INTRODUCTION
Chicken meat is a staple food worldwide because of its nutritional value, easy availability, high growth rate, and relatively low price. Its consumption has constantly been increasing and is expected to grow at the fastest rate in terms of total meat output [1]. However, chicken meat is easily degraded by enzymatic and chemical reactions and bacterial contaminants because of its high final pH, large amounts of nutrients, and high water activity, which limit its shelf life [2]. Therefore, extending its shelf life has long interested the food science and industry [3].
Freezing is one of the most widely used preservation methods for meat and meat products because it can store meat for a long time with relatively less quality loss [4]. Low temperatures during frozen storage inhibit the proliferation of microorganisms and undesirable biochemical reactions, such as the oxidation of proteins and lipids [4,5]. Nevertheless, there is an inevitable and irreversible loss of physicochemical properties and sensory quality after freezing and thawing. As a result, consumers perceive frozen meat as having low quality and are willing to pay less for it than for fresh, non–frozen meat [6]. The formation of ice crystals during freezing is known to cause inferior textural properties and juiciness and changes the nutritional and flavor compounds of meat, which influences the sensory acceptability of consumers [5,7]. Changes in textural properties and juiciness are caused by ice crystallization-induced physical damage to muscle tissues and subsequent deformation of the meat structure [7].
With regard to changes in the flavor compounds of frozen meat, only a limited number of studies have been conducted. Soyer et al. [4] suggested a possible change in meat flavor by freeze-thawing by evaluating the changes in proteins and lipids according to the frozen temperature and storage duration in chicken meat and found increased sulfhydryl content and lipid oxidation products (peroxides and malondialdehyde) by protein and lipid oxidation, implying possible changes in meat flavor. Al-Dalali et al. [8] studied the differences in volatile flavor compounds between fresh and frozen–thawed beef and suggested six major compounds (1-heptanol, 2-ethyl-1-hexanol, benzeneacetaldehyde, hexanal, isoeugenol, and octanal) as indicative markers of freezing. Regarding the aromatic characteristics of chicken meat, Qi et al. [9] revealed differences in the flavor compounds of broths prepared from fresh or frozen–thawed chicken and flavor compounds of chicken meat after making the broth by the duration of the frozen storage [10].
However, research on the flavor compounds in fresh and frozen chicken meat is limited, even though flavor is a critical sensory attribute of meat. In particular, aroma influences the decision making of the consumers by being detected directly by the nose before consumption as well as during eating [11]. In this study, it was postulated that the flavor compounds of fresh and frozen chicken meat would be composed differently. Therefore, this study aimed to identify the aroma- and taste-related compounds in fresh and frozen–thawed chicken breast meat.
MATERIALS AND METHODS
Fresh broiler meat was obtained from a slaughterhouse in Chuncheon, Korea (n=20, 1.1 ± 0.1 kg). The meat was first kept at 4°C in a laboratory; chicken breast meat was deboned from the carcasses and half of them were directly analyzed as a fresh sample. The other half of the carcasses was directly frozen in a freezer at −18°C and kept for 2 months. The frozen–thawed sample was prepared by thawing the frozen chicken carcass in a refrigerator (4°C, 16 h). And its breast meat was deboned and subsequently utilized for analysis.
The method described by Kim et al. [12] was utilized to determine the content of nucleotide-related compounds. Minced chicken breast meat sample (5 g) were taken and homogenized with 0.7 M perchloric acid (25 mL). The homogenate was centrifuged for 15 minutes at 0°C at a force of 2,000×g then filtered through Whatman filter paper (No. 4). The remaining pellet was extracted again with 0.7 M perchloric acid (20 mL) and filtered. The pH of the collected filtrate was regulated to 6.5 using 5 N KOH solution. Then the filtrate was moved to a volumetric flask and was diluted to a final volume of 100 mL with 0.7 M perchloric acid. After being cooled for 30 minutes, the solution was centrifuged (1,000×g, 10 min, 0°C), and the supernatant was filtered using a 0.22-μm syringe filter. The filtered supernatant was analyzed by high-performance liquid chromatography (HPLC; Agilent 1260 Infinity, Agilent technologies, Santa Clara, CA, USA). The HPLC analysis condition included a Nova-pak C18 column (150 × 3.9 mm, 4 μm particles; Waters, Milford MA, USA) eluting 1% trimethylamine · phosphoric acid (pH 6.5) at a 1.0 mL/min flow rate. Standards of 5′-adenosine monophosphate (AMP), 5′-inosine monophosphate (IMP), inosine, 5′-adenosine triphosphate (ATP), 5′-adenosine diphosphate (ADP), and hypoxanthine (Sigma Aldrich, St. Louis, MO, USA) was utilized on analysis.
