INTRODUCTION
South Korea is one of the major hay importers due to its limited forage-producing land and rice (Oryza sativa) dominant agricultural background. Therefore, intensive domestic forage production has targeted maximum production of forage and nutrients per unit area, incorporating the available resources. One of the efforts to achieve these goals has been made through a double cropping system combing highly productive silage corn (Zea mays L) and winter annuals such as Italian ryegrass (Lolium multiflorum L.), cereal rye (Secale cereale L.), oats (Avena sativa L.) or barely (Hordeum vulgare L.) [1]. Silage corn has taken the main value in the forage cropping system because of the high energy concentrated biomass accumulation potential [2–4].
The Rural Development Administration (RDA) in South Korea categorizes silage corn cultivars into three maturity groups: early, medium, and late based on relative maturity or the days from planting to tasseling [5]. Since late-maturing cultivars potentially accumulate more biomass than earlier maturing cultivars due to the longer growing period, the RDA has prioritized medium to late-maturity cultivars in the national corn variety recommendations [5]. Therefore, the RDA recommends early planting of late-maturity corn to capture sufficient growing degree days (GDD) before harvest. However, the low soil temperature in early spring may cause inconsistent seed germination and result in low plant populations [6]. Soil temperatures higher than 10°C were recommended for early planting to establish corn seedlings rapidly to compete with aggressive summer weeds [7]. However, delayed corn plantings due to late spring utilization of cool-season annual forage may cause insufficient GDD for corn to develop maximum ear proportion in the biomass. The reduction of grain production due to late corn planting was also reported in Wisconsin, USA; therefore, corn planting should be done as early as possible when soil temperature and other soil conditions allow [8]. Corn establishment indicated earlier emergence of seedlings on coarse texture soil than on fine texture soil, which was because of higher soil temperature [9].
The models of the national weather service and GDD demonstrated potential for use as harvest decision tools in the US corn belt region [10]. The quantified parameters, such as soil temperature at planting (STP) and GDD, would be more robust than the calendar date for optimum management decisions. Although sufficient GDD is critical for corn to achieve full maturity of a variety, erratic weather and following forage planting readily complicate scheduled management. These conditions often reduce corn production and feeding value [11,12]. The leaf-to-stem proportions decline as the corn growth stage advances to the reproductive stage, while the ear accumulates highly digestible nutrients until the kernels’ black layer development stage [2,8]. Research has indicated that post-kernel milkline development is a critical indicator for silage corn accumulating greater energy value [13]. Corn silage harvested at a more mature stage than the black layer reduces intake and digestibility of the diet. The ear part of mature corn contains approximately three times more crude protein and digestible dry matter (DM) than stems [14,15], also comprising 30% to 50% of whole silage corn biomass [16].
Lactating cows produced more milk and milk protein when fed a diet containing the 2/3 milkline developing stage corn silage than a diet containing the early dent stage [17]. Therefore, the optimum windows for corn silage harvest are recommended between one-half and three-fourths of the kernel’s milkline development stages for the maximum forage biomass and digestible energy production potential [18–21]. Although this harvest window corresponds approximately with seven to fourteen days from the beginning of the dent stage [7], harvest date would be more practical when monitored through the physical development stage of corn and GDD rather than calendar dates because of the greater consistency of crop responses to local weather conditions.
Annual field trials have been conducted with newly adopted silage corn varieties and control varieties since the early 1970s to evaluate biomass responses to various planting and harvest management. Due to the different corn planting and harvest dates across the field trial years, data analysis should be conducted separately by days for planting or harvest. Response surface methodology (RSM) is a useful tool to determine the approximation of optimal response points related to multiple explanatory variables’ involved [22]. This statistical approach can assess long-term data variations affected by yearly growing conditions. Also, analysis of the 2-dimensional response surface patterns may reveal biomass sensitivity to multiple changing environments and management conditions such as soil and aerial temperatures. Baş and Boyacı [23] reviewed RSM to optimize and improve models with time-changing conditions. However, this statistical approach is only available when responses present a quadratic pattern.
Corn planting has been anecdotally recommended at the time of full bloom of plum (Prunus domestica L.) in South Korea between mid-April and early May [3,4]. However, yearly fluctuating weather and global warming conditions make some recommendations uncertain. Although optimum management for planting and harvesting has been conventionally based on calendar dates in South Korea, the timing of critical management should be provided through more robust environmental variables. Therefore, this study was conducted with silage corn trial data collected from independent field trials to quantify biomass responses to STP and harvest GDD.
