DM and WSC in winter forages of different maturity can influence microbial growth and silage fermentation [25].
The decline of WSC concentration occurred in all silage treatments, which was rapid during the first 5 days of ensiling. This pattern matched to the pH declines (Fig. 2B). Silages inoculated with LP, LB or LP + LB exhibited a faster rate of WSC decline during the first 5 days of fermentation than other treatments. Approximately 80% of the WSC in the wilted rye was utilized within 5 days of ensiling in LB, LP, and LP + LB treatments, which was significantly greater than other treatments. After 45 days, the residual WSC concentration was the greatest in the untreated and SDA6-treated silages. This suggests greater WSC utilization by the microbial inoculants. Decline of WSC during ensiling is usually due to conversion of fermentable sugars into organic acids, predominantly lactic acid by lactic fermenting bacteria [6]. Alternatively, WSC could also feed undesirable enterobacteria, clostridia, acetobacter bacteria, mold, and yeast, which results in a low lactic acid concentration silage. The insufficient acidify could not prevent the undesirable bacteria growth [6]. In this experiment, the faster decline of WSC in lactic bacteria inoculated treatments indicated higher lactic acid concentration and lower silage pH.
In all silages, the NH3-N concentration increased gradually as fermentation proceeded, and stabilized to the final phases of ensiling (Fig. 2C). The degree of NH3-N increase was generally greater in the untreated silage than those of treatments. At day 45 of ensiling, the NH3–N concentration was lowest in SDA6-treated and LP-inoculated silages and highest in the untreated silage. The protein degradation into various nitrogenous compounds during ensiling is inevitable. Especially, NH3-N is low in the nutritional value [48]. The formation of NH3-N is accelerated by enhanced activity of microorganisms that degrade true protein fractions into ammonia [6]. The NH3-N concentration should be below 100 g/kg total N in silages to be considered as desirable fermentation [6]. The NH3-N values in this study indicated that severe protein degradations did not occur during ensiling.
Within the early- days of ensiling, proteolysis occurred actively. The increase of NH3-N formation was slowest when treated with SDA6 and LP. At the early of the ensiling, a sharp decline of silage pH reduced enzyme activity and microbial proteolysis [49–51]. Protease begins to decrease its activity as the pH declines, with approximately 67% of the enzyme activity being lost within 24 h at the pH ranging from 4 to 5 [52]. Despite the lower silage pH of the LP + LB-treated silage than SDA6-treated silage, the NH3–-N concentration was relatively higher during the ensiling process of LP + LB-treated silage, possibly because of the antimicrobial properties at 6g SDA per kg wilted forage rye, which may be sufficient to suppress the aerobic microorganisms activities and enzymes involved proteolysis [48]. Zhao et al. [9] reported a substantially higher NH3–N concentration and higher pH of wilted rye than our study. The initial WSC concentration has been identified as a prerequisite for accelerating the pH decline, which is also related to lower NH3–N concentrations [6,53]. In this study, lactic acid concentration was higher and silage pH was lower than Zhao et al. [9], indicating the higher WSC boosted lactic acid fermentation and lead to a lower silage pH, decreasing protein degradation.
Lactic acid formation increased gradually with ensiling, with the highest increase rates within the first 5 days of fermentation (Fig. 3A). From day 0 to 3 of ensiling, lactic acid production was the highest in the LP treated silage, followed by LP + LB or LB, and the lowest amount with the SDA-treated silages. Lactic acid concentration in the SDA-treated silages increased slower than the inoculant treatments. Lactic acid accumulation reached its plateau between 10 and 20 ensiling days, and the concentrations in LP and LP + LB were the highest among the treatments at day 45 of ensiling. Lactic acid concentrations in the experimental silages exceeded the typical ranges (20–40 g/kg DM), because of the high moisture content of the wilted rye at ensiling (650–750 g/kg) promoting lactic acid formation during ensiling [54]. Previous research identified LP as a LAB strain whose main end-product is lactate, which is mainly involved in the rapid acidifications of silage after ensiling, resulting in early suppression of unwanted microorganisms and some fermentation end-products [23,55].
