INTRODUCTION
Recently, ruminants’ production has been challenged with significant environmental issues [1]. These challenges have included feed production, transport, and utilization, which are responsible for environmental problems such as greenhouse gas emissions [2]. Wilkinson [3] reported that the conversion of human-inedible input into human-edible output could be shaped to improve efficiency and achieve sustainability in the ruminant industry. These ideas are supported by the ability of ruminants to convert inedible input, such as crop residues or agricultural byproducts, into high-protein edible products, such as meat and milk [4]. Interest in using agricultural byproducts as feed for ruminants is increasing due to these byproducts’ nutritive values, including those of some bioactive compounds that remain even after industrial processing [5].
Persimmon (Diospyros kaki) peel (PP) is a byproduct of dried persimmon manufacturing, while damaged whole persimmons (WP) are ripped or misshaped persimmons that have lost their economic value. During dried persimmon production, a considerable amount of peel is produced as waste, as are damaged and commercially unavailable persimmons [6]. Most farmers in the dried persimmon industry discard these byproducts or utilize them as fertilizer. However, we speculated that these two byproducts might have potential as sources of ruminant feed in their base forms or with minimal processing, facilitating the production of the meat or milk of the ruminants. Previous studies have reported the use of PP as animal feed. Lee et al. [6] demonstrated the beneficial effects of PP for crossbred pigs. The fatty acid and cholesterol concentrations improved without any remarkable changes in growth performance or meat quality. PP also exhibited the beneficial effects of improving egg quality and layer hen performance in a study conducted by Oh et al. [7]. PP silage showed no negative effect on feed intake or palatability when fed to sheep as 20% dry matter (DM) partial substitution of tall fescue [8]. Another study showed that when PP was added to total mixed ration (TMR) silage, it could resist protein breakdown during ensiling [9]. WP have the same composition as ordinary persimmons, including bioactive compounds such as sugars, vitamins, tannins, dietary fiber, carotenoids, minerals, amino acids, and lipids [10,11]. The benefits of persimmons have been studied in humans in order to treat several diseases, such as cardiovascular disease, hypertension, diabetes, and cancer [12].
Similarly to other agricultural byproducts, these byproducts have high moisture contents that are vulnerable to energy loss during storage [13], as well as to the development of mold. Therefore, in this study, we mixed these byproducts with rice straw. Rice straw has a high DM content but is low in nutritive value and ruminant palatability [14,15]. Some studies have shown that ensiling rice straw with other agricultural byproducts has the potential to enhance the quality of the silage and have beneficial effects on animals [16–18]. We hypothesized that ensiling dried persimmon byproducts with rice straw would enhance the nutritive value of the mixed silages. Therefore, this study evaluated the chemical compositions, digestibility, and palatability of PP and WP, which are byproducts of the dried persimmon industry, ensiled together with rice straw at various ratios in order to be used as ruminant feed.
MATERIALS AND METHODS
Persimmon peel (PP) and damaged WP were collected from local dried persimmon industries in Sangju-si, Gyeongsangbuk-do (refer to Table 1 for the chemical compositions of the samples). These byproducts were divided into eight treatments and mixed with rice straw at ratios (DM basis, Table 2) of 3:7 (PP3R7, WP3R7), 5:5 (PP5R5, WP5R5), 7:3 (PP7R3, WP7R3), and 8:2 (PP8R2, WP8R2). Each of the treatments was prepared in a 30 kg quantity, anaerobically packed into 20 L plastic containers in triplicate, and ensiled for 70 d.
Treatment1) | Mixing ratio (DM basis) | ||
---|---|---|---|
Persimmon peel (PP) | Damaged whole persimmons (WP) | Rice straw (R) | |
PP3R7 | 3 | - | 7 |
PP5R5 | 5 | - | 5 |
PP7R3 | 7 | - | 3 |
PP8R2 | 8 | - | 2 |
WP3R7 | - | 3 | 7 |
WP5R5 | - | 5 | 5 |
WP7R3 | - | 7 | 3 |
WP8R2 | - | 8 | 2 |
After a 70 d ensiling period, the silages were removed, homogenized, and randomly sampled using the coning and quartering method [19]. An aliquot of 300 g of the samples, was oven-dried at 65°C for 3 d to obtain the initial DM value. The dried samples were weighed and ground so they could pass through a 1 mm screen using an ultra-centrifugal mill (ZM 2000, Retch, Haan, Germany), and each sample was used for proximate analysis and determination of tannin content. Another 100 g of the fresh samples was mixed with 900 mL of distilled water and homogenized using a stomacher (Daihan Scientific, Wonju, Korea) for 4 min. The supernatant of each sample was used to determine the silage pH; and the ammonia-N, lactate, and acetate concentrations.
