INTRODUCTION
Vitamin D3 (cholecalciferol, VD3) is a nutrient for animal growth, health, and bone development. Its deficiency damages bone quality, while the addition of VD3 improves growth performance and blood calcium (Ca) and phosphorus (P) homeostasis in broiler chickens [1]. The VD3 requirement of 1–21-day-old broiler chickens estimated by NRC is 200 IU/kg [2]. The recommended dietary VD3 level for broilers in China is 1,000 IU/kg [3]. Research has shown that 1,000–2,000 IU/kg VD3 is needed to support the growth performance and leg bone mineralization of broilers [4]. Thus, the requirement of modern broilers for VD3 may be higher than that recommended by NRC [2]. The primary function of VD3 is to regulate the absorption of Ca and P in animal intestines. Ca and P are the two mineral elements that are added most in feed and needed most in animal body. Research in mammals has shown that the response of intestinal Ca and P absorption of rats to vitamin D correlates with the gene expression of Ca and P transporters [5,6].
Calcium is absorbed through active transcellular transport and passive transport in animal enterocytes [7]. The transcellular transport of Ca involves three procedures: Ca enters intestinal cells, moves to the basolateral membrane, and extrudes into the blood [7]. The first procedure is intestinal Ca transport, which relies on transient receptor potential channels (TRPV6 and TRPV5) [7]. The second procedure is the movement of Ca in the cytoplasm, which depends on two Ca-binding proteins (i.e., CaBP-D28k and CaBP-D9k) [8]. The final procedure is the extrusion of Ca, which is performed through sodium-calcium exchanger 1 (NCX1) and plasma membrane calcium ATPase 1b (PMCA1b) [8]. CaBP-D28k and CaBP-D9k exist in the intestines of poultry and mammals, respectively. Intestinal TRPV6, CaBP-D28k, and PMCA1b have been cloned in laying hens [9,10]. There is no report of TRPV6 in broilers [11].
Phosphate transport from the brush border membrane of intestinal enterocytes into the cytoplasm is an active absorption process. Three P transporters, namely, IIb sodium-phosphate cotransporter (NaPi-IIb), inorganic P transporter 1 (PiT-1), and inorganic P transporter 2 (PiT-2), are involved in active P absorption in animal intestines [8]. The mRNA abundances of NaPi-IIb, PiT-1, and PiT-2 have been detected in the apical membrane of rat intestinal cells [12,13]. NaPi-IIb undertakes the majority of P absorption in mouse intestines, whereas, PiT-1 and PiT-2 assume a minor function in total P uptake [14]. These three P transporters have been cloned in broiler intestines [15].
The active absorption of Ca and P in the intestines of broilers relies on the existence of Ca and P transporters. The capacity of P absorption and gene expression abundance of NaPi-IIb in the duodenum are higher than those in the jejunum and ileum of broiler chicks [16]. The difference in protein abundance of duodenal, jejunal, and ileal CaBP-D28k has been noted in laying hens [17]. By contrast, the spatial expression characteristics of CaBP-D28k, PMCA1b, NCX1, PiT-1, and PiT-2 in the three intestinal segments of broilers have not been reported.
The injection of 1,25-dihydroxycholecalciferol (1,25-(OH)2-D3) increases blood Ca and P concentrations and improves Ca and P absorption by upregulating the protein expression of CaBP-D9k and the mRNA abundance of NaPi-IIb in rat intestines [6,18]. Meanwhile, the relationship between dietary VD3 dosage and the expression of Ca and P transporter genes in broiler intestines has not been clarified.
Thus, two experiments were conducted in this research. First, the differences in gene expression levels of Ca and P transporters in the duodenum, jejunum, and ileum were explored. Second, the response of the mRNA abundances of intestinal Ca and P transporters to dietary VD3 concentrations in broiler chickens was examined.
MATERIALS AND METHODS
The animal experiment procedures in this research were implemented in accordance with the guidelines of the Animal Ethics Committee of Shangqiu Normal University (2020-1012).
Experiment 1 investigated the spatial expression characteristics of Ca and P transporters in the duodenum, jejunum, and ileum of 21-day-old broilers. One-day-old Arbor Acres broiler chickens (70, male) were grouped into five repetitions of 14 broilers per repetition. Broilers were provided with an adequate nutrient diet (Table 1) [3]. On day 21, total 10 broilers (2 chicks per repetition) were randomly selected and euthanized by cervical dislocation. The duodenum (after the gizzard), jejunum (proximal to Meckel’s diverticulum), and ileum (proximal to the ileocecal junction) were isolated. Intestinal mucosal samples from the three segments were scraped with a glass slide, collected in a centrifuge tube, immediately put into liquid nitrogen, and stored in −80°C refrigerator.
1) Trace mineral premix supplied per kg of diet: Fe (FeSO4∙7H2O), 80 mg; Mn (MnSO4∙H2O), 60 mg; Zn (ZnSO4∙7H2O), 40 mg; Cu (CuSO4∙5H2O), 6 mg; I (KI), 0.35 mg; and Se (Na2SeO3), 0.15 mg.
