INTRODUCTION
Nutrient digestion and absorption in the gastrointestinal tract are vital factors in the growth and metabolic functions of animals because only digested nutrients can be used as the backbone of fat and protein in livestock animals. Accordingly, the use of easily digested feed ingredients (soybean meal [SBM], dairy products, plasma protein, corn meal, etc.) could dramatically improve the productivity of livestock compared to high-nonstarch polysaccharide-containing feed ingredients (wheat, barley, cottonseed meal, etc.) [1,2]. As the price of easily digested feed ingredients gradually increases, the livestock industry has difficulty applying these materials in feed; nevertheless, developing new feed ingredients is difficult due to the limitation of the cultivation area and competition between humans and animals [3]. Therefore, recent studies have focused on improving the nutritional value of the feed itself by using additional feed supplements, including enzymes, probiotics, emulsifiers, etc. [4–6].
One of the methods to solve the grain-price problem is using ingredients that are less expensive but have the same or increased nutritional value [7,8]. A byproduct of the beverage industry with several different grains used in the fermentation process, dried distillers’ grains with solubles (DDGS), was introduced to the feed industry in the 1930s [9]. However, the inclusion of DDGS in broiler rations reduced digestibility and nutrient availability due to the high fiber content of DDGS [10]. Lio and Wang reported that supplementary enzymes in animal diets, such as xylanase or cellulase, could be used to overcome this problem [11]. Another experiment demonstrated that supplementary xylanase was proven to promote animal performance by improving digestibility [12]. Nian explained that chicks fed a diet including xylanase improved nutrient and energy utilization and reduced heat increment [13]. Because of the activity of xylanase, more dietary fiber in the diet was degraded, and hemicellulose digestibility was also increased.
There are various types of enzymes used as feed supplements in the livestock industry, which can be largely divided into a single enzyme (SE) and a multienzyme complex (MC) [14,15]. An example of a SE (β-mannanase) is a type of enzyme that targets hard-to-digest ingredients in feed, and an enzyme complex is intended to simultaneously increase the digestibility of various nutrients in feed by adding diverse enzymes at the same time [16,17]. However, since the effects of multienzyme complexes on livestock growth performance are still controversial, further comparison studies will be needed on the efficacies of SE and multienzyme complexes. Collectively, we conducted an experiment with broiler chickens to investigate the feed supplementation effect of a SE and multienzyme complex, and we also analyzed the feed dependence of dietary enzymes.
MATERIALS AND METHODS
Four hundred twenty male Ross-308® broiler chicks (1 day old) were individually weighed and allotted into a 4 experimental treatment groups with 7 replications, and 15 chicks were housed in each pen. The experimental treatments was as follow: CON, control group fed with corn-SBM diet; NC, group fed with DDGS supplemented diet; SE, group fed with 0.05% of mannanase supplemented DDGS-based diet; MC, group fed with 0.10% of multienzyme complex (mannanase and xylanase, glucanase) supplemented DDGS-based diet. The experiment was conducted for 35 days, feeding a starter diet for the first 7 days, a grower diet for the next 14 days, and a finisher diet for the last 14 days. Diets were formulated based on National Research Council in mash form to meet or exceed the nutrient requirements of broilers except for energy content. The starter diet included 5% DDGS, and the grower and finisher diets included 10% DDGS as commercially used in the Korean feed industry. The compositions of the experimental diets are given in Table 1. Body weight (BW) and feed intake were measured at the end of each phase after 8 hours of feed deprivation, and average daily gain (ADG), average daily feed intake (ADFI), and feed efficiency (FE) were calculated.
After 35 days of the growth experiment, 3 broilers from each pen were sacrificed, and cecum content and jejunum tissue were collected. Cecum content was used to determine the microbial population between Lactobacillus, coliforms, and Bacteroidetes by employing quantitative real-time polymerase chain reaction (qRT-PCR) with specific primers after genomic DNA extraction. Additionally, jejunum samples were used for morphological determination (villus height and crypt depth and mucus-secreting cells). mRNA was extracted from jejunum samples, and the expression levels of inflammatory cytokines were determined with real-time PCR.
Digestibility was determined after the end of the growth experiment [18,19]. Broilers were fed a diet containing Cr2O3 for two days, 3 birds were sacrificed in each pen, and digesta were collected. Total tract apparent digestibility was calculated by measuring the dry matter (DM) of consumed feed and excreta from each bird (replicate) and employed for determining DM, crude protein (CP), and gross energy (GE).