The DM (AOAC: 934.01), organic matter (OM; AOAC: 942.05), crude protein (CP; AOAC: 984.13), and ether extract (EE; AOAC: 920.39A) were analyzed according to AOAC [20] methods. An ANKOM2000 fiber analyzer (ANKOM Technology, Macedon, NY, USA) was utilized to determine the neutral detergent fiber (NDF; AOAC: 2002.04) and acid detergent fiber (ADF; AOAC: 973.18) contents [20,21]. The tannin content was analyzed according to the method used by Price and Butler [22]. The ammonia-N concentration was measured with the method used by Chaney and Marbach [23]. The lactate concentrations of the silages were analyzed using high-performance liquid chromatography (Series 200; Perkin Elmer, Waltham, Massachusetts, USA) with a 300 × 7.8 mm Aminex® HPX-87H column (Bio-Rad Laboratories, Hercules, California, USA). A total of 0.008 N H2SO4 was utilized as a mobile phase, with a flow rate of 0.6 mL/min; the column oven was set at 35°C and detection was performed at a wavelength of 210 nm.
Gas chromatography (450-GC, Bruker, Bremen, Germany) with a built-in BR-Wax fame column (BR87503, Bruker, Massachusetts, USA) was utilized to analyze acetate concentrations [24]. The temperatures of the flame ionization detector, injector, and oven were set at 250°C, 250°C, and 100°C, respectively. The airflows of nitrogen, hydrogen, and high-purity air were set at 29, 30, and 300 mL/min, respectively.
The brix degrees (°Bx) of the silages were measured using a PR-101 sugar degree meter (Atago, Tokyo, Japan), while the fructose, glucose, dextran, isomerase, and inverted sugar contents were measured using PALS-14S, PALS-15S, PALS-12S, PALS-16S, and PALS-18S digital refractometers (Atago), respectively. The measurements were conducted using liquid that had originated from the compressed silages.
The silage quality was measured, based on the fermentation products, with Flieg’s score and Flieg’s point. Flieg’s score was obtained based on the lactate, acetate, and butyrate proportions in the silage according to the standard set by Woolford [25]. Flieg’s point was calculated using Kilic [26] equation:
To determine the dry matter digestibility (DMD) of both the PP and WP silages, in vitro rumen fermentation was conducted according to the method used by Tilley and Terry [27]. An aliquot of 0.5 g of dried and ground sample was placed in a 125 mL serum bottle in triplicate, mixed with 50 mL of filtered rumen fluid combined with McDougall’s buffer [28] at a ratio of 1:4, and incubated at 39°C for periods of 6 and 24 h incubation period. The rumen fluid was obtained from two Korean native cattle fed with rice straw silage (60.9% DM, 1.3% EE, 71.6% NDF, and 46.7% ADF) and concentrate (92.5% DM, 20.4% CP, 4.0% EE, 38.7% NDF, and 19.4% ADF) ad libitum, using a stomach tube before morning feeding, and kept on thermos bottles during transportation to the laboratory. It was mixed, filtered with 4 layers of muslin, heated to 39°C in a water bath, and bubbled with CO2 to obtain an anaerobic condition. The maize silage (MS), rice straw silage (RS), whole-crop rye silage (WCRS), and sorghum-sudangrass silage (SSGS) were chosen for comparisons (refer to Table 3). At each interval, samples were removed from the incubator to determine their total gas production, DMD, pH, and ammonia-N. The total gas production was measured using a 100 mL glass syringe (Z314595, Fortuna® Optima®, Merck, Darmstadt, Germany) connected to 3-way stopcock (Sewoon Medical, Cheonan, Korea). The substrate was filtered using 5 × 10 cm nylon bags (R510 concentrate bags, ANKOM Technology) and dried for 24 h at 105°C [15,29]. The supernatant was utilized to analyze the pH, volatile fatty acids (VFA), and ammonia-N concentrations. The VFA produced during the in vitro rumen fermentation was analyzed according to Erwin et al. [24]. While for the ammonia-N analysis, the method used by Chaney and Marbach [23] was employed.