2) Experiment 1, vitamin premix supplied per kg of diet: vitamin A (retinyl palmitate), 4.4 mg; vitamin D3, 25 µg (1,000 IU); vitamin E (DL-α-tocopheryl acetate), 20 mg; vitamin K3 (menadione), 0.5 mg; vitamin B1 (thiamine), 2 mg; vitamin B2 (riboflavin), 8 mg; vitamin B6 (pyridoxine), 3.5 mg; vitamin B12, 0.01 mg; biotin, 0.18 mg; folic acid, 0.55 mg; pantothenic acid, 10 mg; niacin, 35 mg.
3) Experiment 2, vitamin premix (without supplemental VD3) supplied per kg of diet: vitamin A (retinyl palmitate), 4.4 mg; vitamin E (DL-α-tocopheryl acetate), 20 mg; vitamin K3 (menadione), 0.5 mg; vitamin B1 (thiamine), 2 mg; vitamin B2 (riboflavin), 8 mg; vitamin B6 (pyridoxine), 3.5 mg; vitamin B12, 0.01 mg; biotin, 0.18 mg; folic acid, 0.55 mg; pantothenic acid, 10 mg; niacin, 35 mg.
Experiment 2 evaluated the effects of dietary vitamin D3 concentration on growth performance, bone development, and gene expression levels of intestinal Ca and P transporters in 1–21-day-old broilers. One-day-old Arbor Acres broiler chickens (420, male) were randomly grouped into six treatments. Each treatment contained five repetitions with 14 broilers per repetition. Dietary VD3 levels were 0, 125, 250, 500, 1,000, and 2,000 IU/kg. The negative control diet contained 10.0 g/kg Ca and 4.5 g/kg non-phytate P and did not contain supplemental VD3 (Table 1) [2].
The VD3 crystal was supplied by Tianhecheng Biological Technology (Jiaxing, Zhejiang, China). The VD3 solution was prepared in accordance with previous research [19]. After weighing, the crystalline VD3 was dissolved in ethanol. Propylene glycol was used to dilute the VD3 solution. The concentration of the VD3 solution was 45.3 µg/mL (1,812 IU/mL) as measured by high-performance liquid chromatography. The VD3 solution was supplemented in broiler chicken feed with a pipette.
The broilers were housed in pens (width 140 cm, depth 70 cm, and height 35 cm). The broilers were fed with mash feed ad libitum and provided with 23 h of lighting on days 1–3 and 18 h of lighting on days 4–21. The temperature of the room was kept at 32°C on days 1–3, 30°C on days 4–7, and 28°C on days 8–21.
The broilers were weighed by pen at 1 and 21 days of age. The feed intake (FI) per day of the broilers was weighed. The total FI was calculated from 1 to 21 days of age. Body weight gain (BWG) was the difference between the body weight (BW) of the broilers at 21 and 1 day of age. Feed conversion ratio (FCR) was the ratio of the FI to the BWG. The dead broilers during the experiment were weighed and recorded. On day 21, 10 broilers per treatment (2 broilers per repetition) were chosen and euthanized by cervical dislocation. The small intestine was exposed after the broilers were euthanized. Intestinal mucosal samples from the duodenum (after the gizzard), jejunum (proximal to Meckel’s diverticulum), and ileum (proximal to the ileocecal junction) were scraped with a glass slide and collected in a centrifuge tube. Then, the mucosal samples were immediately put into liquid nitrogen and stored in −80°C refrigerator.
The two leg bones (tibia and femur) were removed, stored, and pre-treated [20]. Bone weight and length were measured after the bone was dried at 105°C for 24 h. The ash weights of the tibia and femur were measured after the samples were ashing in a muffle furnace (Selecta, Barcelona, Spain) at 650°C for 48 h. Bone ash, Ca, and P percentages are the ratios of their weight to the bone weight. The Ca contents in the diet and leg bones were analyzed by the AOAC method [21]. Total P contents were measured using the photometric method [22].
Total RNA extraction from the intestinal mucosal samples was implemented by RNAiso Plus Kit on the basis of the recommendation of the manufacturer (Takara Biotechnology, Dalian, Liaoning, China). RNA concentration was analyzed by spectrophotometry.
The cDNA was reverse-transcribed from RNA using PrimeScriptTM RT Reagent Kit based on the instructions of the manufacturer (Takara Biotechnology).