MATERIALS AND METHODS
The silage corn biomass data (n = 188), including cultivar, trial year, cultivation location, planting date, harvest date, and biomass, were collected from the Research reports on livestock experiments operated by National Livestock Research Institute of South Korea between 1978 and 2018. The field trials conducted within the central region of the Korean peninsula between 35° 00’ 58” N, 126° 42’ 39” E and 37° 22’ 15” N, 128° 23’ 25” E were considered for the data analysis. The year average temperature of the region was 6.6°C–12.0°C, and the August daily temperature was 22.8°C to 30.6°C, the highest month. The annual rainfall ranges from 1,031.7 to 1,898.0 mm. Soils were very fine, mixed, mesic family of Typic Paleudalfs (Cutanic Luvisols [Epidystric Profondic Clayic Chromic]) in central east and fine silty, mixed, mesic family of Anthraquic Eutrudepts (Fluvic Hydragric Anthrosols [Eutric Oxyaquic Siltic]) in central west region.
The silage corn cultivars were grouped by relative maturities as less than 115 days for early, between 116 and 124 days for medium, and more than 125 days for late maturities. The corresponding STP in each trial were obtained from the weather database of the Korean Meteorological Administration. GDD of corn were calculated using 10°C as a base temperature. The GDD at harvest was calculated as the following equation.
where i is the corn planting date, and p is the harvest day.
The independent variables, such as STP and GDD at harvest, were obtained by converting planting dates and harvest dates into corresponding temperatures based on the recorded weather data. Then the converted data were sorted by trial year, location, and corn maturity. The experimental data was reviewed for any missing information for validation of the data. Trial data with missing planting dates, harvest dates, or maturity information were eliminated. Also, the data lack of a minimum of three harvest or planting date levels were also eliminated from the research due to inapplicable to the quadratic function.
Some field trial data were eliminated from estimating maximum biomass production because of insufficient cultivation information or unsuitable experimental designs. For example, field trials with less than three levels of STP and GDD treatments were not included because the design could not provide potential quadratic biomass production curve. When the biomass curves presented a downward convex function, maximum biomass was inestimable because of dispersions. Therefore, those trial data were also eliminated from consideration. The compiled trial data were arranged by maturity group, STP, and GDD. The corn biomass data of each group were analyzed considering STP, GDD, and the interaction between STP and GDD as explanatory variables. The random effects were years and replications within a trial. Test site effect within the central region were also considered as a random effect. The data from 188 trials were standardized through parallel movement to converge the projected biomass curves [24]. The regression analysis was conducted using Proc REG of SAS 9.4 with the corn silage data of each maturity group against STP or GDD, and finally, Proc RSREG was applied to estimate the biomass response surface for the optimum response ridge (SAS/STAT® 14.2).
The quadratic model was applied to each corn maturity group as follows.
where Mi,k,G is the maximum biomass of corn silage; G is the maturity group; β0k,G, βi,k,G, βii,k,G, and βij,k,G are the coefficients for constant, linear, quadratic, and interaction terms, respectively; Oi,k,G is optimum STP and GDD by a group; is residual; the moving transformations of STP and GDD.
RESULTS
Fig. 1 presents the average rainfall and temperatures of aerial and soil from 1978 to 2018 throughout the corn-growing months. The rainfall was lower in April and May through early June, then higher after mid-June. The rainfall reached its peak in mid-July. The heavy rainfall, high humidity, and perhaps strong wind are the typical monsoon weather in summer. The rainfall began to decline in August through September. The aerial temperature already reached above 10°C in early April. The daily mean temperatures rose consistently by early August, then declined to the end of the corn growing season. The soil temperature remained lower than the aerial temperature until mid-April, then became higher from mid-May to early September. However, the overall pattern was like the aerial temperature.