A palatability study was conducted using 3 Hanwoo cattle, 3 Korean black × boar goats, and 3 Korean spotted deer through the cafeteria feeding approach for 10 h for 4 consecutive days [30]. The relative palatability index (RPI) was determined according to the method described by Abdulrazak et al. [31].
The results of the proximate and chemical analyses of the silages were subjected to a two-way analysis of variance (ANOVA) by employing general liner models of the SPSS program (version 25, IBM, Madison, NY, USA). In addition, one-way analysis of variance was applied for the in vitro and palatability results. Comparisons among the means were conducted using Duncan’s multiple-range test.
RESULTS
The chemical compositions and nutritive values of the persimmon byproducts combined with rice straw silage are presented in Table 4. After 70 d of ensiling, all of the replicates of WP8R2 treatment were contaminated with fungi; thus, they have been removed from the subsequent analyses. The type of persimmon byproduct (PP or WP) did not significantly affect the DM content. Nevertheless, it significantly affected (p < 0.05) the concentrations of OM, CP, EE, lactate, acetate, and ammonia-N. The mixing ratios of the persimmon byproducts and the rice straw had significant differences (p < 0.05) in almost all chemical composition content, except for the EE content.
1) PP3R7, PP5R5, PP7R3, PP8R2, persimmon peel mixed with rice straw at ratios of 3:7, 5: 5, 7:3, and 8:2, respectively; WP3R7, WP5R5, WP7R3, damaged whole persimmons mixed with rice straw at ratios of 3:7, 5:5, and 7:3, respectively.
Based on calculations of the organic acid produced after a 70 d fermentation period, the WPs showed a higher (p < 0.05) Flieg score compared to the PP. Interestingly, the lowest (p < 0.05) score was present in the silage with persimmon byproducts and rice straw mixed at a ratio of 2:8. In contrast with Flieg score, the type of byproduct did not affect the Flieg point. However, the lowest Flieg point (p < 0.05) was demonstrated by the silage of persimmon byproducts and rice straw mixed in 2:8 ratios. Nevertheless, the prepared silages were categorized as good quality.
The sugar contents of the mixed persimmon byproducts and rice straw silages are shown in Table 5. The rice straw ensiled with added PP have higher (p < 0.05) sugar contents compared to that ensiled with WP. The °Bx values of the silages are significantly different. Treatments PP5R5 and PP7R3 have the highest (p < 0.05) °Bx, fructose, dextran, and inverted sugar percentages compared to the other treatments. The silages mixed at a byproducts to rice straw ratio of 5:5 show the highest (p < 0.05) sugar contents compared to the others.
1) PP3R7, PP5R5, PP7R3, and PP8R2, persimmon peel mixed with rice straw at ratios of 3:7, 5: 5, 7:3, and 8:2, respectively; WP3R7, WP5R5, and WP7R3, damaged whole persimmons mixed with rice straw at ratios of 3 7, 5: 5, and 7:3, respectively.
The in vitro rumen fermentation characteristics of the rice straw ensiled with persimmon byproducts compared to those of the MS, RS, WCRS, and SSGS are described in Table 6. At 6 h of incubation, PP7R3, PP8R2, and WP7R3 showed the highest (p < 0.05) DMD compared to the other treatments, and these values are higher than those of the MS, RS, WCRS, and SSGS. Meanwhile, at 24 h of incubation, PP8R2 had the highest (p < 0.05) DMD among the mixed persimmon byproduct and rice straw silages; which is lower (p < 0.05) than those of the maize, whole-crop rye, and sorghum-sudangrass silages but higher (p < 0.05) than the rice straw silage. There was no significant difference in the supernatant pH at 6 h of incubation. However, after the 24 h incubation period, WP3R7 had the highest pH compared to the others. Nevertheless, the pH, ranging from 6.88 to 6.99, was within the normal range for ruminants The total gas production after the 6 h incubation period was different between the treatments. At 24 h, PP8R2 demonstrated the highest (p < 0.05) total gas production compared with the other mixed byproduct and rice straw silages; this is lower (p < 0.05) than those of the maize and whole-crop rye silages but higher (p < 0.05) than the rice straw and sorghum-sudangrass silages. All of the treatments showed lower (p < 0.05) ammonia-N concentrations compared to the maize, whole-crop rye silage and sorghum-sudangrass silages after a 6 h incubation period. However, after a 24 h incubation period, all of the persimmon byproduct silages had comparable ammonia-N concentrations to the maize and rice straw silages.