Real-time PCR analysis was conducted for the Ca and P transporter gene expression (i.e., CaBP-D28k, PMCA1b, NCX1, NaPi-IIb, PiT-1, and PiT-2). Glyceraldehyde 3-phosphate dehydrogenase (GAPDH) was used as the reference gene. PCR primers (Table 2) were synthesized in Shanghai Sangon Biotech(Shanghai, China). Gene expression was performed using the TB GreenTM Premix EX TaqTM II Kit (Takara Biotechnology) and the Roche Lightcycler® 480 Real-time PCR System (Basel, Switzerland). The PCR products were verified by melting curve analysis based on the following conditions: 95°C for 60 s, 40 cycles of 95°C for 10 s, 60°C for 30 s, and 72°C for 30 s. The mRNA abundances of the target genes relative to that of the GAPDH gene were calculated by the 2−ΔΔCt method [23]. The average ΔCt value from the negative control diet was used as the calibrator of each gene.
PCR, polymerase chain reaction; bp, base pair; CaBP-D28k, calcium-binding protein 28-kDa; PMCA1b, plasma membrane calcium ATPase 1b; NCX1, sodium-calcium exchanger 1; NaPi-IIb, IIb sodium-dependent phosphate cotransporter; PiT-1, inorganic phosphate transporter 1; PiT-2, inorganic phosphate transporter 2; GAPDH, glyceraldehyde-3-phosphate dehydrogenase.