The method described at Ali et al. [13] was utilized to determine free amino-acid composition of the sample, with slight modifications. A homogenized chicken breast meat (2 g) with 2% TCA solution (27 mL) was centrifuged at 17,000×g for 15 min. The supernatant was filtered through a syringe filter (0.45 μm). The filtrate was subjected to be analyzed on an amino-acid analyzer (S433 A.A., Sykam GmbH, Eresing, Germany): a column size of 4.6 mm i.d. × 150 mm, lithium form resin, lithium citrate buffer (pH 2.9, 4.2, and 8.0), flow rates of 0.45 mL/min, and 0.25 mL/min for ninhydrin. The column temperature was 37°C, the reaction temperature was 110°C, and the analysis time was 120 min. The amount of amino acids analyzed by comparing the absorption intensities of the samples to those of a standard stock solution with a known amino acid content (type PH, Sykam GmbH).
The methods described by Kim et al. [14] was used to analyze the fatty-acid composition. The lipids were extracted from a 2 g sample of chicken meat using 15 mL of Folch solution (2:1 mixture of chloroform and methyl alcohol, v/v). To prevent oxidation, 40 μL of butylated hydroxy anisole solution was added to the homogenates prior to extraction. After homogenization, the homogenate was filtered using Whatman No. 1 filter paper. After adding 4 mL of KCl (0.88%), the filtrate was vortexed and centrifuged (10 min, 783×g). The separated lower layer of the filtrate, which contained lipid, was condensed using nitrogen gas. A 25 mg lipid was taken in a glass tube and mixed with 1.5 mL of 0.5 N NaOH (in methyl alcohol). After heating the tube at 100°C for 5 min, it was added with 1 mL of 10% BF3 and heated again at 100°C for 2 min. After adding 2 mL of isooctane and 1 mL of saturated NaCl in the tube, it underwent centrifugation at 783 ×g for 3 min. The iso-octane extract aliquot was utilized on gas chromatograph analysis (GC; Agilent 7890N, Agilent Technologies, Santa Clara, CA, USA). The GC was equipped with an Omegawax 250 capillary column (30 m × 0.25 mm × 0.25 mm, Supelco, Bellefonte, PA, USA). On identification of each fatty acid, a mixture of fatty acid standards (PUFA No.2; Animal Source, Supelco) was used to compare their retention time with that of samples.
The profile of VOCs was determined gas chromatography–mass spectrometry (GC–MS) analysis using headspace solid-phase micro-extraction (HS-SPME) method as described by Lv et al. [15]. The homogenized chicken meat samples (5 g) were placed in a glass vial (20 mL) and capped, then were incubated at 60°C for 25 min in a water bath. The absorption of the volatiles was done by exposing a DVB/ CAR/PDMS fiber (50/30 μm, Merck, Darmstadt, Germany) to the vial headspace for 30 min in the same condition of water bath. The lengths of the fibers in the headspace were constantly kept. Prior to each analysis, the fibers were exposed for 30 min in the inlet of the GC-MS to eliminate volatile contaminants.
The analysis of volatiles was implemented using a GC (Agilent 8890, Agilent Technologies) coupled to a MS (Agilent 5977 B, Agilent Technologies). To identify the volatile compounds, two methods were used. Firstly, linear retention indices (LRI) were compared to standard compounds and literature data for homologous series of n-alkanes (C8–C24, Polyscience, Niles, IL, USA). Secondly, MS data was compared to reference compounds and MS data obtained from the NIST 20 library (NIST/EPA/NIH Mass Spectral Library with Search Program) to deconvolute mass spectra and identify target components. The data was reported as the abundance of the characteristic anions for each component (area × 106). The flavor characteristics of the VOC were obtained from the following online databases: Flavornet (http://www.flavornet.org/), FlavorDB (https://cosylab.iiitd.edu.in/flavordb/), and FooDB (https://foodb.ca/).
