INTRODUCTION
At least 20% of chicken fresh-cut carcasses are transformed into mechanically deboned chicken meat (MDCM) [1]. Mechanically deboned chicken meat is high-calorie food material as 12 kJ/g and contains plenty of unsaturated fatty acids including phospholipids [2]. However, as it contains approximately 12% crude fat, MDCM has low preservability due to rancidity [2,3] Therefore, most of MDCM has been used as substitutes in processed meats, such as meat emulsion, paste meat and chicken nuggets [1].
The companion animal food industry relies heavily on by-products of meat processing industries as sources of protein [4]. Therefore, the less-utilized MDCM could be reused as a value-added protein resource in the companion animal food industry through suitable procedures. The drawback of MDCM with respect to low preservation could be fully overcome by well-established fermentation technology. It is well known that the food fermentation has several advantages as follows: it preserves the raw materials at low cost; enhances the nutritional quality by improving digestibility and protein quality; destroys toxic and undesirable components and harmful biota, protects against re-infection and adds antibacterial components; and improves the appearance, flavor and texture of foods [5,6]. In addition, allergic reactions induced by the increased use of proteins could be resolved by suitable fermentation [7]. With this background, the aim of this study was to evaluate the MDCM-based fermented snack for dogs.
MATERIALS AND METHODS
Chicken breast meat (CBM) and MDCM as main protein ingredients were obtained from a local chicken processing plant (Singgreen Food System, Iksan, Korea). Upon the receipt of CBM and MDCM, they were stored at −20°C until use. The proximate analysis revealed the MDCM had 63.1% moisture, 53.2% CP, 18.9% EE and 2.7% ash on a DM basis. The CBM was composed of 73.5% moisture, 87.8% CP, 4.6% EE and 5.4% ash on a DM basis.
Pediococcus acidilactici (ATCC 8042) and P. pentosaceus (ATCC 8081) were obtained from the Korean Culture Centre of Microorganisms (KCCM, Seoul, Korea). Strains were enriched on MRS Agar (BD Difco, NJ, USA) at 37°C for 24 h. Colonies were suspended with sterilized distilled water and used as a starter culture.
The CBM and MDCM based meats were prepared for snack production. In brief, MDCM and CBM were thawed in refrigerator at 4°C, cut by 3 × 3 × 3 cm in size, and chopped with a meat grinder (M-12S, Hankook Fujee, Hwaseong, Korea) into small particles of 2.5 mm in diameter. The chopped raw meats were then mixed with sodium chloride, nitrate, nitrite, phosphate, cornstarch, and glucose. The mixtures were then divided into six equal batches of 1 kg each and wrapped with foils. Three of the batches were sterilized immediately at 121°C for 20 min, kept in −20°C until use and served as a non-fermented snack control. The other three batches were inoculated with the prepared starter culture to reach 107 CFU/g meat mixtures and incubated in incubator (HB-101L, Hanbaek, Bucheon, Korea) at 37°C for 24 h. The fermented batches were sterilized as described in the non-fermented control and kept in −20°C until use. The ingredients and chemical compositions of chicken meat-based snacks were presented in Table 1.
During a 24-hr fermentation, small portions (n = 3) were sampled at 0, 3, 6, 9, 12, 18, and 24 h after the initiation of the incubation to measure the pH and the enumeration of lactic acid bacteria (LAB). In addition, the fermented and non-fermented snacks were analyzed for lactic acid, ammonia, moisture, crude protein, crude fat, crude ash, minerals, cholesterol, gross energy, amino acids, fatty acids, and in vitro pepsin digestibility.
To test preservability of the fermented or non-fermented snack food, approximately 200 g of the wet samples were weighed into 10 cm petri dishes and kept them with lid open on a laboratory at room temperature. Samples were taken at 0, 1, 2, 3, 5, 7, and 14 d after exposure to the environment, and analyzed for the total bacteria, pathogenic bacteria, and LAB.
Seven 2-year-old male adult Maltese with average body weight of 3.4 kg (SD = 0.35) were used for palatability trial. The dogs were randomly allocated to individual stainless cages of 75 × 50 × 60 cm. A commercial feed (Natural Balance, Suwon, Korea) was used as the basal feed which contained 10% moisture, 25.6% CP, and 14.4% EE on a DM basis. All dogs were initially fed to satiety with the basal diet and subsequently provided each dog with 50 g (approximately 1.5% of the individual body weight) of the fermented and non-fermented snacks. Palatability parameters monitored included smelling and eating behaviors, first choice, and eating time during 10 min after snack presentation. During 10 min, smelling and eating behaviors on respective snacks were counted, and first choice was calculated as the percentage of dogs eaten the first snack sample. After 10 min of the test, the leftovers were measured to calculate food intake. Snacks were located in the same distance from each dog. The palatability test was repeated three times and averaged.