The total VFA tended to be higher (p = 0.070) in PP5R5, PP8R2, WP5R5, and WP7R3 compared to the maize and whole-crop rye silages at 6 h of incubation (Table 7). However, after 24 h, PP8R2 and the maize silage showed the highest (p < 0.05) total VFA concentrations compared to the others.
The RPIs of the mixed persimmon byproduct and rice straw silages compared to the rice straw silage are presented in Fig. 1. When the silages were offered to deer, the RPIs were significantly different, with the following rankings: PP8R2 < PP7R3 < WP7R3 < RS, PP3R7, PP5R5, WP3R7, and WP5R5. With goats, the RPIs were in the following order: PP7R3 < RS, PP5R5, PP8R2 < PP3R7, WP3R7, WP5R5, and WP7R3. On the other hand, Hanwoo cattle preferred PP7R3, PP8R2, PP5R5 < P3R7, WP3R7 < RS, WP5R5, and WP7R7. Overall, PP7R3 had the highest palatability compared to the rice straw and the other treatments.
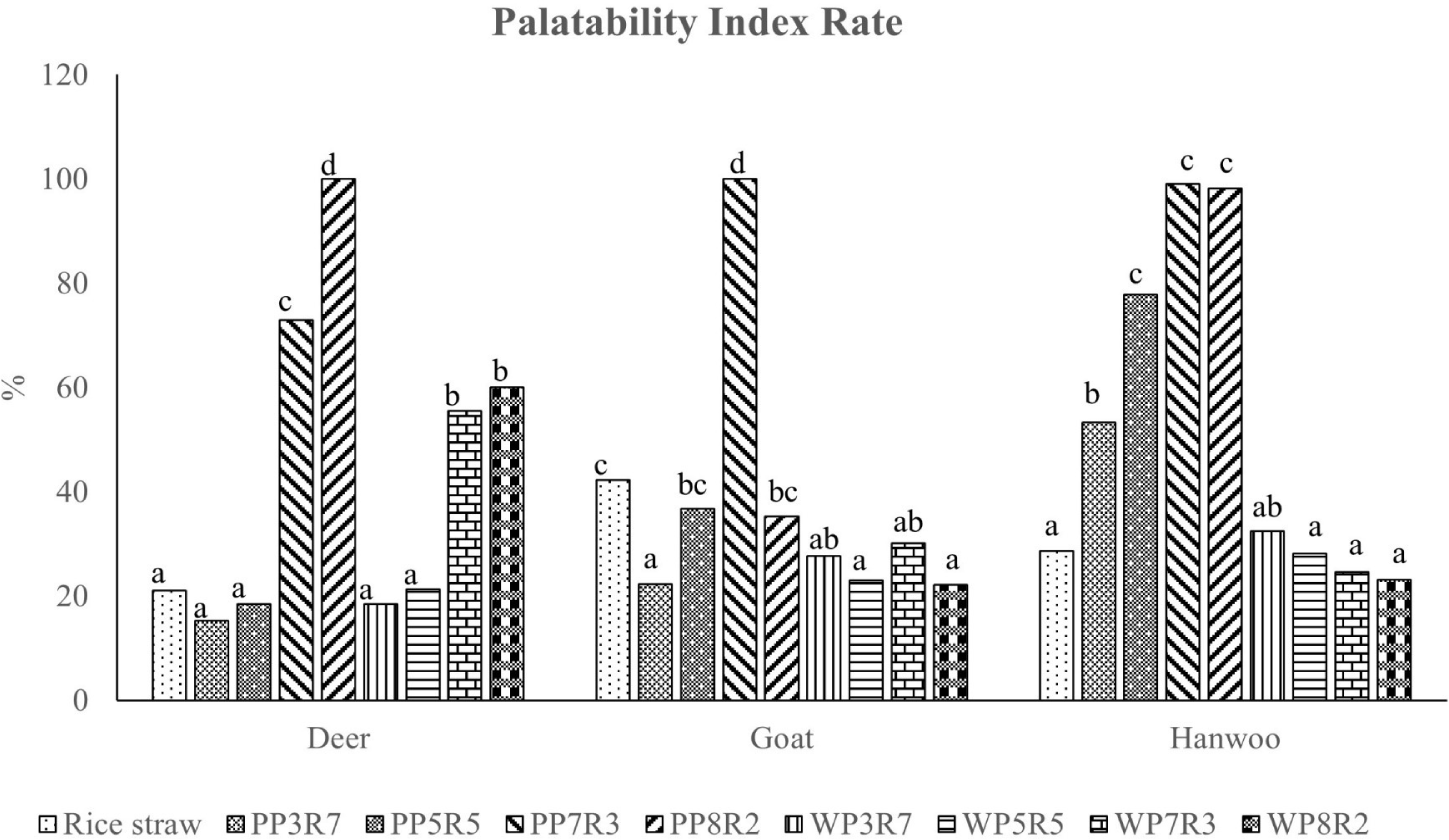
DISCUSSION
Ensiling fruit byproducts with agricultural byproducts such as rice straw is a method used to produce feed with desirable nutrients [32]. In this study, we ensiled persimmon byproducts with rice straw in several mixing ratios for 70 d. The addition of the persimmon byproducts appeared to give lower DM contents compared to the plain rice straw silages [15,33,34], particularly for those with higher amounts of persimmon byproducts which initially had a higher moisture content. For instance, a study by Xue et al. [35], of ensiled rice straw and banana byproducts showed similar results. The WP and rice straw failed to be ensiled at a ratio of 8:2 due to fungal spoilage. We hypothesized that silage that contains more persimmon byproducts would tend to be spoiled by fungi due to the high moisture contents. Gallo et al. [36] mentioned that some fungi, such as Aspergillus spp., have been reported in silage with high moisture.
The ensiling of persimmon byproducts with rice straw increased the CP contents of the silages compared to the original rice straw silages [15,18,34]. Adding agricultural byproducts to rice straw silage has been proven to increase its CP content [35,37]. In this study, WP silages showed higher CP content compared to those with PP. Numerically, the original WP showed higher CP compared to the PP (WP: 3.94%, PP: 3.62%), which can be the reason for the higher CP content of the silages. Thus, the higher WP ratios contents (WP7R3) showed the highest (p < 0.05) CP compared to other treatments. However, during the ensiling period, when proteolysis usually occurs, the rate of this process can be defined by the ammonia-N concentration in the silage [38]. Therefore, the ammonia-N concentration is generally used as an indicator of silage