Repetition pens were used as experimental units. Data analysis was conducted by one-way ANOVA in SAS 9.0 software [24]. Orthogonal polynomials contrast analysis was used to evaluate the linear and quadratic effects of dietary VD3 concentration on growth performance, bone development, and gene expression levels of intestinal Ca and P transporters. Figures were produced with GraphPad Prism software. Means were compared by Tukey’s test. The significance level was set at p < 0.05.
RESULTS
The highest mRNA abundances of the three Ca transporters (CaBP-D28k, PMCA1b, and NCX1) were expressed in the broiler duodenum (Figs. 1A, 1B, and 1C). For P transporters, the mRNA abundance of NaPi-IIb in the duodenum was higher than those in the two other intestinal segments (p < 0.05, Fig. 1D). By contrast, the mRNA abundances of PiT-1 and PiT-2 were the lowest in the duodenum and highest in the ileum (Figs. 1E and 1F).
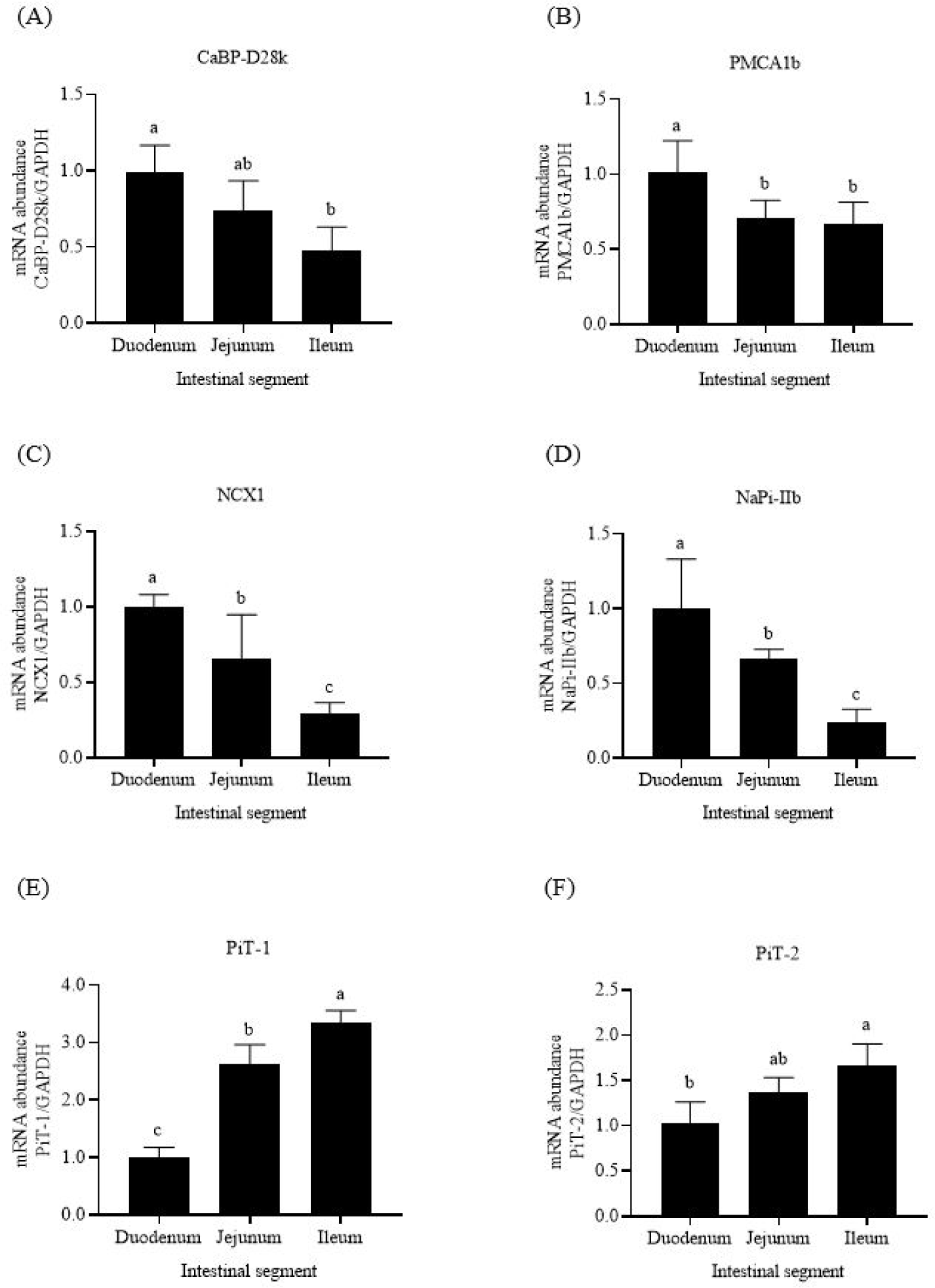