The sensory analysis of chicken breast meat was evaluated by 15 panelists between the ages 21 and 38. The vacuum-packed chicken breast meat using polyethylene bag was cooked in a 75°C water bath for 45 min until its internal temperature reached 73 ± 2°C. Subsequently, 1×1×2 cm size pieces were served. Between treatments, the panelists were asked to rinse their palates with water to minimize the influence of the flavor of the previous sample on the evaluation of the next sample. According to a 9-point hedonic scale, color, aroma, taste, flavor, and texture (1 = very bad, 9 = very good), juiciness (1 = very dry, 9 = very juicy), and tenderness (1 = very hard, 9 = very tender) of the fresh and frozen–thawed breast meat of broiler was evaluated. In advance of the sensory analysis, it was approved by the Institutional Review Board of Kangwon National University (KWNUIRB-2021-05-004-001).
Mean values and standard deviations are presented based on five replicates of the analyses. Statistical analysis was performed using one-way analysis of variance and Tukey’s test to identify the significant differences between treatments (p < 0.05). SAS software v.9.4 (SAS Institute, Cary, NC, USA) was utilized. The different superscripts indicated significant differences between fresh and frozen–thawed meat. Principal component analysis, hierarchical clustering analysis, and heat map analysis were performed using Metaboanalyst 3.0 online analysis software.
RESULTS AND DISCUSSION
Among the analyzed nucleotide-related compounds, AMP, IMP, inosine, and hypoxanthine are taste-active compounds. AMP contributes to the sweet taste of meat at concentrations of 50–100 mg/100 mL [16]. The results showed that its content was below the required level to produce a sweet taste in both fresh and frozen–thawed meat (Table 1, 6.61 mg/100 g and 8.54 mg/100 g, respectively). When its concentration is below 50 mg/100 mL, AMP can synergistically increase the umami taste with IMP rather than being sensed as its own sweet taste [17]. Hence, the AMP contents in this study implied that it would have synergistically elevated the umami taste of chicken meat along with IMP. In particular, frozen–thawed meat had a significantly higher AMP content than fresh meat, which increased the umami taste of frozen–thawed chicken meat more than that of fresh meat. IMP has an intense umami taste that is much stronger than that of monosodium glutamate (MSG) [17]. Because it positively affects meat flavor, IMP is considered an important factor in the chicken meat flavor [18]. We found that IMP was the major nucleotide-related compound, followed by inosine, hypoxanthine, and ATP, in both fresh and frozen–thawed broiler breast meat (Table 1). The IMP content in fresh meat was 62.5% higher than those in frozen–thawed meat (p < 0.05). It was reported that the higher the IMP content in meat, the more positively it affects the flavor of the meat [19]. Therefore, the higher IMP content of fresh meat compared to frozen–thawed meat would have contributed to the better flavor of fresh meat compared to frozen–thawed meat. The degradation products of IMP, inosine, and hypoxanthine are known to produce a bitter taste [20]. In this study, the inosine content was higher (p < 0.05) in frozen–thawed meat. Hypoxanthine content tended to be higher in frozen–thawed meat but was not significantly different from that in fresh meat. The increased inosine and hypoxanthine contents could have negatively impacted the taste of frozen–thawed meat.
These differences in nucleotide-related compounds between fresh and frozen–thawed chicken meat may be due to the damage of muscle cells during freezing and thawing. An increased drip loss after thawing meat can result in meat with less acceptability due to the loss of taste compounds, such as amino acids or nucleotides [21]. Like the results of this study, a previous study comparing the flavor compounds of fresh and frozen chicken meat utilized for stewing [10] reported that frozen chicken meat had lower IMP and guanosine monophosphate (GMP) content than fresh chicken meat. However, the increased inosine and hypoxanthine contents after freezing and thawing may have occurred through another pathway. Inosine and hypoxanthine can be produced from IMP by 5’-nucleotidase and nucleoside phosphorylase, sequentially [16]. Thus, the increased inosine and hypoxanthine levels suggest the contribution of nucleotide-metabolizing enzymes to the changes in nucleotides after freezing and thawing meat [9].