Dogs that had been used for the palatability trial were initially provided with the commercial feed (Natural Balance, Suwon, Korea) every morning at the same time and the allowance was calculated based on the maintenance energy requirement [8]. Fermented and non-fermented snack foods were prepared as described earlier. The basal feed was gradually replaced with either fermented or non-fermented snack over the course of 7 days. After 7-d adaptation period, four Maltese dogs were provided with the non-fermented snack while three Maltese with fermented one during subsequent 12 days. Snacks (as-is basis) equivalent to 10% of the individual body weight were supplied ad libitum. The fecal and urine samples were collected during the last 5 days of the experimental period and pooled per dog. Urine samples were recorded per dog. All dogs were individually weighed at the initiation and the termination of the trial. All dogs had ad libitum access to drinking water using companion animal waterers. This experiment repeated twice with one-month interval. All experiment protocols were approved by the Animal Care Committee of KULF Corp. (KULF-19-1).
A 5-g portion of the snack and fecal samples was homogenized with twice (w/w) the amount of distilled water, and measured by a pH meter (HI 120, Hanna instruments, RI, USA).
The proximate composition of MDCM, CBM, non-fermented and fermented snacks, and fecal samples were analyzed by the methods of AOAC [9]. Gross energy was measured using Bomb calorimeter (C200H, IKA, Staufen, Germany). Cholesterol was quantified as described by An et al. [10] using IATRO SCAN (MK5, Iatron Laboratories, Tokyo, Japan) after extraction of the total lipid according to the methods of Folch et al. [11]. Lactic acid and ammonia were pre-treated by lactic acid kit (Megazyme, Wicklow, Ireland) and ammonia kit (Megazyme, Wicklow, Ireland), respectively, and then the concentrations were read at 340 nm by spectrophotometer (Synergy2, BioTek, VT, USA). To determine the fatty acid composition of the snack samples, total lipids were extracted according to the Folch et al. [11]. Then, the fat was saponified with 0.5 N methanolic sodium hydroxide and methylated with boronitrifloride-methanol complex according to the method of Metcalfe et al. [12]. The methylesters were separated and quantified by gas chromatography (HP5890, Agilent, CA, USA). Amino acid contents in the snack samples were quantified by amino acid analyzer (S433, Sykam, Eresing, Germany), following hydrolysis in 6 N HCl for 24 h at 100°C as described elsewhere [9]. All the analysis was triplicated.
Pepsin digestible nitrogen in the snack samples was determined according to the procedure of the AOAC [9] using 0.2% pepsin solution. The samples were defatted before analysis. The analysis was triplicated.
Total bacteria, LAB, and pathogenic bacteria were measured using LB agar media (BD Difco, NJ, USA), MRS agar media (BD Difco, NJ, USA), and Orientation agar media (CHROMagar, Paris, France). Ready-to-use orientation agar medium is a non-selective medium for the isolation, direct identification, differentiation and enumeration of pathogens including Escherichia coli due to pathogen-specific colony color. Plates were incubated for 24 h at 37°C, and colonies were counted and expressed as log10 transformed colony forming unit (CFU).
All data, if not stated otherwise, were analyzed by general linear model (GLM) of a SAS (SAS Institute, Cary, NC, 2002). Pearson’s correlation between the pH and LAB in the fermented snack during fermentation process was also assessed. A p < 0.05 was considered statistically significant.
RESULTS AND DISCUSSION
Changes in pH and LAB population of the snack samples during fermentation process were presented in Fig. 1. The pH of the fermented snack decreased from 6.28 to 5.08 during the first 12 h of fermentation, and further declined to 4.76 at the end of fermentation. The initial LAB numbered 6.2 × 107 CFU/g and dramatically increased to 2.8 × 109 CFU/g by the time of 12 h fermentation. Based on this result, it is expected that the decrease in pH was secondary to the increased production of lactic acid contents by LAB during fermentation. The inverse relation (r = –0.96) (Fig. 1) between pH and lactic acid seen in this study has been well documented [13,14].