Jejunum samples were fixed in formalin solution and embedded into paraffin blocks. We prepared 5-μm cross sections using a Rotary Microtome CUT 5062 (SLEE MAINZ, Mainz, Germany), and these sections were stained with hematoxylin and eosin and Alcian blue staining methods. Fifteen villi and crypts were randomly selected and measured to calculate the ratio of villus height to crypt depth. A blind observer measured villus height and crypt depth and counted the goblet cell numbers.
TRIzol® (Invitrogen, Grand Island, NY, USA) was used to extract total RNA from jejunum samples according to the manufacturer’s procedure, and the RNA purity and concentration were measured using a Nanodrop spectrophotometer (Thermo Scientific, Wilmington, DE, USA). cDNA samples were synthesized with the High-Capacity cDNA Reverse Transcription kit (Applied Biosystems, Carlsbad, CA, USA). Gene expression levels related to the immune response in the jejunum (interleukin [IL]-2, IL-4, IL-6, tumor necrosis factor [TNF]-α; IFN-γ, transforming growth factor [TGF]-β) were determined using a RealHelixTM Premier qPCR kit (NanoHelix, Daejun, Korea) with a StepOnePlus Real-Time PCR System (Applied Biosystems, Carlsbad, CA, USA). Primers for the target genes are listed in Table 2. Glyceraldehyde 3-phosphate dehydrogenase (GAPDH) was used as a housekeeping gene, and the 2−ΔΔCt method was used to quantify relative mRNA expression levels.
A Quick-DNATM Fecal/Soil Microbe Microprep Kit (Zymo Research, CA, USA) was used to extract total genomic DNA from the cecal samples according to the manufacturer’s protocol. The concentration and purity of bacterial DNA were determined using a Nanodrop spectrophotometer (Thermo Scientific, Wilmington, DE, USA).
The population of cecal bacteria (Lactobacillus, coliforms, and Bacteroidetes) was determined using a RealHelixTM Premier qPCR kit (NanoHelix, Daejun, Korea) with a StepOnePlus Real-Time PCR System (Applied Biosystems, Carlsbad, CA, USA), and the expression level of total bacteria was used as a housekeeping gene. Primers for the target genes are listed in Table 2, and the 2−ΔΔCt method was used to quantify relative gut microbial population levels.
All data were analyzed using Analysis of variance (ANOVA) with Statistical System 9.4 (SAS Institute, Cary, NC, USA). The experimental unit of growth performance was a pen, and the experimental units of results related to digestibility, gut morphological indices, cecal microbial population, and jejunal inflammatory cytokines were a chick. Significant differences between treatments were determined using Duncan’s multiple-range tests and were defined at the p < 0.05 level.
RESULTS
Broiler chickens fed the control diet showed better growth performance than DDGS-supplemented diet-consuming chickens. On Day 35, the BWs of birds in the MC group was significantly heavier than the BWs in the NC group (Table 1; p < 0.05). In finisher phase, ADFI of MC group was significantly increased compared to CON and NC treatment groups, and the FE of DDGS-supplemented diet groups were significantly lower compared to CON group (p < 0.05). In the overall experimental period, MC supplementation significantly increased ADG compared to the CON group; however, ADFI also increased by SE and MC supplementation compared to CON group (p < 0.05). Collectively, dietary SE and MC could increase growth in DDGS-supplemented diet groups as much as that of CON group, and it might be due to the increment of ADFI by SE and MC supplementation (Table 3).
All kinds of nutrient digestibility (DM, CP, and GE) were significantly decreased by DDGS supplementation in the basal diet (Fig. 1). However, dietary SE and MC supplementation in the DDGS diet could restore DM, CP, and GE digestibility as much as those of the corn-SBM diet group.