The addition of VD3 increased the growth rate of 1–21-day-old broilers (Table 3). The broilers provided with 125 IU/kg VD3 had higher BW, BWG, and FI, and lower FCR than those supplied with the negative control diet (p < 0.05). Increasing the VD3 level from 125 to 1,000 IU/kg enhanced the BWG and FI (p < 0.05). No difference was detected in the BWG, FI, and FCR of the broilers provided with 1,000 and 2,000 IU/kg VD3 (p > 0.05). Dietary VD3 levels did not affect the mortality of broilers (p > 0.05).
Dietary VD3 improved leg bone (tibia and femur) quality in 1–21-day-old broilers (Tables 4 and 5). The addition of 125 IU/kg VD3 increased the weight, length, and percentage and weight of Ca and P in the two leg bones (p < 0.05). The rise in dietary VD3 levels from 125 to 1,000 IU/kg increased the weights of the ash, Ca, and P (p < 0.05), but it did not influence their contents (p > 0.05). No difference in the weight, length, and percentage and weight of ash, Ca, and P of the tibia and femur was noted in the broilers provided with 1,000 and 2,000 IU/kg VD3 (p > 0.05).
The primary function of CaBP-D28k is to transfer Ca in intestinal cells. Compared with the negative control feed, supplementation with 125 IU/kg VD3 increased the mRNA abundance of intestinal CaBP-D28k in broilers (p < 0.05, Figs. 2A, 3A, and 4A). Increasing the VD3 level from 125 to 1,000 IU/kg enhanced the mRNA abundances of jejunal and ileal CaBP-D28k (p < 0.05). The mRNA levels of duodenal, jejunal, and ileal CaBP-D28k increased by 536, 1,161, and 28 folds, respectively, after adding 1,000 IU/kg VD3. No differences in the mRNA abundances of jenunal and ileal CaBP-D28k were detected between the broilers fed with 1,000 and 2,000 IU/kg VD3 (p > 0.05).
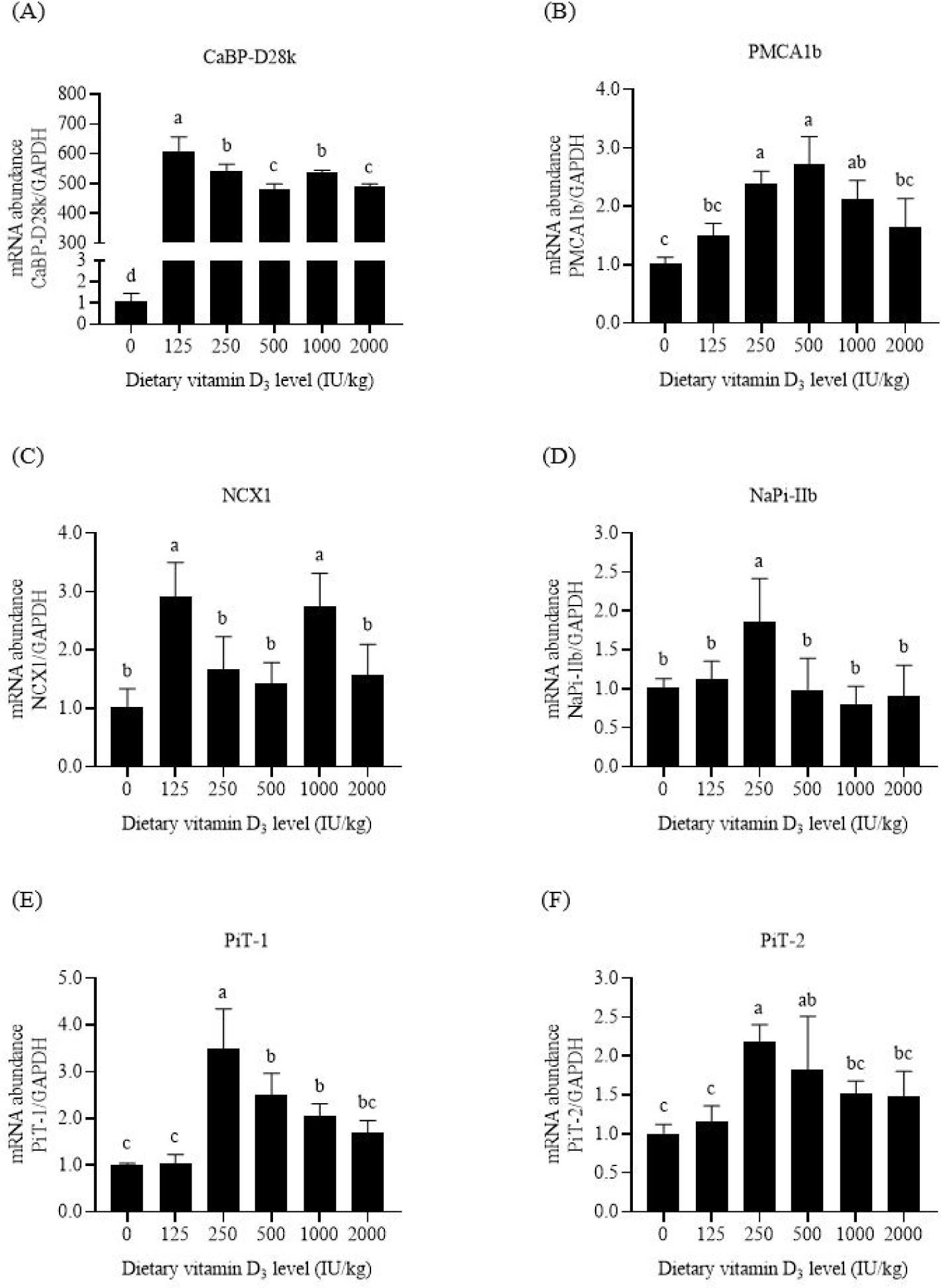
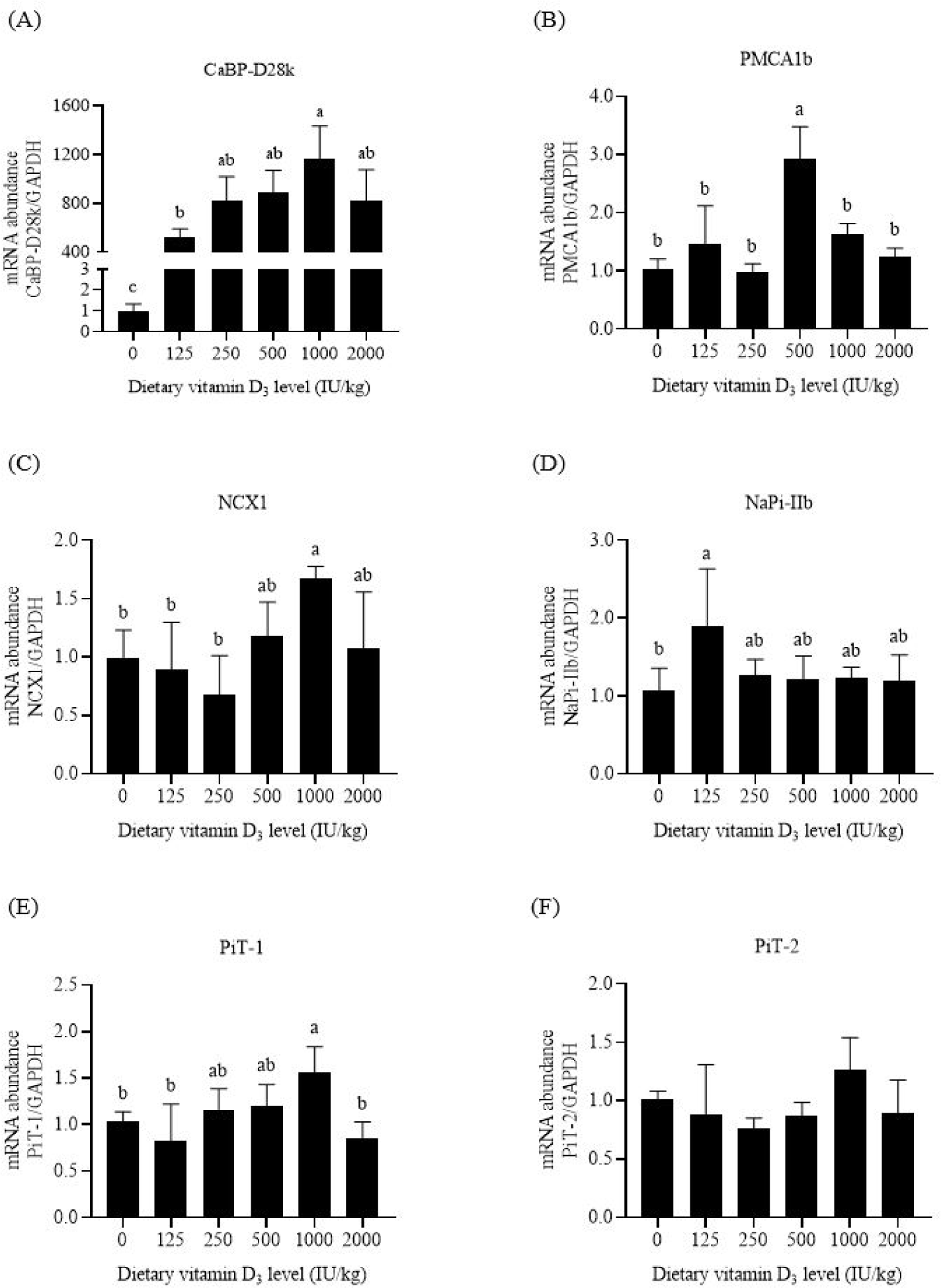
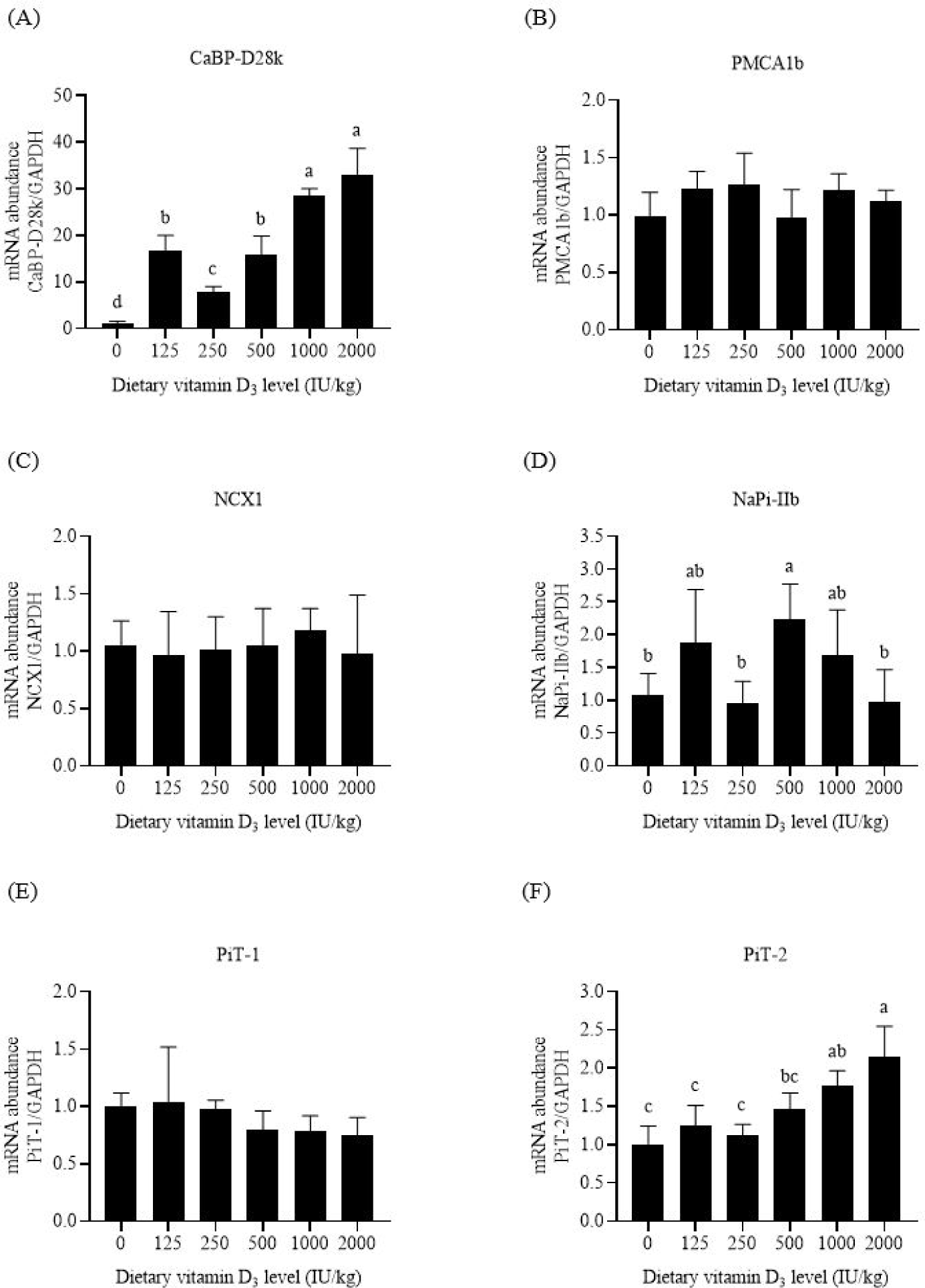
PMCA1b is responsible for Ca extrusion. Dietary VD3 levels upregulated the mRNA abundances of duodenal and jejunal PMCA1b (p < 0.05, Figs. 2B and 3B). The mRNA abundances of PMCA1b in the duodenum of broilers supplied with 250–1,000 IU/kg VD3 and that in the jejunum of broilers supplied with 500 IU/kg VD3 were higher than those in broilers provided with the negative control feed (p < 0.05). Dietary VD3 did not influence the mRNA abundance of ileal PMCA1b (p > 0.05, Fig. 4B).