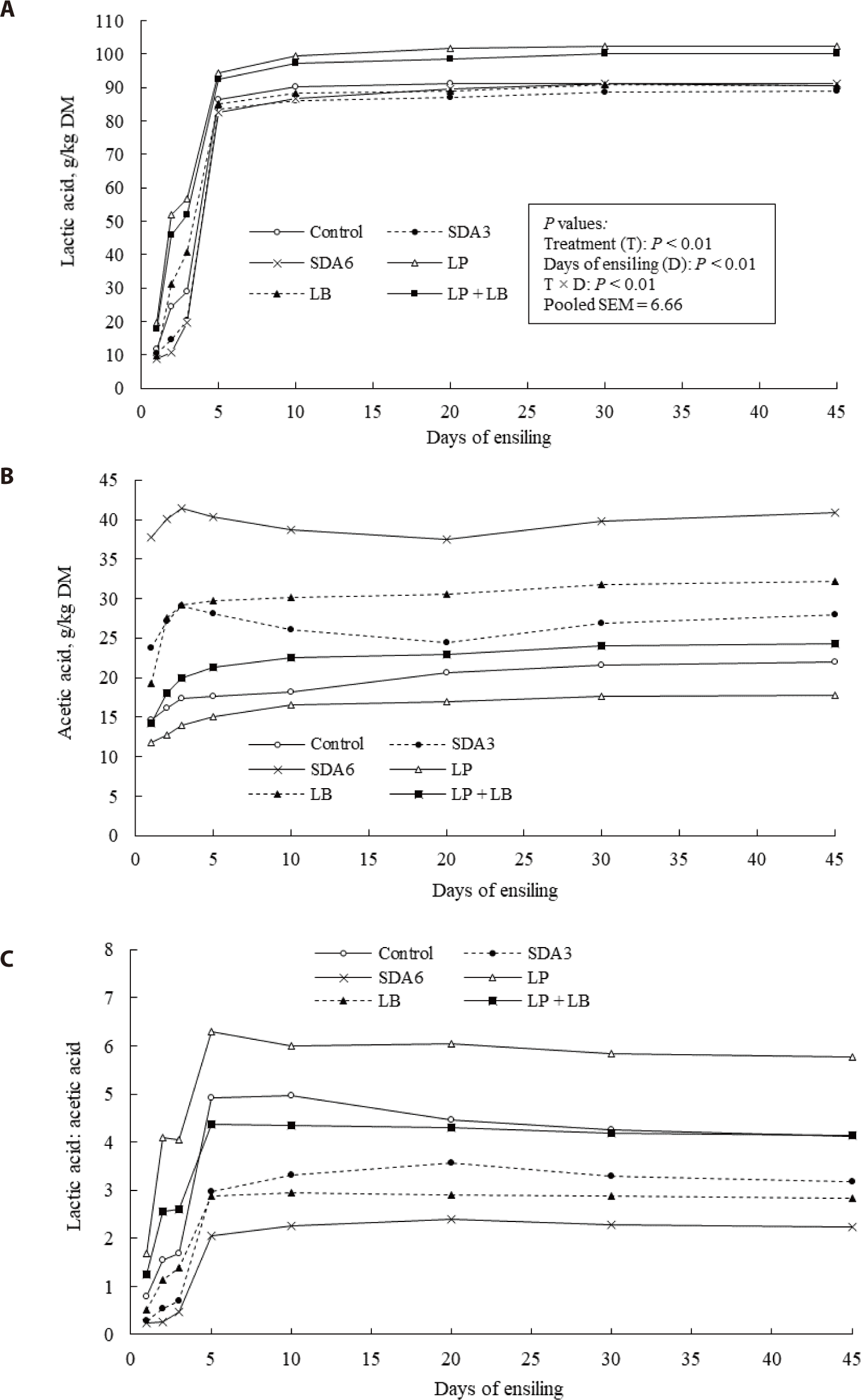
Acetic acid presented in the highest concentration when treated with SDA at the first day of ensiling (Fig. 3B). From day 2 to 5 of ensiling, acetic acid concentration increased gradually followed by a slight reduction, until no significant changes to the end of ensiling period. Acetic acid concentration in the LB-treated silages maintained at the typical range of 3%–4% as the previous report [54]. The substantially high acetic acid formation in LB-treated silage could be explained with the promotion of heterofermentative metabolism in this treatment [20,24,56]. Acetic acid concentration in this study was lowest in the LP-treated silage, which is consistent with the findings of Auerbach and Theobald [5], who reported higher lactic acid but lower acetic acid concentrations by LP inoculation.