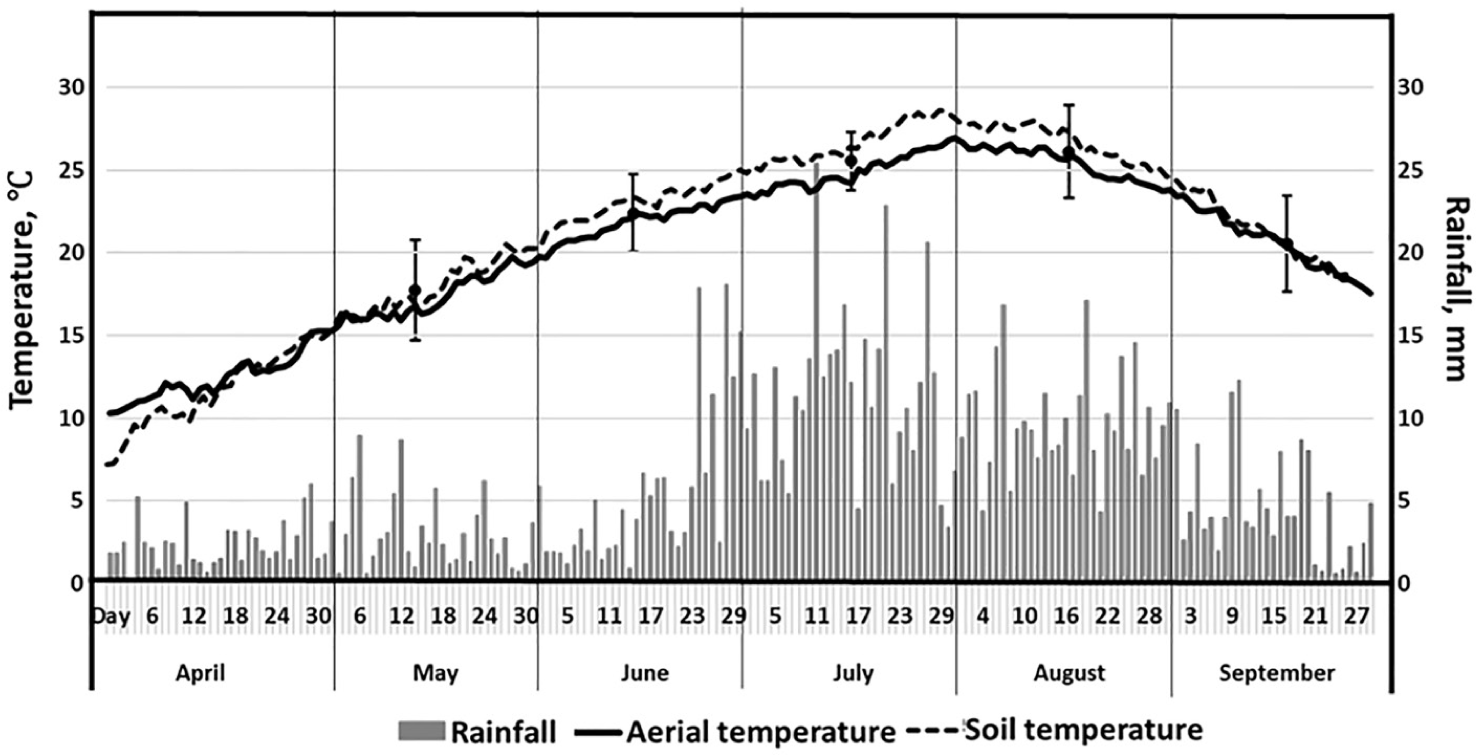
The STP, GDD, and biomass by the corn maturity group are presented in Table 1. The STP was similar between the early and medium maturity groups. However, the mean STP of the late maturity was one-degree unit lower than that of the early or medium maturity corn group, indicating that the field trials tended to plant late maturity corns earlier than other maturity corns. In response to the longer growing period, the mean GDD was greater for late-maturity corn than early maturity by 118°C. The number of field trials of early maturity corn was lower than those of medium or late maturities. The mean biomass differed among the maturity groups. The medium maturity group produced greater biomass than the other two groups. Varying weather conditions in the retained field trials probably caused inconsistencies in the corn group’’ mean STP, GDD, and biomass. The calendar days for the planting were mainly in mid-April in the trials, while harvest dates ranged from mid-August to late September.
The biomass data are presented in Figs. 2 and 3. The distributions of trial data were spread along the STP between 10°C and 20°C. Most trial data demonstrated convex upward patterns and presented maximum biomass points (Figs. 2A, 2B, and 2C). Compared with the STP, the distribution of biomass data by GDD trials demonstrated more dispersed patterns (Figs. 3A, 3B, and 3C), especially late-maturing corn (Fig. 3C). The biomass of early maturing corn was distributed mostly between 1,100°C and 1,800°C, while those of medium and late maturity corn were more widely distributed in the range from 500°C to 1,750°C, indicating that some trial data of these maturity groups are out of the reasonable temperature ranges.