Free amino acids contribute to the bitter, sweet, or umami taste of meat [16]. Sulfur-containing amino acids (cysteine and methionine) contain sulfur notes. Arginine, histidine, isoleucine, leucine, lysine, methionine, phenylalanine, threonine, tryptophan, tyrosine, and valine have bitter taste [17,22]. Threonine, serine, glycine, and alanine can be classified as sweet amino acids. Sweet amino acids synergistically interact with IMP and exhibit increased sweetness in the presence of IMP [23]. In the presence of sodium salts, glutamic acid and aspartic acid are known to have umami taste. Taurine, asparagine, and glutamine have miscellaneous tastes or are tasteless [17,24].
The compositions of free amino acids in fresh and frozen–thawed chicken breast meat are shown in Table 2. Freezing and thawing increased the total amount of free amino acids. The contents of most amino acids increased significantly, except for tryptophan. With an increase in each free amino acid, the contents of bitter-, sweet-, and umami-related free amino acids also increased. Considering their effect on the taste of fresh and frozen–thawed chicken meat, discussing the effect of their tastes on the overall flavor of each meat was difficult because the contents of sweet, bitter, and umami amino acids were simultaneously increased by approximately 3 to 4 folds. Nevertheless, the increased content of total free amino acids in frozen–thawed chicken meat compared to fresh meat may have increased the flavor intensity of the meat [25].
In a previous study on differences in the taste-related compounds upon stewing fresh and frozen stored bone-in chicken meat [10], increases in some free amino acid content after freezing and thawing was reported, similarly to the result herein. However, differences were observed in the individual amino acids. In their study, the glutamic acid, cysteine, valine, methionine, isoleucine, leucine, tyrosine, phenylalanine, and lysine contents increased, while those of threonine and arginine decreased. Aspartic acid, glycine, alanine, and proline levels were similar before and after freezing and thawing. Qi et al. [10] referred to the cause of the increased free amino acids in frozen–thawed meat as a promoted migration of free amino acids into the meat from the bone with a high amino acid content, which was caused by freeze–thawing. As described above, the frozen-thawed chicken breast meat in the present study was frozen with bones and deboned after thawing. Therefore, the migration of free amino acids could also explain the increase in free amino acids in frozen–thawed meat. In another study [9], the possibility of changes in taste compounds caused by endogenous meat enzymes after thawing frozen meat was speculated. During the thawing of meat, ice crystal-induced damage in muscle cells allows the release of enzymes from lysosomes and the mitochondria into the sarcoplasm. Through reactions with solutes in the sarcoplasm, the enzymes would increase the content of taste compounds in meat [9]. Coombs et al. [26] also reported that the rate of proteolytic enzyme hydrolysis increased after freezing meat. In contrast to previous reports by Qi et al. [10], the threonine, serine, glycine, alanine, arginine, and proline contents increased after freeze–thawing in this study. These differences depend on whether the sample is cooked, as free amino acids undergo complex reactions with other food components during cooking [19].
Among the fatty acids, oleic acid, which is highly correlated with the flavor of meat [18], composed the highest proportion in both fresh and frozen–thawed chicken meat (Table 3). It was followed by palmitic, linoleic, and stearic acids. This trend is similar to that previously reported for the fatty acid composition of broiler chicken breast meat [18,27]. In this study, the oleic acid composition was not affected by freezing or thawing. In addition, palmitic acid and linoleic acid contents were stable after freezing and thawing.
A decrease in the fatty-acid composition after freeze–thawing was mostly observed for arachidonic acid, adrenic acid, eicosapentaenoic acid (EPA) and docosahexaenoic acid (DHA), which are unsaturated fatty acids (UFAs). The same trend in which unsaturated fatty acids decreased after freeze–thawing was previously reported by Igene et al. [28], regardless of the cuts of chicken meat and beef. The loss of unsaturated fatty acids could be caused by the instability of UFAs upon lipid oxidation compared to that of saturated fatty acids [28,29]. Arachidonic acid is known to contributes to the umami flavor in chicken meat [19,30]. EPA and DHA are indispensable for the optimal functioning of cells, tissues, organs, the brain, and the immune system, imparting early development to the brain and eyes and as precursors of anti-inflammatory eicosanoids, respectively [31]. Therefore, a decrease in these fatty acids in freeze–thawed chicken meat negatively affects the flavor and nutritional aspects of the meat. In addition, the content of stearic acid, which was saturated, decreased. A decrease in stearic acid content could affect the taste of frozen–thawed meat because it is reportedly related to fatty taste [32].