All silage treatments showed an increase in the lactic acid to acetic acid ratio during the early phase of ensiling, which then decreased slightly until stabilized (Fig. 3C). This ratio was generally higher for the LP- and LP + LB-treated silages, reaching its maximum value after 5 days of ensiling. The untreated and LB-treated silages reached their peak ratio after 10 days of ensiling, while the SDA-treated silages reached their peak ratio after 20 days of fermentation. At day 45 of ensiling, the highest ratio (5.76) was seen for the LP-treated silages, while lower ratios were observed in SDA6- and LB-treated silages. A lactic acid to acetic acid ratio higher than 3 indicates dominant homolactic fermentation [49], and all the treatments except for SDA6 and LB demonstrated the ratio higher than 3.0.
In all silages, LAB multiplied rapidly and generally became dominant within the first 5 days of ensiling, especially in the inoculated silages, indicating that LAB was dominant in the fermentation microbial community (Fig. 4A). Generally, LAB counts reached their maximum on days 5–10 of ensiling, followed by a gradual decrease. For example, LAB counts in the untreated and SDA3-treated silages reached their maximum after 10 days of ensiling. At day 45 of ensiling, LAB counts were highest in the LB-treated silages and lowest in the untreated and SDA3-treated silages. The total microorganism population followed the same pattern as the LAB count throughout the fermentation process (Fig. 4B). Mold count declined with progression of ensiling, reaching an undetectable level in the additive-treated silages after 20 days of ensiling (Fig. 4C). Mold became undetectable after 45 days of ensiling in the untreated silage. However, molds reached to undetectable count level faster (day 10) in the silages treated with LP, LP + LB, or SDA6. In these silages, pH reduction to below 4 occurred within 5–10 days of ensiling (Fig. 2A). At low silage pH (~4), a greater proportion of acetate molecule exists in an undissociated form, which can easily penetrate the cell membrane of yeasts and molds and release hydrogen ions into the cytosol. This increases the ATP expenditures of the organism needed to maintain homeostasis, thus disrupting cellular metabolism [17,57].