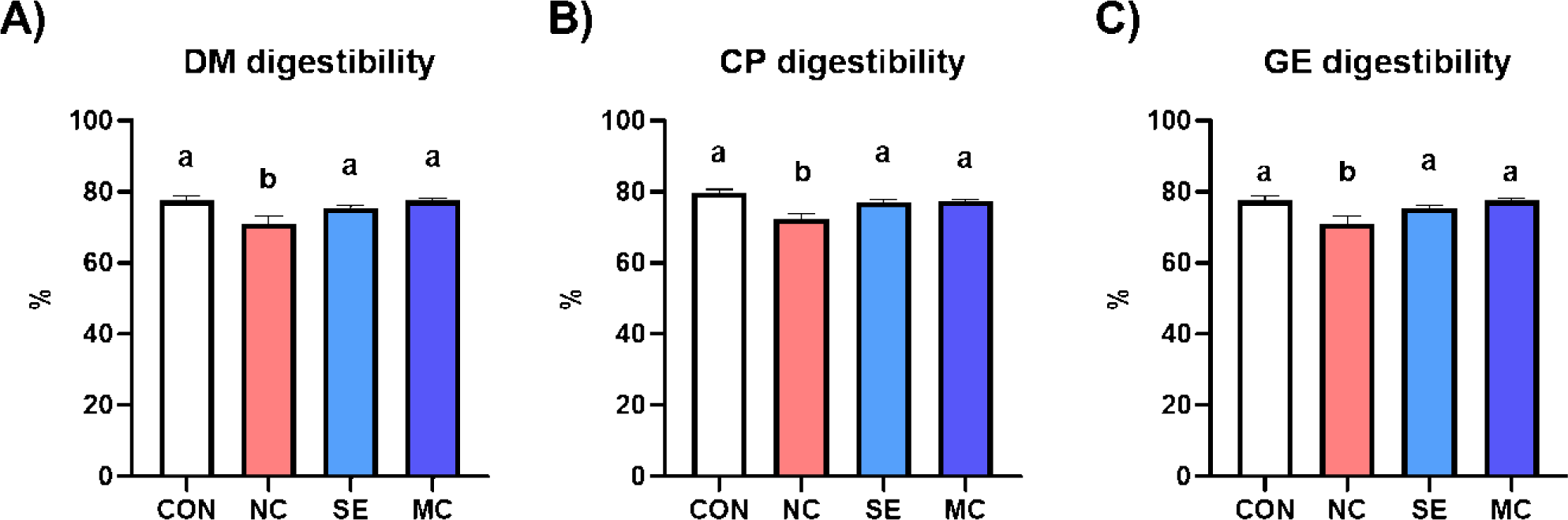
The cecal bacterial population is shown in Fig. 2. The population of Lactobacillus was significantly increased by dietary MC supplementation compared to the CON group (Fig. 2A; p < 0.05). Conversely, the population of coliforms was significantly decreased by SE and MC supplementation in the DDGS-supplemented diet groups compared to NC group (Fig. 2B; p < 0.05). Moreover, dietary addition of SE and MC significantly decreased the population of Bacteroidetes compared to the CON and NC groups (Fig. 2C; p < 0.05).
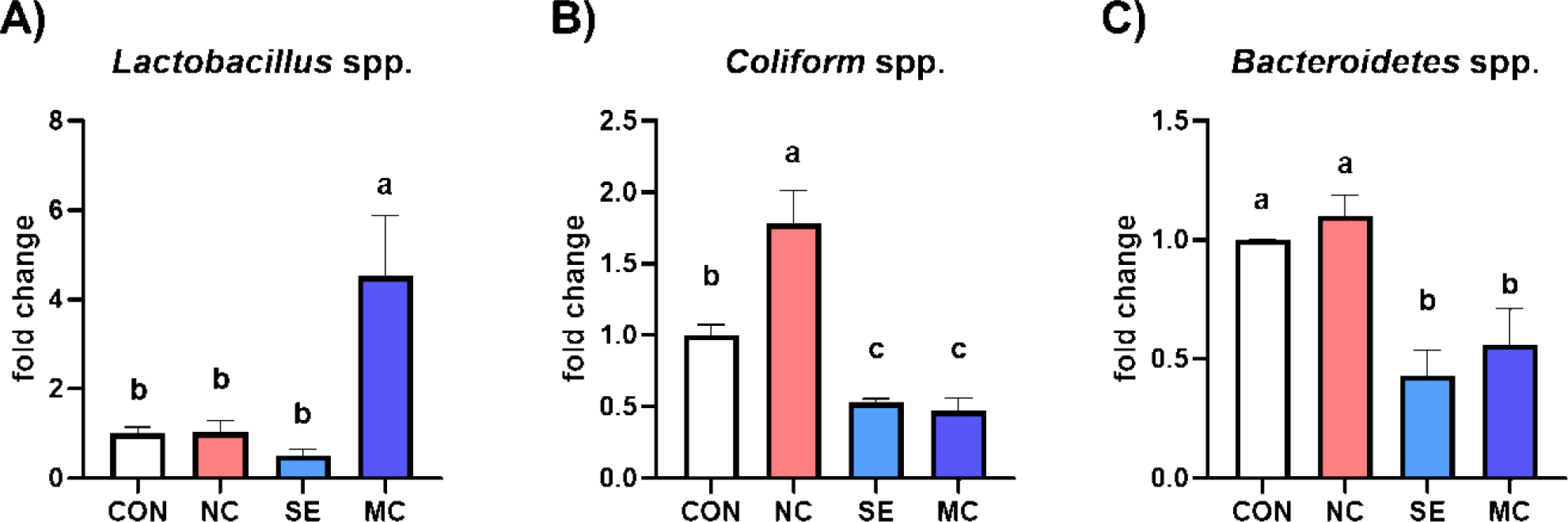