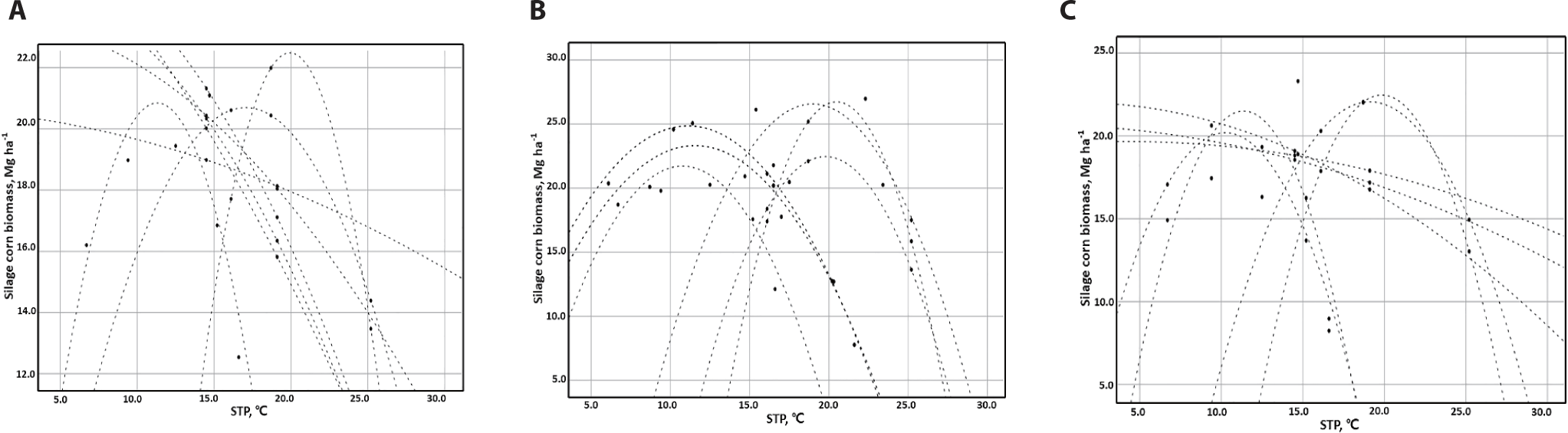
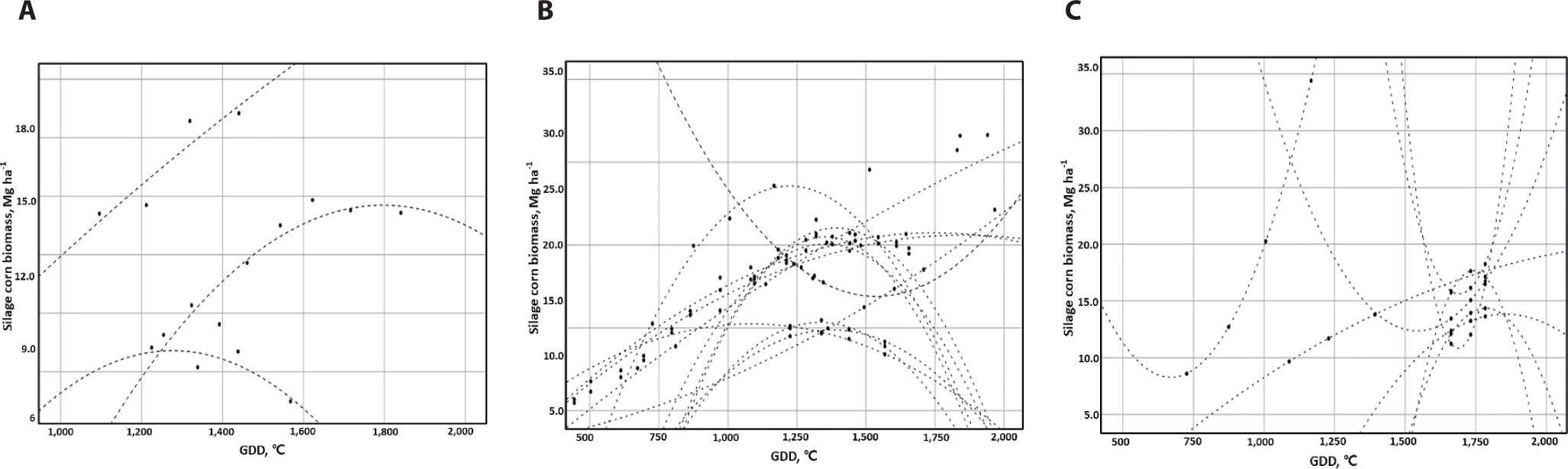
Since field trials were designed for STP or GDD, the corn biomass data were regressed separately against STP or GDD for each maturity group (Table 2). The coefficient of determination (R2) was lowest when the biomass of early maturity corn was regressed against STP. Those of the other two maturity groups were greater than 0.5. All the quadratic terms of STP were significant in the three maturities (p < 0.05), while the linear terms were insignificant.