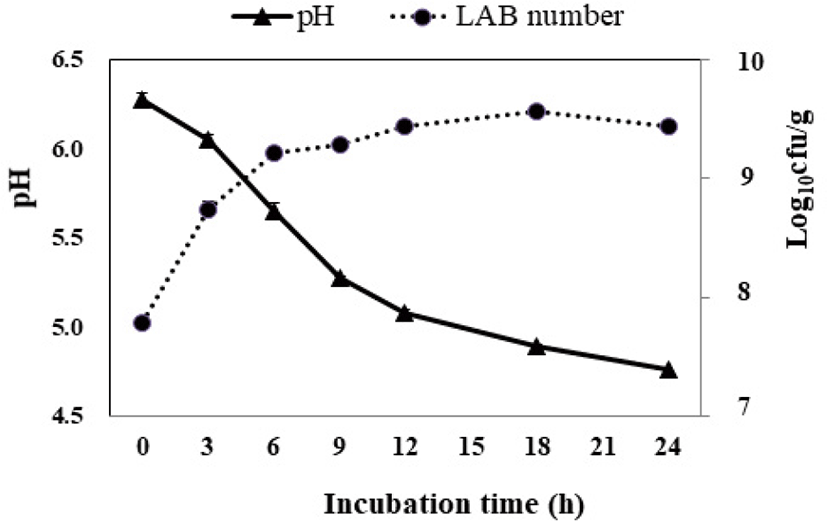
Chemical compositions of fermented and non-fermented snack samples were shown in Table 2. Amino acid and fatty acid compositions were presented in Tables 3 and 4, respectively. Overall, fermented and non-fermented snack samples were not different in the analyzed nutrient compositions. However, fermented vs. non-fermented snack contained higher lactic acid and ammonia concentration, but had lower pH. It was shown that lactic acid concentrations increased from 2.5 mg/g to 6 mg/g when pork was fermented with LAB at 20°C for 48 h [15]. Bruna [16] reported that ammonia contents increased approximately 200% when the surface of sausage batch was sprayed with Penicillium camemberti and fermented at 22°C to 18°C for 72 h. Xu [17] also reported that protein is decomposed to peptides and amino acids. Thus, the latter two studies explained the increased ammonia concentration in the fermented snack seen in this study.
In vitro pepsin digestibility in the fermented vs. non-fermented snacks was increased (Fig. 2), of which observation could provide the evidence that the fermentation can improve nutritive value. However, proximate analysis showed that fermentation did not affect the chemical compositions, amino acids and fatty acids.
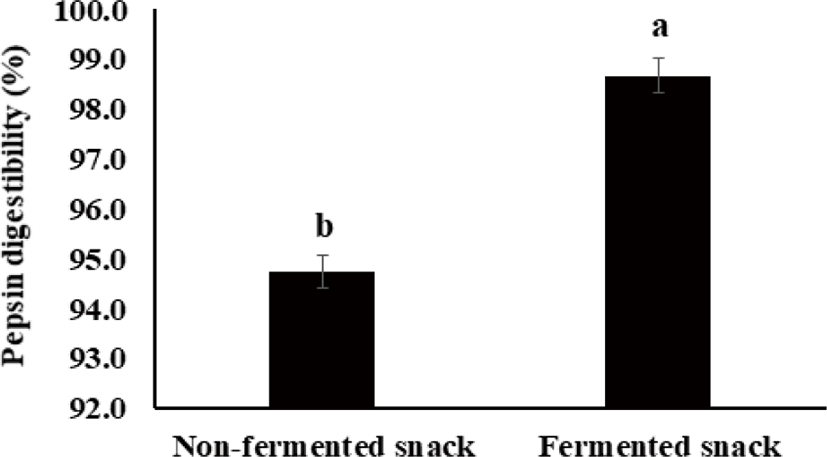
Fig. 3 shows the preservability of the fermented and non-fermented snacks. The total bacteria of the non-fermented snacks started to appear on the first and the second day after exposure, respectively, and they increased linearly up to the 7th day, reaching at 5.23 log10 CFU/g. Lactic acid bacteria grew slowly, and kept lower in number compared with the total and pathogenic bacteria. On the other hand, total bacteria of the fermented snack reached to 3.18 log10 CFU/g on the 14th day of exposure which was substantially lower than that of the non-fermented snack. Lactic acid bacteria appeared after the 7th day of exposure. Colonies in Orientation agar plate were formed with non-fermented snack sample, but color-identifiable pathogen-specific colonies were not detected. No single colony on Orientation agar plate was found with the fermented snack during the exposure. Based on this result, it is likely that organic acids, or bacteriocins, if any, present in the fermented snack could inhibit or delay the growth of bacteria. It is well documented that LAB could rapidly acidify the raw materials during fermentation, through the production of organic acids, mainly lactic acid and acetic acid, and also produce a variety of antimicrobial substances, which can consequently prevent the growth of the most hazardous food microorganisms [18].