The jejunal morphological indices are presented in Fig. 3, and representative pictures of jejunum cross sections stained by the hematoxylin and eosin method and Alcian blue method are shown in Fig. 4. The villus height in the enzyme-supplemented groups were significantly higher than those of NC group without affecting the crypt depth (Figs. 3A and 3B; p < 0.05). The ratio of villus height to crypt depth was significantly decreased in the DDGS group among treatments; however, dietary SE and MC supplementation significantly increased as much as that of CON group (Fig. 3C; p < 0.05). DDGS supplementation decreased the goblet cell count; however, the dietary enzyme-supplemented groups showed restoration of goblet cells compared to the NC group (Fig. 3D; p < 0.05).
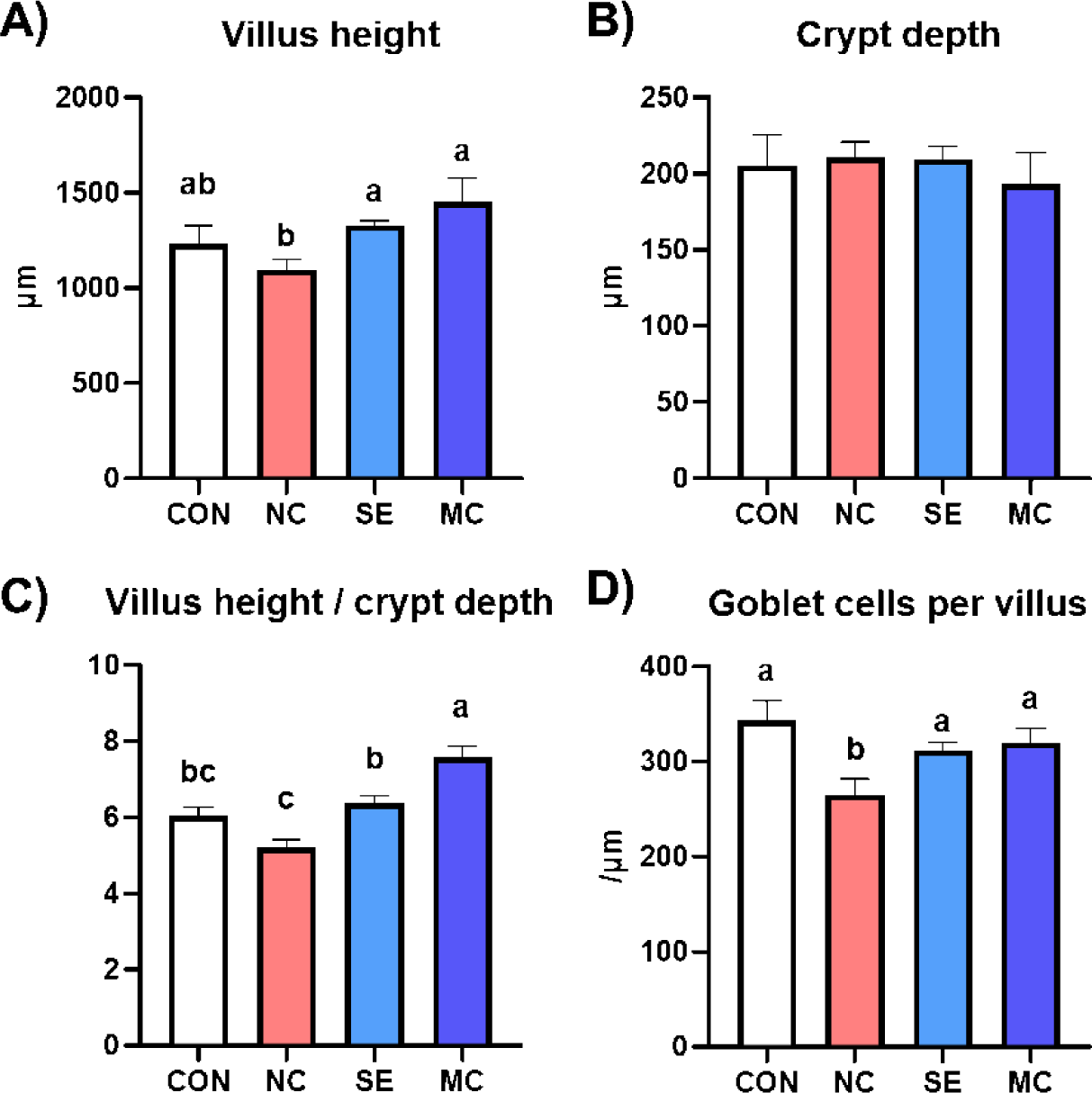
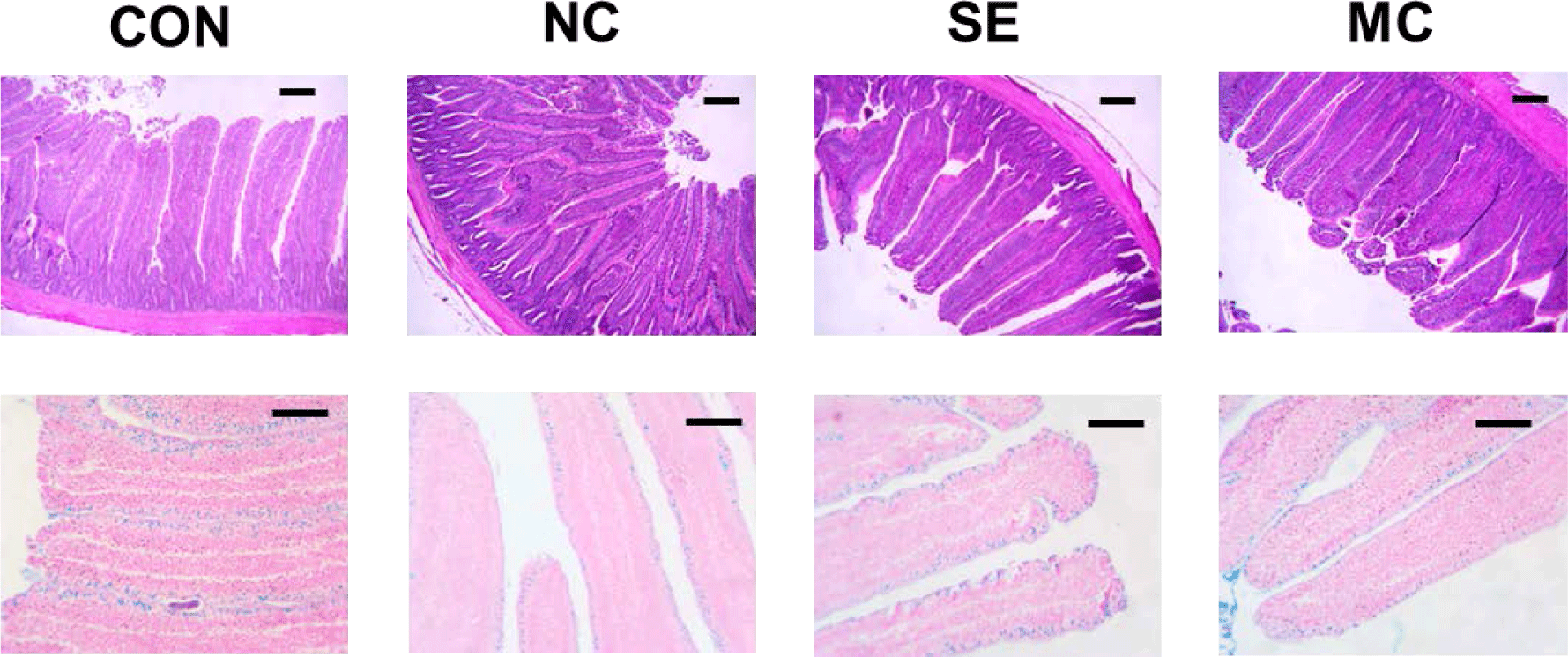
The gene expression levels of anti-inflammatory cytokines are presented in Fig. 5. Dietary SE supplementation in the DDGS diet significantly increased the expression level of IL-4 compared to NC and MC treatment groups, and dietary DDGS supplementation also significantly decreased the expression level of IL-6 compared to CON group (Figs. 5A and 5B; p < 0.05). Otherwise, dietary DDGS supplementation could increase the expression level of TGF-β, however the SE and MC supplementation could decrease the TGF-β level as much as that of CON group (Fig. 5C; p < 0.05).