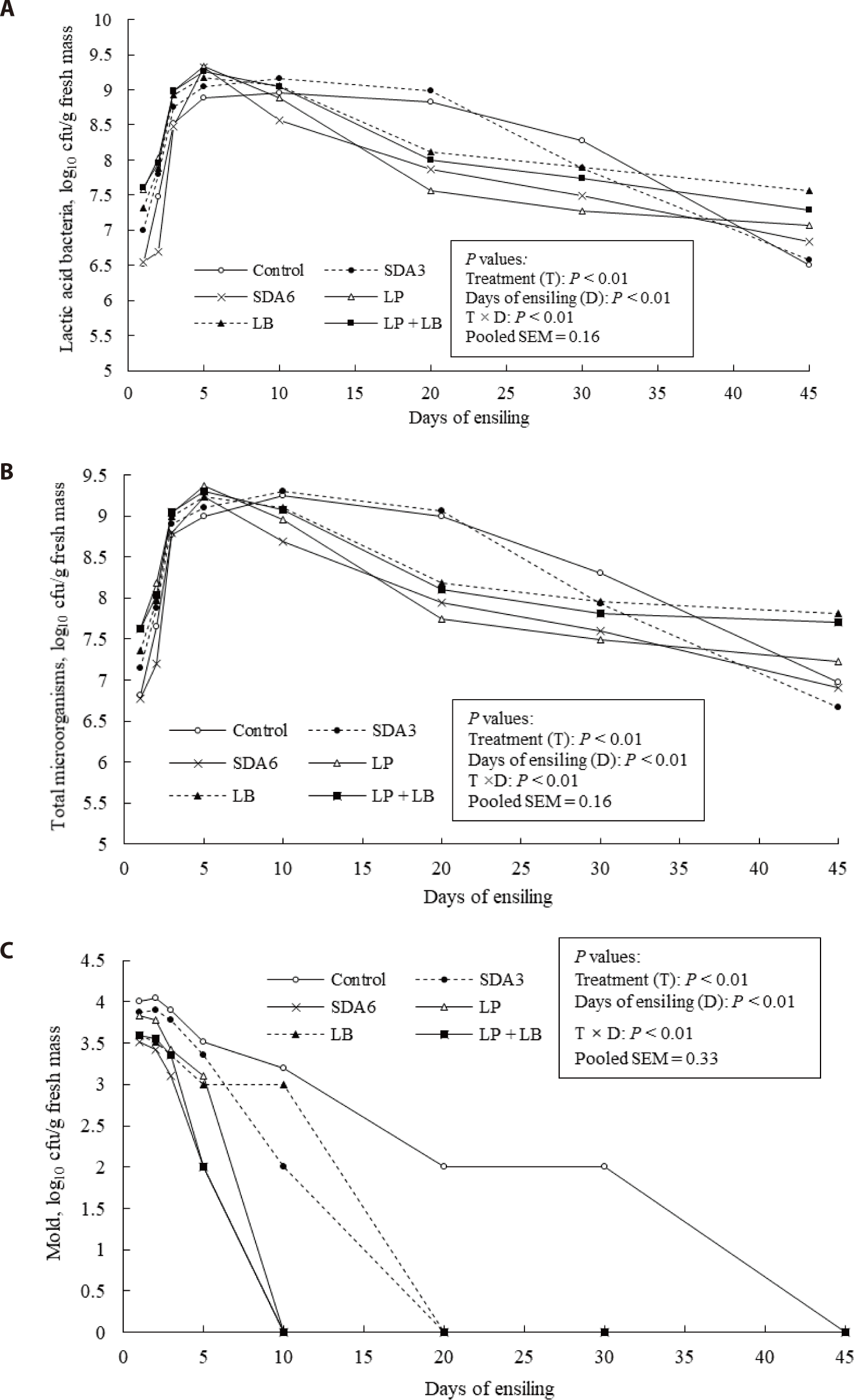
Yuan et al. [14] reported that SDA applied at 7 g/kg fresh weight had an inhibitory effect on both undesirable microorganisms and LAB development. This study also confirmed lower lactic acid production and lower count of LAB than other treatments when SDA was applied at 6 g/kg wilted rye. Wen et al. [16] also observed an initial delay in lactic acid formation compared to control silage when alfalfa (Medicago sativa L.) forage was treated with SDA (7 g/kg fresh weight), which was ascribed to the suppressive effect of SDA on LAB viability. The progressive decline of LAB with longer ensiling durations is likely linked to the excessively low silage pH and exhaustion of WSC, both of which suppress LAB growth [6,58]. Low silage pH suppresses the dominance of LAB, when the pH reaches 4.0 [50,59]. These could well explain the decline of LAB population in the later stage of ensiling in this experiment.
There was a gradual decline in DM concentration over the course of the ensiling process (Fig. 5A), likely because of the loss of organic matter to water and carbon dioxide under anaerobic conditions [6,58].
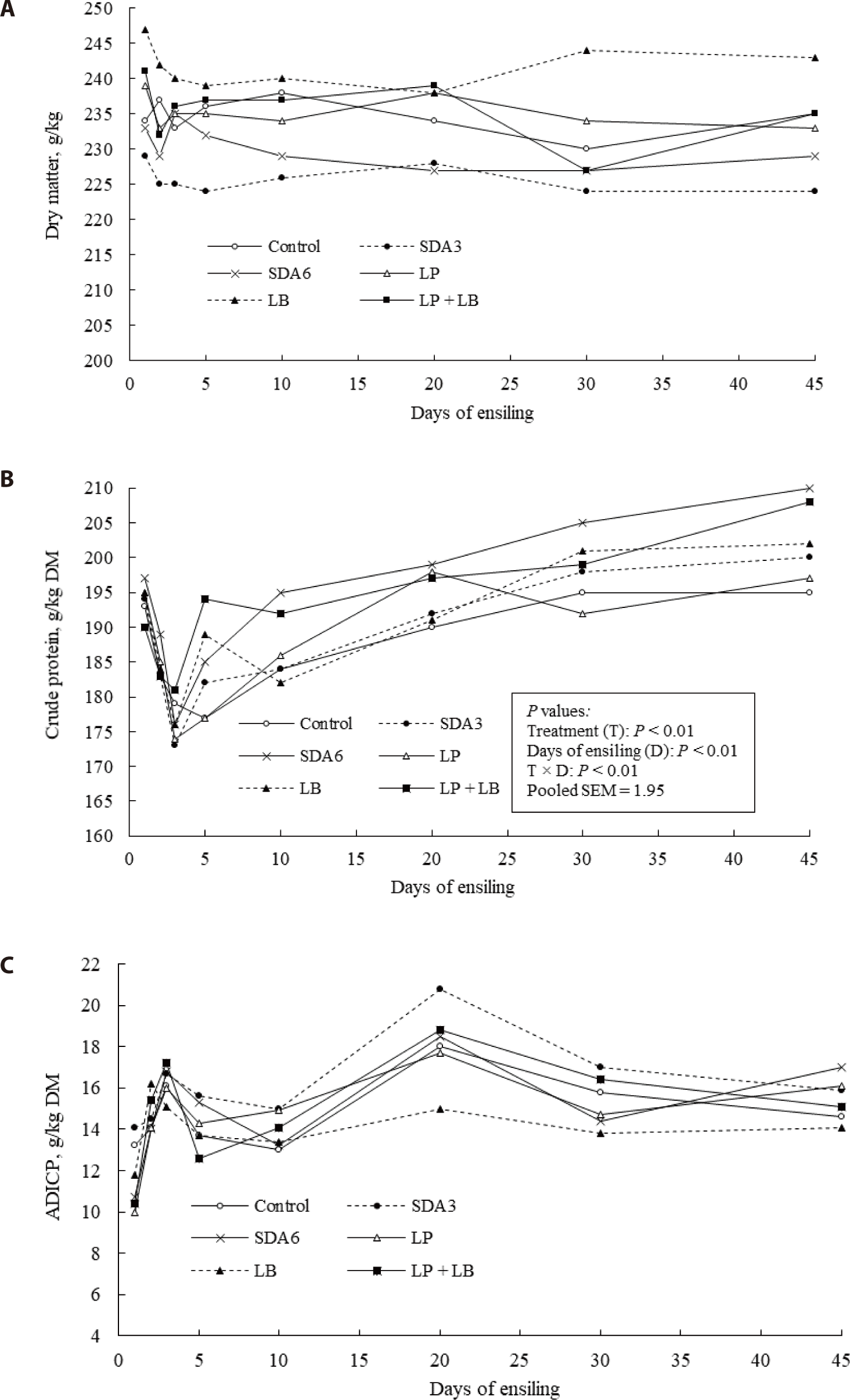
The decrease in CP concentration during the first 3 days of ensiling in this study (Fig. 5B) could be ascribed to the increased proteolysis caused by the activity of plant enzymes and existing microorganisms in the initial phases of ensiling [6,54,60]. Ammonia is a volatile compound and degradation of protein fractions into NH3–N, which particularly increased in the first 3 days of ensiling, may possibly explain the decrease of CP concentration [6]. After day 45 of ensiling, SDA6-treated silages had the greatest CP concentration while the lowest NH3–N concentration, implying lower loss of the protein fractions as ammonia by the microbial proteolysis. The lower NH3–N concentration in SDA6-treated silage indicated that SDA had significant effect in preserving forage proteins throughout ensiling. After 45 days, the average ADICP concentration in all silages was 76.5 g/kg CP (Fig. 5C), which is near the threshold of 75 g/kg CP reflecting normal silage fermentation [61]. The ADICP demonstrated significant variations among the treatments around day 20 and the variation in ADICP became narrow to a 3% range in the later phase of ensiling. A high concentration of ADICP is indicative of heat-damaged protein, which has low nutritional value for animals [62].