quality [39]. A higher ammonia-N concentration is associated with poor fermentation quality of silage due to Clostridium fermentation and often results in decreased intake [39,40]. However, in our study, the ammonia-N concentration in the silage ranged from 0.2 to 0.5 g/kg total N, which is considered to be good quality [41]. Additionally, a study by Fitri et al. [9] mentioned that addition of PP to TMR silage can decrease proteolytic enzymes during the ensiling period due to the tannins contained in the PP, which have a high affinity to bind with protein and protect against proteolysis [8].
On the other hand, the addition of the persimmon byproducts significantly reduced the NDF and ADF contents during the ensiling. One of the reasons for this might be the xyloglucan endotransglycosylase/hydrolase (XTH), which is responsible for cell wall softening, in persimmon [42]. Several other studies have mentioned that fermentation that occurs during the ensiling process can reduce the NDF and ADF contents [43,44]. Morrison [45] mentioned that silage microflora also produces cellulase and hemicellulose enzymes, which results in some fiber loss in silage.
The pH values of the mixed persimmon byproduct silages in this study ranged from 3.89 to 4.20, which is lower than the general pH of rice straw silage [34,46] and fulfills one of the desirable silage characteristics mentioned by McDonald et al. [47]: a pH of 4.2 or less. The pH of the silage mainly resulted from the organic acid produced during the ensiling and became an indicator of the silage fermentation quality [47,48]. In the current study, increased concentrations of lactate and acetate were noted with increased ratios of persimmon byproducts. In addition, lactate in the silage could preserve it and was mainly responsible for its pH dropping [48]. Another point to consider is the DM content of the silage; Kung and Shaver [48] indicated that high DM content is associated with lower lactate content in silage. The mixing ratios of the treatments provided significant differences between the lactate concentrations in the present study. Treatments with ratios featuring more rice straw than persimmon byproducts showed lower lactate concentrations than those with a lower rice straw to persimmon byproduct ratio. The pH of the mixed persimmon byproduct silage in the current study also supports the study conducted by Muck [49], which mentioned that lower pH is related to the moisture content of silage. The mixing ratio affected the pH primarily due to the moisture contents of the silages. For instance, treatment PP8R2, which showed the lowest pH compared to the other treatments, had the highest (p < 0.05) moisture content.