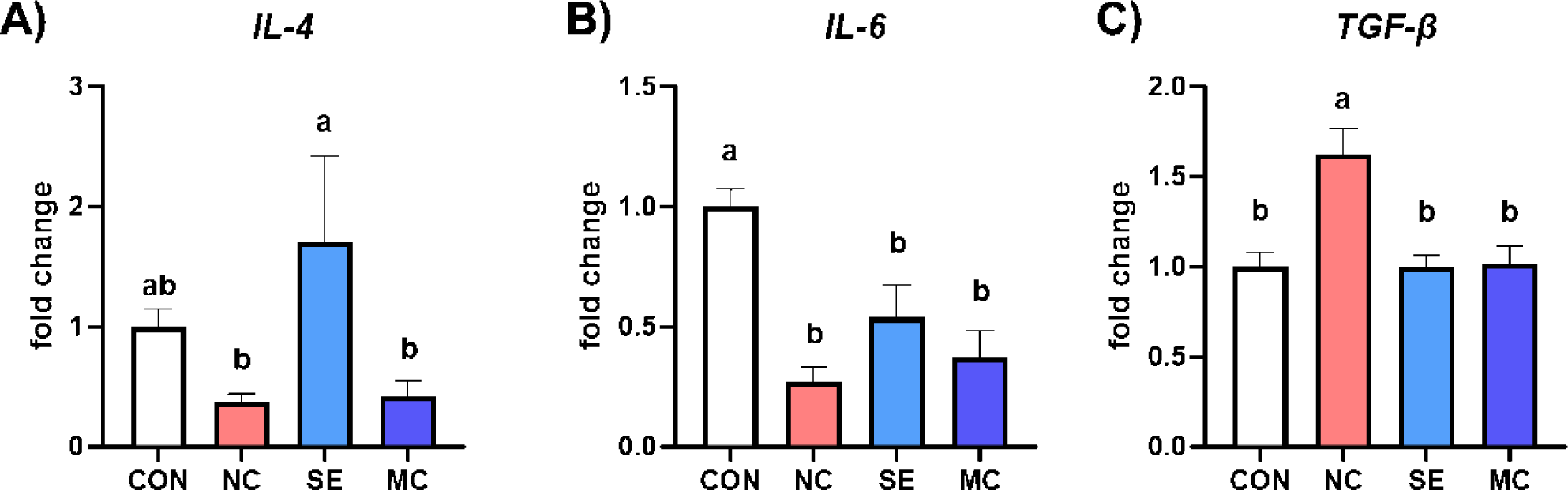
The mRNA expression levels of proinflammatory cytokines are shown in Fig. 6. The expression level of IL-2 was significantly lower in NC group compared to CON and SE groups (Fig. 6A; p < 0.05). However, dietary SE supplementation in DDGS-supplemented diet showed significantly lower expression level of TNF-α compared to the levels of NC and MC diet groups (Fig. 6B; p < 0.05). Furthermore, the expression level of IFN-γ was increased by DDGS supplementation; however, SE could reduce the IFN-γ expression level to as much as the level of the CON group (Fig. 6C; p < 0.05).