The biomass responses regressed against the accumulated GDD were greater than 0.8 for early and medium. The R2 of the late-maturity corn biomass against GDD was the lowest among the group. The significance of quadratic and linear terms of GDD also presented similar patterns with the corn biomass regression against STP.
The corn silage biomass reductions from approximated maximum biomass with reduced STP and GDD were estimated for the maturity groups based on the regression analysis (Table 3). When the same degree of STP departures was considered, the greatest percentages of biomass reductions from the approximation of maximum corn biomass occurred in the late-maturity corn group. Although the biomass reductions by GDD were not as much as those by STP in the maturity groups, the biomass reduction percentage was greatest in the late maturity group.
The response surface analysis models were projected to approximate the maximized biomass surface by considering STP and GDD simultaneously (Fig. 4). The three maturity corns demonstrated different biomass change patterns with departures of STP and GDD from their values at the approximation of maximum biomass. The surface responses of late-maturity silage corn biomass were steeper than the other two maturity groups with the departures of the two independent variables. However, the impacts of STP and GDD on biomass reductions from the 3-dimensional biomass responses were difficult to quantify.
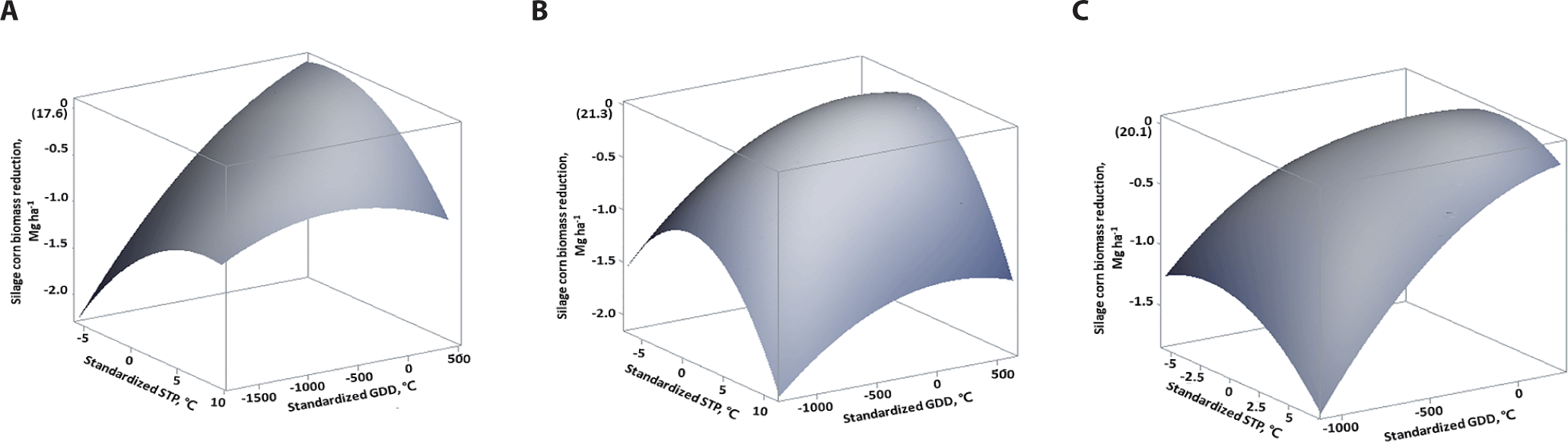
When considered for STP and GDD simultaneously in the regressions (Table 4), the R2 indicated substantial improvement of the model fits from those considered with STP or GDD separately. As the response surface analysis indicated, the regression coefficients were greater for the departures of STP than for the departure of GDD from those at the maximum biomass. The linear term of STP was only significant for late-maturity corn, while the quadratic terms were significant for all maturity groups. The linear GDD term was significant only for the medium maturity, while the quadratic terms were significant for the medium and late maturity groups. The interaction between STP and GDD was significant only for the late-maturity corn.