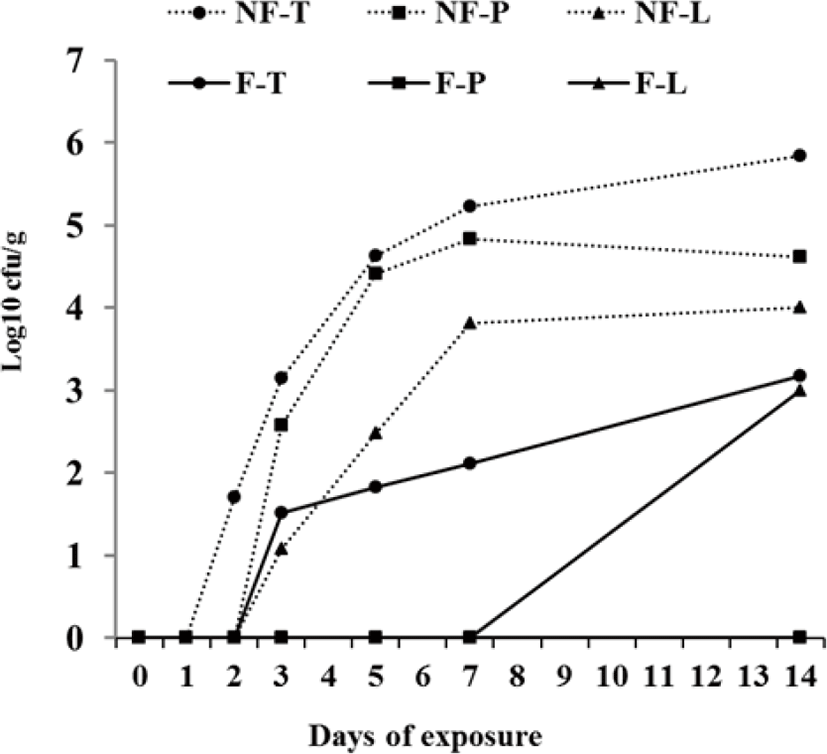
In palatability trial, dogs provided with the fermented vs. non-fermented snacks ate less, spent less time, and were reluctant to eat (p > 0.05) which was manifested by lowered frequency in smelling and eating (Table 5). The first choice of the non-fermented snack was significantly higher (p < 0.05) than that of the fermented snack. Based on these results, it seems that the palatability of the fermented snack is low due to the strong odor of fermentation as indicated elsewhere [19]. Alternatively, low pH of the fermented snack per se may have reduced its palatability which was the case with pigs [20]. In any events, our study indicates that additional measures such as supplementation of natural flavors are needed to increase palatability of the fermented snack.
Dogs were fed with fermented or non-fermented snack foods for 12 days after 7-day transition period from the basal to the experimental food. During the trial, all dogs fed fermented or non-fermented snack food gained weight by 450 g and 340 g (Table 6). Dogs fed fermented vs. non-fermented snack ate more, but drank less, albeit that the difference was not statistically different. It is likely that low voluntary water intake in dogs fed fermented snack was secondary to the increase in involuntary water intake by consuming more moisture-rich snack. In this study, dogs preferred non-fermented vs. fermented snacks in the palatability trial while those offered fermented snack ate more compared with non-fermented snack-fed dogs in the 12-d-feeding trial. In addition, there was large variable in feed intake between individuals especially fed the non-fermented snack in the feeding trials. At this stage, a clear explanation is not readily available, but it seemed that individual dog differently responded to fermented snacks depending on the degree of satiety and/or feeding duration which needs to be answered.
Urine production was comparable between the treatment groups (Table 6). Ammonia concentration in feces was significantly lower in dogs fed fermented snack compared with those fed non-fermented snack. In contrast, fecal lactic acid content was significantly elevated in fermented snack-fed dogs compared with those fed non-fermented snack. However, elevated lactic acid in feces of the fermented vs. non-fermented snack did not affect fecal pH. Decreased ammonia content and increased lactic acid in feces induced by dietary fermented snack may be in part secondary to balanced gut microbiota, i.e., LAB which could then inhibit pathogenic bacteria [21].
CONCLUSION
In this study, we manufactured Pediococcus spp.-fermented MDCM-based snack food for dogs. The nutritional compositions of the snack were not significantly altered by fermentation. However, in vitro pepsin digestibility was significantly increased by fermentation. Microbiological assay revealed that bacteria growth in fermented snack exposed to environment was delayed. In a palatability trial, dogs preferred non-fermented over fermented snack. In a feeding trial, fecal ammonia content was reduced, but fecal lactic acid content was increased in dogs fed fermented vs. non-fermented snack food. Collectively, the fermented MDCM-based snack for companion dogs can be used as a digestible snack with favorably long-term preservability. To our knowledge, this is the first report to show the possibility for utilizing the poultry by-product MDCM by fermentation process as a value-added snack for dogs, of which is considered of importance to companion animal industry.