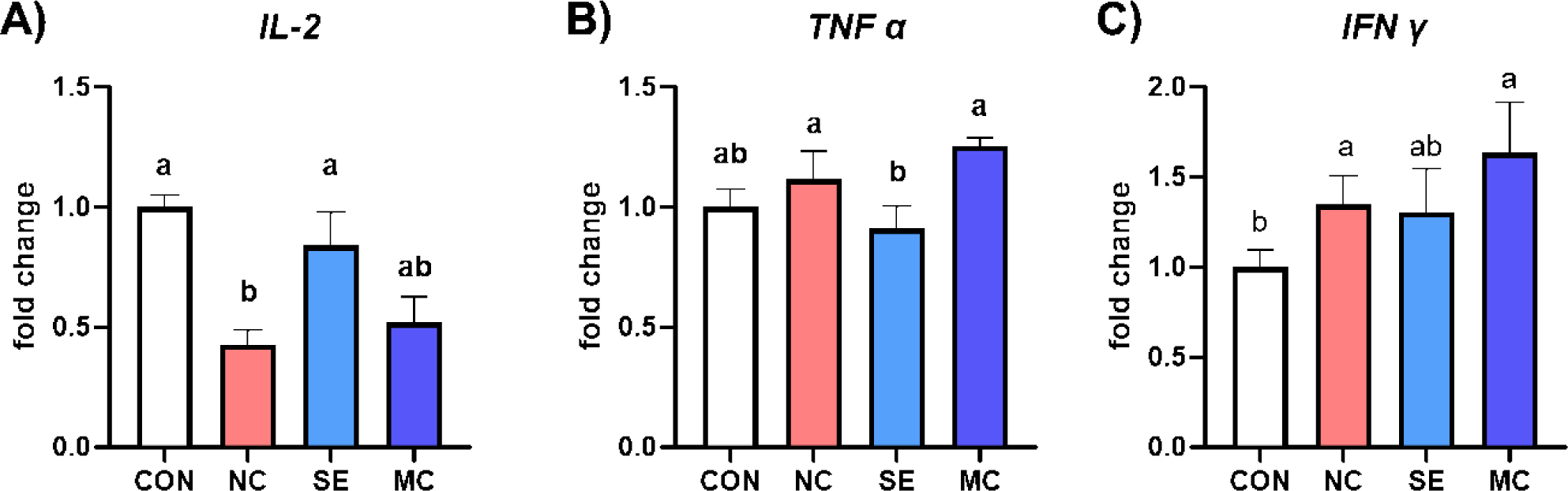
DISCUSSION
This study was conducted to investigate the efficacy of SE and MC when they are supplemented in two types of diets, corn-SBM diet and DDGS-supplemented diet, and the results of this study demonstrated that each enzyme additive improves the growth of broiler chickens via different mechanisms according to diet type. All kinds of enzymes in the DDGS-supplemented diet could increase nutrient digestibility, including DM, CP, and GE, and we can assume that dietary enzymes could improve nutrient bioavailability by modulating the gut microbiome population. In particular, dietary enzymes significantly reduce the population of Bacteroidetes, which is related to the host’s hepatic lipid metabolism. However, SE and MC1 supplementation in the corn-SBM diet showed improved growth performance, and MC2 supplementation in the DDGS diet also showed better growth performance compared to the DDGS group. In the corn-SBM diet groups, SE addition decreased proinflammatory cytokines by decreasing the pathogenic bacterial population. Coliforms and Bacteroidetes were found in the gut microbiome. Otherwise, MC1 supplementation in the corn-SBM diet increased the Lactobacillus population and could lead to improved gut morphological environments and accelerated growth. In the DDGS-supplemented diet groups, MC2 addition significantly upregulated growth performance, with an increased beneficial bacterial population and a reduced pathogenic bacterial population. Dietary MC2 in the DDGS diet also significantly improved gut microenvironments, including the local immune response and gut morphological indices.
Dietary exogenous enzyme addition in a DDGS-supplemented diet could increase the bird’s growth performance to as much as the growth performance of the control group. This result indicates that dietary supplementation with exogenous enzymes could increase the nutrient availability of feed itself by supplementing the fiber contents of DDGS. In 2021, Van Hoeck et al. demonstrated that dietary xylanase and wheat supplementation could increase the growth of birds [20]. In addition, Klarie also proposed that dietary β-mannanase supplementation could accelerate growth in diverse livestock animal models, including broiler chickens, laying hens, turkeys, sows, and nursery-finishing pigs [21]. Collectively, dietary enzymes with their proper high-NSP-containing feed ingredients could be advantageous in the livestock industry.