DISCUSSION
Due to the substantially different field trial conditions in the years and the trial locations, a data transformation method was adopted to standardize the various cultivation conditions. For example, different yearly weather conditions during the corn growing season caused the calendar dates to be less meaningful than actual temperatures. Therefore, the planting and harvest dates were transformed into the corresponding year’s STP or the accumulated temperature at harvest to be more robust across the field trials. However, some field trial data were eliminated from the modeling considerations due to the conflicting study purpose and experimental designs. The reduced trial data in the modeling considerations apparently diminished some differences in maximum biomass among the maturity groups. The trial data elimination caused the mean biomass of late-maturity corn not to numerically exceed that of medium-maturity for STP and the differences with the early maturity group to be insubstantial (Table 1).
The regression analysis indicated significant quadratic responses of corn biomass to the changing STP and GDD. Therefore, when the biomass reached its plateau, the STP and GDD were recognized as the optimum points for silage corn production. Furthermore, this approach could quantify the potential biomass reductions as the two management factors departed from the optimum points.
Because the planting date determines the accumulated temperature and corn growth development until harvest, the field trials were designed to determine the impacts of STP or GDD on the corn biomass, not both. The regression analyses demonstrated the consistent significance of the quadratic functions of STP and GDD, indicating that most of the trials included the planting or harvesting temperature ranges covering the maximum biomass in the corresponding field trials. When comparing the biomass data point distributions (Figs. 2 and 3), more biomass data points were located on the left side (pre-optimum GDD) than the right side (post-optimum GDD), especially in the late maturity group (data not presented). These uneven data point distributions indicate that more corn trials presented insufficient GDD, especially in the late-maturity corn. When the accumulated GDD departs from the maximum biomass point by 300 degrees, the production reductions were from 2.2 to 5.4 Mg ha−1, showing more biomass reductions in medium and late-maturity corns than in early-maturity corn.
The response surface method was an approach to evaluate the interactive impacts of STP and GDD on corn biomass by combining those two factors at the same dimension (Fig. 4), even though the impact of STP or GDD was investigated separately in the trials. As Kim et al. [24] demonstrated with sorghum-sudangrass (Sorghum bicolor L.) hybrid data, the method could present the impact of STP and GDD on sorghum biomass. However, as found with the data, interactions between STP and GDD were not confirmed in early and medium-maturity corn. Since the best fit of biomass reduction curves by the departures of management factors are considered symmetrical between before and after reaching the maximum biomass point, some attention should be exercised in reflecting the seasonal impact on silage corn biomass with the current biomass curves. This interpretation should count the different biological responses of corn plants as the growing season advances.
According to a study conducted in Missouri, USA, planting at around 15°C was promising to achieve uniform corn emergence within 21 days [6]. The current study’s modeling indicated maximum biomass production at around 16°C soil temperature. Besides biomass, ear development is critical in the energy content of corn silage. Therefore, even with the advantages of the rapid and uniform establishment of corn seedlings, delayed planting limits the period for accumulating total heat units for full ear development of mature corn. Early June planting lost forage biomass by 24% and total digestible nutrients by 28%, compared with the late April planting in central South Korea due to insufficient GDD [25]. Furthermore, Choi et al. [26] reported a decline in ear proportion from 40% in late April planting to 28% in late May in South Korea due to the increased chance of corn seedling infection by rice black-streaked dwarf virus (Reoviridae fijivirus).
The comparison of the obtained regression models for the three maturities indicated higher sensitivity of biomass reductions in the late-maturity corn than in the other two maturity corns with the departure of STP of 4°C, resulting in more than 20% reduction in late-maturity corn. In contrast, the reductions were only around 10% for the other two maturity corns (Table 3). As indicated before, the biomass of late-maturity corn is expected to be greater than that of the other two maturity groups when there is enough GDD for a sufficient growing period. However, the data did not support the superior biomass of the late mature corn. Probably, the corn was often harvested before reaching full maturity in the trials due to the planting of the following winter cereal forage crops. When the GDD departed from the optimum by 250°C, the biomass reduction of late-maturity corn was around 11.3%, more than those of early and medium-maturity corn.