Freezing and thawing induced increases myristic and α-linolenic acid contents. Regarding the increase in some fatty acids after freezing and thawing, some literatures addressed that the contents of some saturated fatty acids and UFAs were higher in frozen–thawed beef than in fresh beef. He et al. [33] reported increases in saturated fatty acid (SFA) and monounsaturated fatty acid (MUFA) contents and Al-Dalali et al. [8] presented increase in palmitic, palmitoleic, stearic, oleic and eicosanoic acids composition. Those increase may be considered as the result of proportional increase after decrease of some fatty acids. However, it cannot explain the increase of each fatty acids, therefore, the mechanisms underlying these increases in fatty acid levels have not yet been fully elucidated. The known cause for changes in fatty-acid composition is mainly about the effect of the feed offered to chickens and individual variation between chickens [34]. Hence, further research is required to clearly understand the differences in the increase in some fatty acids after freezing and thawing meat.
In fresh and frozen–thawed broiler breast meat, 95 VOCs were detected and classified as acids, alcohols, aldehydes, esters, hydrocarbons, and ketones (Table 4). The total amount of VOCs was higher in frozen–thawed chicken meat than in fresh meat, with significantly higher amounts of aldehydes and ketones. Increases in the aldehyde, ketone, and alcohol classes of VOCs have been reported in stewed chicken meat that had undergone freezing and thawing; the authors also referred to their cause as lipid oxidation [9]. In addition, flavor precursors (e.g. peptides, amino acids, organic acids, sugars, adenine, and nucleotide breakdown products) are produced during frozen storage and postmortem. These precursors are known to be degraded during frozen storage by diverse chemical reactions, such as proteolysis, lipolysis, and oxidation, forming diverse flavor compounds [8].
The increase in alcohols could imply the deterioration of protein-based foods because they can be produced through the microbial metabolism of proteins and amino acids [35]. Alcohols are also produced by fat degradation [35]. Among the alcohols, 1-octen-3-ol showed the largest, approximately 11-fold, increase after freezing and thawing. Owing to its raw, fishy, oily, earthy, and fungal aroma, 1-octen-3-ol negatively affects the aroma of freeze–thawed chicken meat. Alcohols are also considered to be responsible for the warmed-over flavor of meat and can be found in the internal parts of boiled pork meat [36]. After freeze–thawing, (S)-(+)-3-methyl-1-pentanol (cocoa, cognac, fruity, fusel, and green aroma) and 1-dodecanol (coconut, earthy, honey, wax, fat, and soapy aroma) increased (p < 0.05). In particular, 1-nonanol (fatty, dusty, floral, rose, clean, bitter, wet, orange, and oily aroma) and 2-octen-1-ol, (E)- (coconut, orris, fruity, and waxy aroma) were newly produced after freezing and thawing; therefore, they may be utilized as markers for differentiating between fresh and frozen–thawed chicken meat.
Among the aldehydes, most volatile compound levels were increased by freeze–thawing, including nonanal (citrus, rose, green, waxy, fishy, fresh, aldehyde, orris, and grapefruit aromas, p < 0.05) and octanal (lemon, citrus, fat, soap, waxy, fatty, aldehyde, and green aromas, p < 0.05). In a previous research, these compounds were reported to be the products of lipid oxidation in beef, along with pentanal, hexanal, and heptanal [37]. Moreover, 2-nonenal, (E)- (aldehyde, citrus, fat, cucumber, green, and paper aromas), 2-octenal, (E)- (green, nut, and fat aromas), and 5-ethylcyclopent-1-enecarboxaldehyde (aroma data not available; NA) were distinctive compounds found only in freeze–thawed meat but not in fresh meat. Although the aroma profile of 5-ethylcyclopent-1-enecarboxaldehyde is unknown yet, it could be considered important with analytical implications to identify frozen-thawed meat.
The number of esters was the only that decreased after freezing and thawing. Arsenous acid, tris(trimethylsilyl) ester (NA), and propanoic acid, 2-methyl-, 3-hydroxy-2,2,4-trimethylpentyl ester (NA) levels significantly decreased. Ethyl 9-hexadecenoate (NA), ethyl oleate (fatty type odor), hexadecanoic acid, and ethyl ester (mild waxy, creamy, fruity, milky, and balsamic aromas), which are present in fresh chicken meat, could not be detected after freeze–thawing; therefore, they might be potential markers for fresh chicken.