The effects of the additives and days of ensiling on TDN and IVDMD of the silages are presented in Table 2. The silages demonstrated inconsistency in TDN and IVDMD among the treatment across the progress of ensiling period.
1) Treatments were untreated silage (Control); SDA3, sodium diacetate applied at 3 g/kg fresh weight; SDA6, sodium diacetate applied at 6 g/kg fresh weight; LP, L. plantarum; LB, L. buchneri; LP + LB, L. plantarum + L. buchneri (1:1 ratio). Application rate of inoculants was 1 × 106 cfu/g fresh weight.
At the end of ensiling, the average IVDMD was highest in the silage treated with SDA6 (840 g/kg DM), followed by SDA3, and lactic bacteria inoculation treatments. The untreated silage was lowest. The difference in IVDMD across the silages treated with microbial inoculants was not significant (mean of 817 g/kg DM). There must be decrease of non-structural carbohydrates and this reduction resulted in the relative increase of structural carbohydrate proportions, thereby lowering TDN and IVDMD as ensiling progresses [63]. Some reports proposed possible hydrolysis of fibrous carbohydrates due to the impact of accumulated acidifying agents during ensiling fermentation [6,64], however, the inconsistency of the data do not support the theory.
CONCLUSION
Although existing conditions of the wilted rye may be sufficient to achieve lactic acid fermentation, the application of fermentation aids will carry specific benefits when the cool-season annual forage crop produces a large amount of wet biomass. As confirmed in this study, 24-hour wilting is marginally enough for moisture control. Also, the chances of unfavorable weather conditions warrant the application of silage additives to achieve enhanced forage preservation. For example, the application of SDA demonstrated its effectiveness in suppressing overall microbial activities while homolactic bacteria inoculation may help to boost lactic fermentation of the forage. Silage additives must be applied considering variable harvest environment and storage conditions.