NCX1 is a sodium and Ca exchanger. The broilers fed with 1,000 IU/kg VD3 had higher mRNA abundances of duodenal and jejunal NCX1 than those supplied with the negative control feed (p < 0.05, Figs. 2C and 3C). Dietary VD3 levels did not affect the mRNA abundance of ileal NCX1 (p > 0.05, Fig. 4C).
NaPi-IIb is the main P transporter. Dietary VD3 enhanced the mRNA abundances of intestinal NaPi-IIb (p < 0.05, Figs. 2D, 3D, and 4D). The mRNA abundance of NaPi-IIb was the highest in the duodenum of the broilers fed with 250 IU/kg VD3, in the jejunum of the broilers provided with 125 IU/kg VD3, and in the ileum of the broilers supplied with 500 IU/kg VD3 (p < 0.05).
PiT-1 is inorganic phosphate transporter 1. VD3 regulated the mRNA abundances of PiT-1 in the duodenum and jejunum (p < 0.05, Figs. 2E and 3E). The mRNA abundance of duodenal PiT-1 was enhanced after the addition of 250–1,000 IU/kg VD3 (p < 0.05), and that of jejunal PiT-1 was increased by 1,000 IU/kg VD3 (p < 0.05). On the contrary, the mRNA abundance of ileal PiT-1 was not affected by dietary VD3 (p > 0.05, Fig. 4E).
The function of PiT-2 is similar to that of PiT-1. The mRNA abundances of duodenal and ileal PiT-2 were enhanced by VD3 (p < 0.05, Figs. 2F and 4F). The mRNA abundance of PiT-2 was higher in the duodenum of the broilers fed with 250–500 IU/kg VD3 and in the ileum of the broilers supplied with 1,000–2,000 IU/kg VD3 than those of the broilers provided with the negative control feed (p < 0.05). The mRNA abundance of jejunal PiT-2 was not affected by VD3 (p > 0.05, Fig. 3F).
DISCUSSION
The duodenum is the first segment of the small intestine, followed by the jejunum and ileum. The mRNA and protein abundances of duodenal CaBP-D28k and PMCA1b are higher than those in the two other segments in laying hens [10,17,25]. Our results were consistent with those of previous reports. The highest mRNA abundances of CaBP-D28k, PMCA1b, and NCX1 were noted in the duodenum of broilers in this research. Thus, the Ca transporters are mainly expressed in poultry duodenum. These data suggest that the capacity of active Ca absorption declines from the duodenum to ileum of poultry.
NaPi-IIb is the primary P transporter in mouse intestines [14]. The mRNA abundances of jejunal and ileal NaPi-IIb are lower than that of duodenal NaPi-IIb in broilers [16,26]. Similar results were observed in this research, and the highest mRNA abundance of NaPi-IIb existed in the duodenum. Thus, the ability of NaPi-IIb to transport P in the duodenum is higher than that in the jejunum and ileum of poultry. PiT-1 and PiT-2 play a smaller role in intestinal P absorption than NaPi-IIb [14]. The mRNA abundance of duodenal PiT-1 is lower than those of jejunal and ileal PiT-1 in laying hens [10]. The present research showed that the highest mRNA abundances of PiT-1 and PiT-2 were observed in the ileum of broilers. PiT-1 and PiT-2 may promote the P absorption in the distal segment of the small intestine of poultry.
Dietary VD3 insufficiency decreases the growth rate of poultry [19]. In this research, the lowest BWG and FI were detected in the broilers provided with the negative control feed. Adding 125 IU/kg VD3 increased the FI and decreased the FCR of broilers. The BWG was increased with the addition of VD3 when more nutrients were retained in the body of broilers. Increasing VD3 concentration from 125 to 1,000 IU/kg significantly elevated the BWG. The recommended VD3 dosage by NRC is 200 IU/kg [2], which can not meet the requirement of broilers for growth rate. A further increase in VD3 level from 1,000 to 2,000 IU/kg did not improve broiler performance in this research. Similar results have been reported [27], in which increasing dietary VD3 level from 1,000 to 7,000 IU/kg does not influence the BW of 1–38-day-old broilers. Thus, it’s necessary to maintain the VD3 levels of broilers at 1,000–2,000 IU/kg.