Hydrocarbons represented the largest portion of VOCs in both frozen and frozen–thawed chicken meat. Among hydrocarbons, the contents of hexathiane (NA), methane, dichloronitro- (NA), oxetane, 3-(1-methylethyl)- (NA), and pentadecane (alkane aroma) were significantly increased, and nonane, 2-methyl- (NA), and oxetane, 3,3-dimethyl- (NA) were newly produced after freezing and thawing. In addition, indole (burnt, animal, naphthalene, fishy, jasmine, floral, honey, and fecal aromas) and nonane, 2,5-dimethyl- (NA) disappeared after freezing and thawing. Ketones exhibited an 8-fold increase after freezing and thawing compared to fresh meat (p < 0.05). 2-Butanone (acetone, camphor, ether, and fruity aromas) was newly generated after freezing and thawing and was the most abundant ketone in frozen–thawed chicken breast meat. 5,9-Undecadien-2-one, 6,10-dimethyl-, and (E)- (NA) levels decreased after freezing and thawing (p < 0.05). In ketone and acid classes, VOCs, which are formed mainly as a result of lipid oxidation in meat, also increase after freeze–thawing: furan, 2-pentyl- (green bean, and butter aromas) in ketones and hexanoic acid (cheese, fatty, sour, and sweat aromas [37]). Among the unclassified VOCs, a large amount of sec-butylamine, which has an ammonia and fishy aroma, was generated after freezing and thawing, with a significantly higher value than that in fresh meat.
Taste compounds (nucleotide-related compounds, free amino acids, and fatty acids) and aroma compounds (VOCs) were analyzed using a heatmap and multivariate analysis (PLS-DA) for estimation of the impacts of the different compounds on fresh and frozen–thawed chicken meat. Hierarchical clustering heatmap analysis revealed clear clusters of fresh and frozen–thawed chicken meat for both taste and aroma compounds with different compositions of each compound (Fig. 1). The PLS-DA results for taste compounds and VOCs also clearly separated the 95% confidence region between fresh and freeze–thawed chicken meat (Fig. 2). The variable importance in the projection (VIP) score quantifies the significance of each variable in the PLS-DA model based on the variance observed between two distinct groups. A higher VIP value indicates a larger disparity in the content of a given variable among different groups and the more importance in the classification [38]. The VIP score showed major effects of amino acids and IMP on the discrimination of fresh and frozen–thawed chicken meat. The total amounts of free amino acids, sweet amino acids, bitter amino acids, IMP, alanine, umami amino acids, serine, glycine, glutamic acid, and leucine contributed significantly to the separation in PLS-DA, with a high VIP score (> 1.2, in order of higher values). With respect to aroma compounds, VOCs such as ketones, oxetane, 3,3-dimethyl-2-butanone, arsenous acid, tris(trimethylsilyl) ester, indole, ethyl oleate, and 1-dodecanol had high VIP scores (>1.2, in order of higher values). When the VIP score of a component is above 1.2, it can be considered a potential marker to distinguish a certain effect [39]. Therefore, the contents of these compounds could be utilized as distinguishing markers for fresh or frozen–thawed chicken breast meat. In particular, VOCs that can be detected exclusively in fresh chicken breast meat such as indole, ethyl oleate, and hexadecanoic acid and only in frozen–thawed meat such as oxetane, 3,3-dimethyl-, 2-butanone, and 1-nonanol are worth referring to as prospective key markers.