Exogenous enzyme supplementation in a DDGS-supplemented diet resulted in increased DM, CP, and GE digestibility compared to no enzyme-supplemented groups. In accordance with our study, Fries-Craft et al. also demonstrated that DDGS as a dietary ingredient could reduce nutrient digestibility [22]. However, the results of the study of Hussain et al. indicated that dietary exogenous enzyme supplementation could recover nutrient digestibility, including DM, CP, and energy, as much as that of the control group [23]. In particular, SE supplementation presented the highest GE digestibility among treatments, and this result agreed with the studies performed by Latham and White. They demonstrated that β-mannanase supplementation could increase energy utilization and digestibility in both laying hens and broiler chickens [24,25]. Additionally, Ko and colleagues also demonstrated that dietary enzyme addition could increase DM and GE digestibility [26]. Altogether, this study could propose that DDGS would decrease nutrient availability when it is used as a feed ingredient; however, dietary enzyme supplementation could restore these harmful effects.
Dietary inclusion of DDGS could increase the representative pathogenic bacteria and coliforms; however, cosupplementation of DDGS and xylanase could decrease the population of coliforms in broiler chickens [27]. Coliforms could have a negative effect on gut microbial ecology, and the decrease in the coliform population can be considered a more balanced intestinal microecosystem with the improvement of beneficial bacteria [28]. In this study, all kinds of exogenous enzymes could restrict the spread of pathogenic bacteria such as coliforms and Bacteroidetes. Additionally, this study proposed that MC2 supplementation could increase the population of beneficial bacteria, Lactobacillus, in both the corn-SBM diet and DDGS diet, and MC1 supplementation could upregulate the Lactobacillus population in only the corn-SBM diet. In accordance with this study, Mountzouris et al. also demonstrated that β-galactosidase supplementation could be attributed to the increased level of the Lactobacillus population [29]. In addition, gut microbial modulation by Lactobacillus has a positive relationship with gut immune homeostasis and defense systems [30]. These results proposed that dietary DDGS could increase pathogenic bacteria and decrease beneficial bacteria populations; however, proper enzyme inclusion could reverse the population of the pathogenic and beneficial bacteria.
Alizadeh et al. demonstrated that dietary DDGS supplementation could destroy villus morphology by upregulating immune responses [31]. However, Amer et al. also proposed that dietary enzyme supplementation could restore gut histology [32]. These results were consistent with our study; however, further studies will be needed to investigate the exact mode of action of dietary exogenous enzymes. However, the gut mucus layer is important because it is the first line of defense protecting the epithelium against luminal threats, including physical forces, pathogens, and the gut microbiome [33]. Consistent with the results of this study, Alizadeh et al. also demonstrated that dietary DDGS could demolish the mucus layer in the small intestine [31]. However, the inclusion of enzymes restored the number of goblet cells. Walk and colleagues also proposed that dietary enzyme supplementation could reconstruct the mucus layer by the reestablishment of goblet cells [34].
Dietary enzyme supplementation could improve gut immune status by strengthening the gut mucosal layer and regulating inflammatory cytokine secretion. Min et al. demonstrated that dietary DDGS supplementation could increase representative inflammatory cytokines such as IL-1β and IL-6 [35]. In accordance with this study, our study also demonstrated that the DDGS diet increased the populations of TGF-β, TNF-α, and IFN-γ; however, the expression levels of the interleukin family members IL-2, IL-4, and IL-6 were conversely decreased by DDGS supplementation. TGF-β is a major immune regulator in livestock animals, and dietary DDGS supplementation significantly increased the expression level of TGF-β [36]. However, dietary exogenous enzymes could decrease the level of TGF-β, and it might be a key mediator leading to improved gut environments and growth performance. Additionally, MC1 in the DDGS diet increased IL-4 and IL-6, and this result indicated that MC1 could regulate immune responses in the jejunum by modulating the interleukin family. In the corn-SBM diet groups, SE decreased all kinds of inflammatory cytokine secretion, and this result could suggest that SE in the corn-SBM diet is more suitable than MC for improving the immune status of broiler chickens.
CONCLUSION
Dietary DDGS supplementation decreased growth; however, exogenous enzymes in a DDGS-supplemented diet improved growth performance as much as the growth performance of birds fed the corn-soy diet. In particular, exogenous enzymes could improve nutrient digestibility by increasing villus height and the villus crypt ratio through the downregulation of the key immune factor TGFβ. Additionally, dietary SE and multienzyme complex supplementation showed lower expression levels of proinflammatory cytokines, which might be due to the improved gut defense system by increasing the number of mucin-secreting cells. Collectively, the effects of dietary SE and multienzyme complexes were similar, and further studies will be needed to investigate the selection of enzymes that directly target NSPs in feed ingredients.