During the corn growing season, the daily mean temperatures rarely fall into extremely cold (< 10°C) or hot (> 40°C) conditions in central South Korea, so there would be no noticeable weather stress for silage corn growth [19,20]. However, when the soil temperature is fixed at around 16°C, the estimated optimum GDD ranges from 1414 to 1565 in the corn groups. Therefore, earlier planting will be desirable for the maturity group to secure a sufficient growing period to produce maximum biomass and digestible nutrients. According to a corn maturity and biomass accumulation study, maximizing ear production assured maximum nutrient production in the early September harvest in South Korea [27].
As previously addressed, the main crop is traditionally rice in South Korea; land use and resources are limited for forage production. Contracted agricultural services accomplish the primary management for silage corn cultivation in the country, such as planting and harvesting, and the contractors schedule their services based on calendar dates. Therefore, biomass reduction due to departures from optimum STP or GDD would be probable in livestock operations in the country. In addition to the required STP or GDD, uneven rainfall distribution influences corn management. For example, about 70% of rainfall occurs during the summer monsoon season in mid-July and another short monsoon season in mid-August. This weather pattern is accompanied by strong wind and heavy rain, sometimes exceeding 7.6 mm hr−1 [28]. Considering rainfall required for corn cultivation around 450 to 600 mm [29,30], the concentrated rainfall throughout summer and fall is challenging in corn silage harvest [31].
Because silage corn has greater biomass and energy production potential than the following cool-season annuals, the forage cropping system on limited land has focused on corn silage production [1]. Therefore, the corn planting and harvest should be planned to secure sufficient GDD for corn with little impact anticipated on the following cool-season annual forage crops. The mean STP of late-maturity corn was around 15°C which is lower than the other two maturity group mean values by one degree (Table 1). Therefore, it seems that the late-maturity corn was planted earlier to secure enough GDD before cool-season annual planting. However, the mean corn biomass of the late maturity group was not substantially more than that of early or medium-maturity corn, indicating insufficient GDD before harvest when trials were designed with fall crop planting.
Meanwhile, the biomass of early-maturity corn was equivalent to that of late maturity corn, which indicates the GDD requirement was more likely fulfilled in the current study for early maturity corn but not for late maturity corn. However, fewer trial data for the early maturity group than for the other maturity groups warrants more valid trials in the future. Also, the lower number of early-maturity corn trials probably reflects the greater cultivation priority for late-maturity corn in the Rural Development Administration of South Korea because of the relatively greater biomass accumulation potential than early-maturity corn. However, as presented in this study, less sensitive biomass reductions from the less-than-optimal STP and harvest management and more complete fulfillment of GDD requirements, early-maturity corn has a particular value in the corn–cereal forage cropping system. Bello et al. [32] reported that early-maturity corn cultivation is more advantageous because of more production stability in Nigeria, with relatively low risks of yield loss with poorly timed harvest caused by the erratic rainfall pattern. Because harvest and ensiling of silage corn should be done early enough to provide an adequate growing period for fall planted cool-season forage crops to ensure winter survival, early maturity corn would be more suitable in the double cropping system than late maturity corn.
Although corn grain harvest is recommended at the black layer stage of ear development [33,34], silage corn harvest should not pass the stage, considering silage intake and digestibility [17]. Moreover, the degree of biomass or feed value reductions typically differs between pre- and post-biomass peaks. The scarcity of feed value data limited the estimation of such aspects with the acquired field trial data. Therefore, this silage corn biomass modeling could not include the feed value aspects at harvest. However, the accumulation of ear biomass provides more energy accumulation since the ear is the primary storage of digestible nutrients of corn [14]. Therefore, ear proportion should be maximized in the whole crop biomass. The theoretically greater biomass accumulation of late-maturity corn cultivars was not achieved in the past 40 years of the Korean field trial data. Considering the erratic weather patterns, available forage production resources, and cropping system, South Korean forage production should reassess the value of silage corn maturity as presented by the silage corn biomass models. Since silage corn is more productive than cool-season annual forage crops in terms of forage biomass and energy production potential, a double cropping system should be designed to secure the full growth of corn cultivars to maximize the biomass potential.