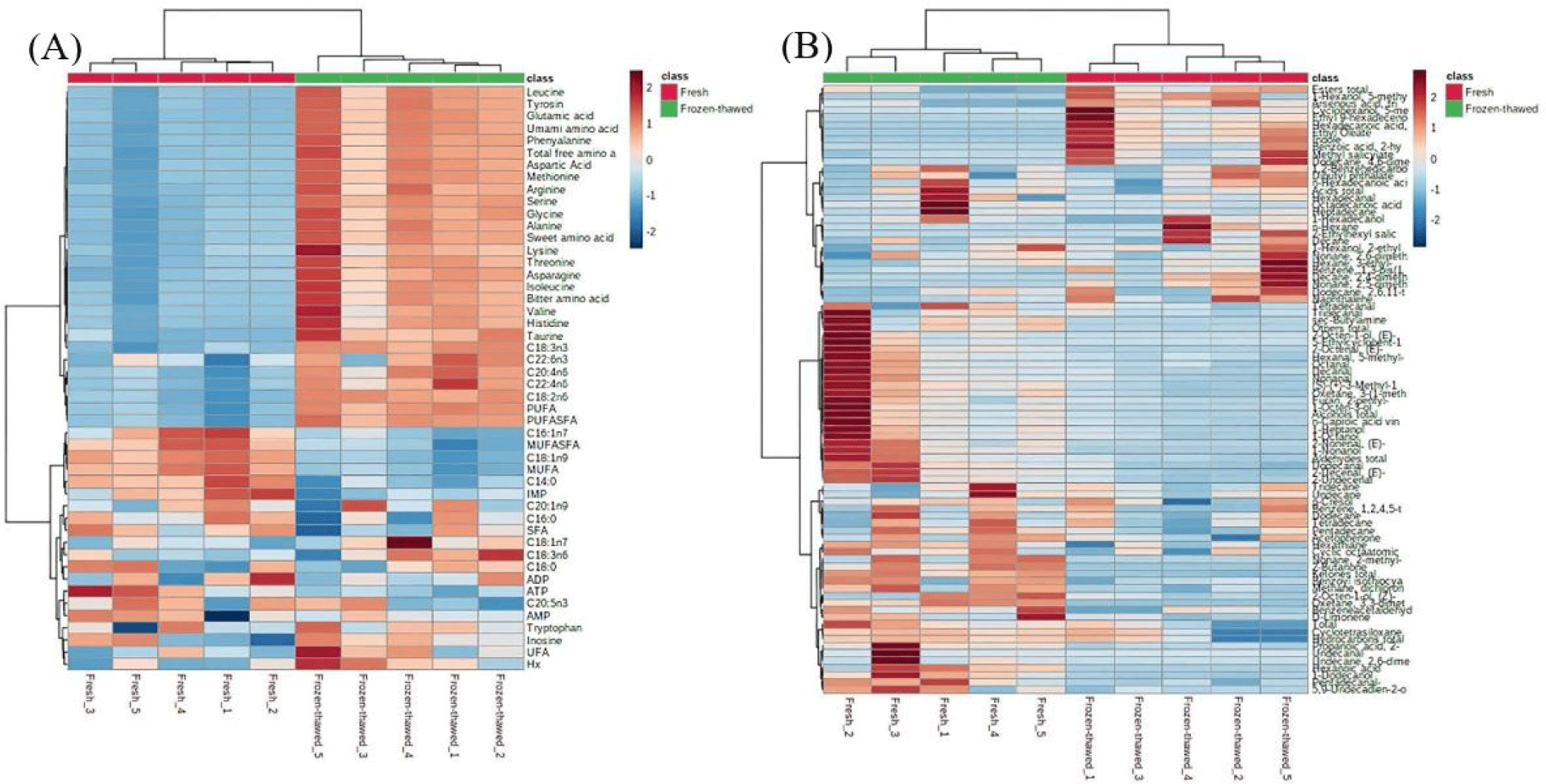
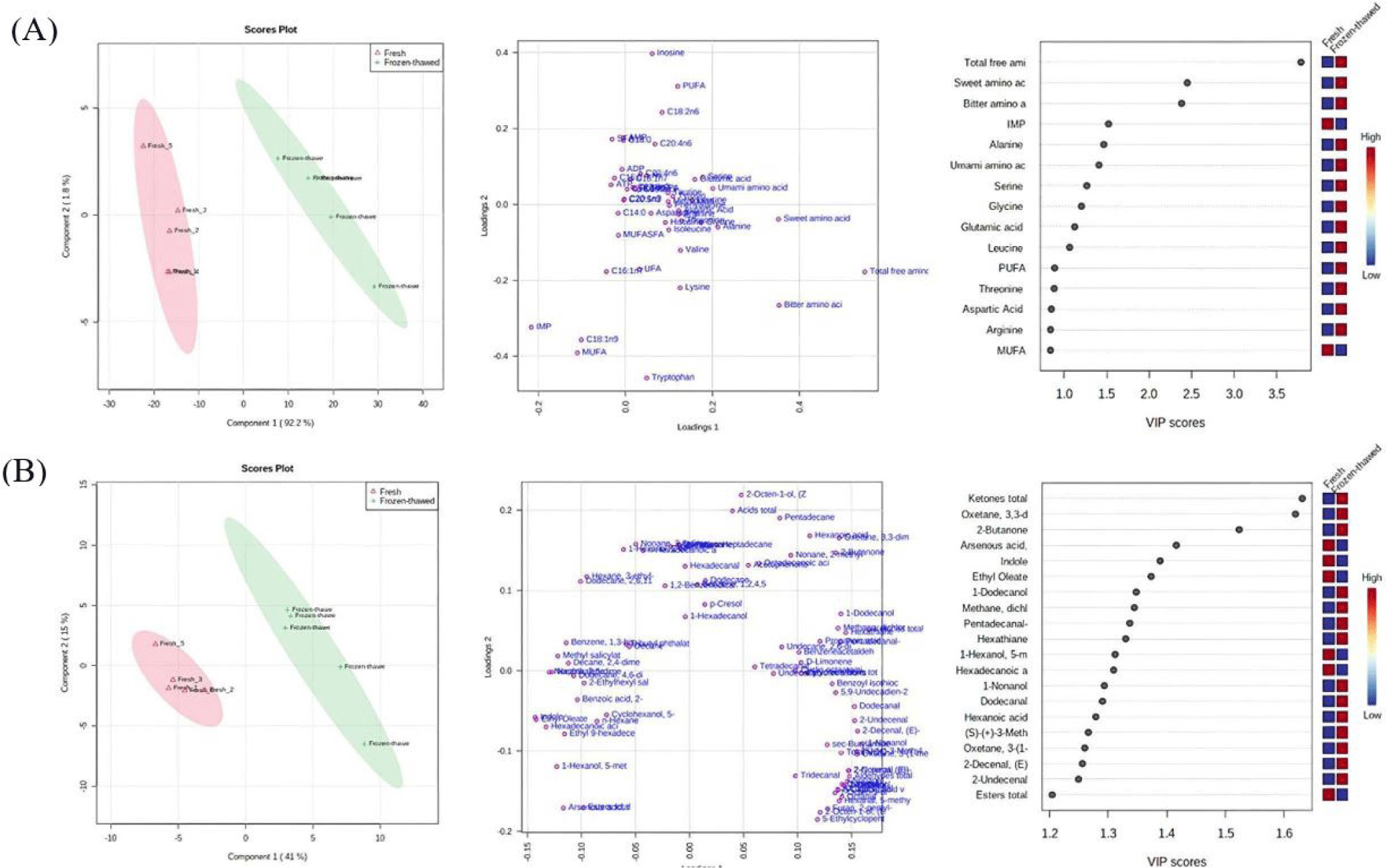
Although numerous differences in taste and aroma compounds were observed between fresh and freeze–thawed meats were in this study, only slight differences were observed in sensory evaluation of this study (Table 5). The color, aroma, taste, flavor, and overall acceptability scores of the frozen–thawed samples were not significantly different from those of the fresh sample but tended to be lower than those of the fresh sample. Although the difference in the aroma, taste, and flavor scores was not significant in this study, it is well known that the freezing and thawing generally deteriorate food flavor [40]. Therefore, further studies are required to determine the effect of specific changes in taste and aroma compounds after freezing and thawing on the sensory properties of chicken meat.
CONCLUSION
Freezing and thawing of chicken breast meat significantly affected the profiles of taste-related compounds and VOCs. Analysis of nucleotide-related taste compounds showed that IMP levels decreased, and inosine levels increased. The content of free amino acids, including bitter, sweet, and umami amino acids, increased significantly. Some changes in polyunsaturated fatty acid (PUFA) were observed. Although the difference in sensorial flavor between fresh and frozen–thawed chicken meat was insignificant, the analyzed flavor compounds displayed divers differences and the discriminated PLS-DA results for both taste and aroma compounds support this. The detected compounds with high VIP scores, including free amino acids, sweet amino acids, bitter amino acids, IMP, ketones, oxetane, 3,3-dimethyl-, and 2-butanone, could be used as markers to differentiate between fresh and frozen–thawed chicken meat. In addition, the increased or newly generated compounds in frozen–thawed chicken meat, such as oxetane, 2-butanone and oxetane, 3,3-dimethyl- could be utilized to offset the defects in frozen chicken meat flavor.