In this study, we did not determine the sugar contents of the raw materials (i.e., PP and WP). However, based on previous studies, we can estimate that the sugar content of the WP was around 11–20°Bx [50–52], while that of the PP was around 4.8°Brix [53]. The fermentation process plays an essential role in reducing sugar molecules and converting them into organic acids during the ensiling period [54], as shown by the relatively low sugar contents in the silages after the 70 d. The differences in the sugar contents of the byproduct types might be the reason that there were significant differences in the lactate and acetate concentrations of the silages. During the ensiling period, the fermentation of sugars, contained in the forage crop or substrate, by microorganisms, mostly epiphytic lactic acid bacteria, occurred [48].
The in vitro digestibility of the persimmon byproduct mixed with rice straw at the ratios of 7:3 and 8:2, at 6 h of fermentation was higher compared to those of the silages typically fed to ruminants. The results in the current study are in agreement with previous studies using agricultural byproducts [41,55]. This result reflects the NDF and ADF contents of the silage. Gasa, Castrillo [56] reported that the in vitro digestibility reflected the NDF content of the feed in their study. In addition, Getachew et al. [57] demonstrated the negative effect of the NDF content on digestibility. In this study, the persimmon byproduct mixed with rice straw at the ratios of 7:3 and 8:2 showed lower NDF contents compared with the other treatments.
At 6 h of incubation, the total VFA concentrations of the mixed dried persimmon byproduct and rice straw silages were comparable with those of the typical silages fed to ruminants. After 24 h of incubation, most of the silages had higher total VFA compared to the rice straw silage. The PP mixed with rice straw at the 8:2 ratio was similar to maize silage in this regard. This might relate to the sugar contents contained in mixed dried persimmon byproduct and rice straw silages. VFA is generated as the end product of fermentation in the rumen and significantly affects ruminants’ production [58]. Based on our in vitro results, adding dried persimmon industry byproducts to rice straw appears to have the potential to enhance the quality of in vitro rumen fermentation. Previous studies using agricultural byproducts have reported similar results of improved feed digestibility. For example, a study by Li et al. [17] used sweet potato vines as an additive in ensiling rice straw, and a study by Foiklang et al. [59] used grape pomace as an additive reported improved DM digestibility.
The number of animals used in the palatability test in this study did not fulfill the requirement set by Meier et al. [60]. Nevertheless, the results showed perspicuous effects of silage byproducts on the RPI. When offered to the ruminants, the mixed dried persimmon and rice straw silages, especially the PP treatments mixed at byproduct to rice straw ratios of 7:3 and 8:2, showed a higher RPI than rice straw silage. The authors of previous studies that used vegetable and fruit byproducts as additives to ruminant feed reported similar results [32,61]. Provenza [62] reported that ruminants generally select food based on their nutritional needs and tend to avoid toxin-contaminated food. In addition, Baumont [63] also mentioned that ruminants prefer feeds which can provide high satiety levels rapidly. The high RPI of PP silages at 7:3 and 8:2 ratios provide evidence that these silages may be considered nutritious feeds. Both of these PP silages have relatively low NDF and ADF contents, high lactate, acetate, and sugar contents.
In this study, dried persimmon byproducts mixed with rice straw at several ratios showed potential to be used in ruminant feeds. The chemical compositions of the mixed silages were improved as the ratio of dried persimmon byproduct to rice straw increased. During the in vitro rumen fermentation, the DMD and the total VFA concentration of the dried persimmon byproduct and rice straw silages mixed at the ratios of 7:3 and 8:2 were comparable to those of silages usually fed to ruminants, such as maize, whole-crop rye, and sorghum-sudangrass. In addition, when offered to ruminants such as Hanwoo cattle, Korean native goats, and Korean spotted deer, the mixed dried persimmon and rice straw silage showed a higher RPI than the rice straw silage. Further studies of the rumen, total-tract digestibility, and production may be necessary. A further study, related to the economic aspects of incorporation of the persimmon-byproducts-based silages and their effect on greenhouse gas emissions, may also be necessary.