Ingested Ca and P cannot be effectively absorbed in the blood and deposited in bones of broilers when dietary VD3 is deficient [1]. The lowest Ca and P weights of the leg bones were noted in the broilers provided with the negative control feed in this research. The addition of 125 IU/kg VD3 increased the leg bone Ca and P contents. Ash includes Ca, P, and other minerals. The ash percentage and weight were elevated by the addition of VD3. Our results were in accordance with those reported by previous research [4]. The leg weight and length enhanced with the increase in bone mineral contents after adding 125–1,000 IU/kg VD3. Dietary 200 IU/kg VD3 given by NRC [2] can not meet the needs of bone development of broilers. No difference was noted in the bone quality of broilers provided with 1,000 and 2,000 IU/kg VD3 in this research. Thus, in order to support the leg bone growth and mineralization, 1,000–2,000 IU/kg VD3 should be added to the broiler diets.
CaBP-D28k and CaBP-D9k are observed in poultry and mammal enterocytes, respectively. The present research showed that supplementation with 125–2000 IU/kg VD3 increased the mRNA abundance of CaBP-D28k in the three intestinal segments of broilers to varying degrees, especially in the jejunum. 1,25-(OH)2-D3, the final active form of VD3, increases mRNA abundance of intestinal CaBP-D28k in laying hens [28]. Thus, VD3 and 1,25-(OH)2-D3 stimulated CaBP-D28k gene transcription in the intestinal cells of poultry and contributed to the increase in leg bone Ca content. In addition, research in mammals has shown the positive effect of 1,25-(OH)2-D3 on the gene expression of intestinal CaBP-D9k [5].
PMCA1b is expressed in the basolateral membrane. The addition of 1,25-(OH)2-D3 enhances the mRNA abundance of duodenal PMCA1b in mice [29]. Similar results were noted in this research. The mRNA abundance of PMCA1b in the duodenum of broilers increased after adding 250–1,000 IU/kg VD3. Thus, optimal levels of VD3 upregulated PMCA1b gene expression and promoted Ca extrusion from intestinal cells into the blood. Notably, the addition of 2,000 IU/kg VD3 insignificantly affected intestinal PMCA1b mRNA level compared with the negative control diet.
Similar to PMCA1b, NCX1 also exists in the basolateral membrane. Dietary VD3 insufficiency leads to a decrease in the mRNA abundance of NCX1 in chick duodenum, which is increased after the injection of 1,25-(OH)2-D3 [30]. This research showed that adding 1000 IU/kg VD3 enhanced the mRNA abundance of duodenal and jejunal NCX1 by 0.67–1.74 folds. These data suggest that VD3 promoted the exchange of Ca and Na in intestinal cells and the blood.
NaPi-IIb, the major P transporter, is found in the apical membrane of rat intestinal cells [12,13]. This research showed that the mRNA abundance of NaPi-IIb in the three intestinal segments was increased by dietary VD3 to varying degrees. The effects of the VD3 on NaPi-IIb gene expression have been observed in broilers [31], in which a high VD3 dosage upregulates the mRNA abundance of intestinal NaPi-IIb compared with a low VD3 level. The addition of VD3 enhances the protein expression level of jejunal NaPi-IIb in broilers fed with P-insufficient feed [15]. Thus, VD3 stimulated NaPi-IIb gene expression and the active P absorption in broiler intestines.
PiT-1 is expressed in the apical membrane of rat enterocytes [13]. Compared with NaPi-IIb, PiT-1 plays a minor role in phosphate transport into the intestinal epithelial cells of mice [14]. Supplementation of 250–1,000 IU/kg VD3 increased the mRNA abundance of duodenal PiT-1 in this research. The addition of VD3 also enhanced the protein abundance of jejunal PiT-1 in broilers provided with P-inadequate diets [15]. These data reveal the positive effects of VD3 on intestinal PiT-1 gene transcription in broilers.
The gene expression of PiT-2 has been observed in the apical membrane of rodent intestinal cells [12,32]. PiT-2 involves P absorption in mouse intestines upon dietary P restriction [32]. This research showed that the addition of VD3 increased the mRNA abundances of duodenal and ileal PiT-2 in broilers. Thus, VD3 upregulated PiT-2 gene expression and promoted P transport in the apical membrane of broiler intestinal cells.
In conclusion, the highest mRNA abundances of CaBP-D28k, PMCA1b, NCX1, and NaPi-IIb were detected in the duodenum of broilers. On the contrary, the mRNA abundances of PiT-1 and PiT-2 in the duodenum were lower than those in the two other intestinal segments. Adding 125–2,000 IU/kg VD3 improved growth performance and leg bone quality of 1–21-day-old broilers. The mRNA level of CaBP-D28k increased by 536, 1,161, and 28 folds in the duodenum, jejunum, and ileum, respectively, after adding 1,000 IU/kg VD3. By contrast, the mRNA abundances of other Ca and P transporters (PMCA1b, NCX1, NaPi-IIb, PiT-1, and PiT-2) increased by 0.57–1.74 folds by supplementation with 1,000–2,000 IU/kg VD3.