INTRODUCTION
Weaning is the most stressful time in a pig’s life in modern swine farming and often causes diarrheic syndrome and growth depression [1-3]. At weaning, pigs are subjected to multiple stressors, including sudden dietary and environmental changes, that induce intestinal inflammation and unstable intestinal microbial communities [1,2,4]. The change from highly digestible milk to less digestible solid feed causes behavioral changes and intestinal infections, mostly because of coliform overgrowth [5,6], and is responsible for the 17% mortality rate of piglets in Europe [7]. The decreased feed intake and structural changes in the intestine influence the absorptive potential of nutrients through enterocytes, which may affect the growth rate [8,9]. Since the 2006 ban on antibiotics growth promoters by the European Union, research has focused on finding non-antibiotic supplements with antimicrobial potential to support gut health and weight gain of weaned pigs [10,11]. Among the alternatives, the pharmacological doses of ZnO (2,500 and 3,000 mg Zn/kg) have been extensively used in the diets of weaning pigs for therapeutic purposes [12-14]. However, environmental concerns have encouraged several countries, including Korea and those in the European Union [15], to legislate strict rules limiting the use of ZnO. The urgent challenge is to develop an alternative strategy to prevent post-weaning diarrhea because the supplementation with pharmacological ZnO will be phased out by June 2022.
The extent to which an element is absorbed during digestion determines bioavailability [16,17]. In recent years, the common form of ZnO has been used routinely in piglet diets; however, the low biological potential of ZnO increased its supplementation level to 25 to 30 times more than the growth requirement. Nano techniques have been extensively used in the food and pharmaceutical industry to produce nano-sized particles with greater biological activity than conventional sources owing to the increased surface area [18]. The nano mineral particle sizes are in the range of 1 to 100 nm; however, it is influenced by the molecular size and source of the minerals [18]. The decreased particle size of minerals correlates with their bioavailability [13,19,20]. Apart from the size of the nanoparticles, the nano process directly influences the biochemical properties of the elements. Hot-melt extrusion (HME) is among the most practical processes in the pharmaceutical field [18]. This method is based on mechanical shear and the polymer matrix to balance the mixing process at high temperatures to solubilize ingredients homogeneously [18,20,21]. The source and ratio of polymers determine the rate of aggregation and possibly the quality of the product. Our previous studies confirmed that the HME production process could be used as an environmentally friendly method in the animal feed industry to reduce trace mineral excretion [16,22]. Considering the importance of avoiding the pharmacological use of ZnO in the near future, the results of the current study may be a new hope to overcome this critical challenge. Therefore, the aim was to determine the effects of ZnO and nano-ZnO sources on body weight gain, nutrient digestibility, intestinal microbiota, gut integrity, and oxidative stress in weanling pigs.
MATERIALS AND METHODS
The protocol for this study was approved by Kangwon National University (Institute of Animal Care and Use Committee).
The experimental farm of Kangwon National University, located in Chuncheon, Korea, was used to conduct this trial (Approval No: KW-210503-6). The experimental diets were balanced to meet or exceed nutritional requirements (Table 1) of swine nutrient specification except for Zn [23]. Experiment 1: A total of 168 weanling pigs (Landrace × Yorkshire × Duroc, 21 ± 1 d old; body weight [BW] 6.81 ± 1.06 kg) were randomly distributed among the treatments based on a completely randomized design (CRD) according to initial BW. There were four treatments, six replicates, and seven pigs per replicate. The treatments were as follows: basal diet without Zn supplementation (control), 2,500 mg ZnO/kg (In2500), 500 mg nano-ZnO/kg (N500), and 150 mg nano-ZnO/kg (N150). The experiment was divided into two phases, from days 0 to 14 (phase 1) and days 15 to 28 (phase 2). Experiment 2: A total of 168 weaned piglets (Yorkshire × Landrace × Duroc, 21 ± 1 d old; BW, 6.59 ± 0.86 kg) were randomly divided between treatments based on a CRD design according to initial BW. There were three treatments, eight replicates, and seven pigs. The treatments were as follows: basal diet without ZnO supplementation (control), 2,500 mg ZnO/kg (In2500), 300 mg nano-ZnO/kg (N300), and 150 mg nano-ZnO/kg (N150). Experiment 2 was performed for 28 days and two phases (phase 1, days 0 to 14; and phase 2, days 15 to 28).
1) Supplied per kg of diet: 16,000 IU vitamin A (palmitate), 2.00 mg vitaminB1 (thiamin), 5.00 mg vitamin B2 (riboflavin), 2.00 mg vitamin B6 (pyridoxine), 0.03 mg vitamin B12 (cyanocobalamin), 25.00 mg niacin, 0.40 mg folic acid, 0.05 mg biotin, 5.00 mg ethoxyquin, 2,000 IU vitamin D3 (cholecalciferol), 75.00 mg vitamin E (dl-α-tocopheryl acetate), 2.00 mg vitamin K3 (menadione).
The HME technique was used to produce zinc oxide (TMC, Anyang, Korea). Zinc oxide, Span 80, Tween 80, and PEG 6000 were mixed at a 25:12:4:64 ratio. The processing procedure of the mixture (45 g/min) was performed using a hopper. The extruder had a twin screw (STS-25HS, Hankook E.M., Pyeongtaek, Korea) and a round-shaped die with a 1 mm diameter [18]. The temperatures of 55°C and 50°C were maintained in the barrel and die, respectively. The conveying and kneading were performed in the barrel at a speed of 0.025×g. The product was extruded through the die section and milled using an HBL-3500S grinder (Samyang Electronics, Gunpo, Korea) and transmission electron microscopy (TEM; JEM 1010, JEOL, Tokyo, Japan) was used to measure ZnO extrudate particle size. Powder of ZnO extrudate was dispersed in deionized water at 20 mg/mL and their particle properties were tested. The particle shape of ZnO extrudate was observed by TEM. The dispersed nanoparticles of ZnO were placed in the copper grid with film and dried for 10 min to be observed by TEM.
The BW gain was monitored during each phase by weighing on days 1, 14, and 28. At the end of each phase, the remaining feed in individual feeders was collected and weighed to determine the average daily gain (ADG), feed conversion ratio (FCR), and average daily feed intake (ADFI).
An in vitro microbial test was performed to evaluate the antimicrobial effects of ZnO (0, 300, 500, and 2,500 ppm) and nano-ZnO (300 and 500 ppm) mixed with nutrient broth, following the method of Joray et al. [24]. To test the antibacterial effects against Escherichia coli O157:H743895ATCC, Salmonella enteritidis ATCC 13076, and Listeria monocytogenes Scott A 19119 ATCC, 108 CFU/mL were mixed in the flasks. The inoculated broths were shaken at 120 rpm at 37°C for 24 h, and samples were taken at h 3, 6, 9, 12, and 24. The collected samples were serially diluted in sterile water and incubated on plate count agar. As per Hosseindoust et al. [12], fecal samples were collected on days 14 and 28 and sealed for microbiological analysis. The analysis was performed immediately after fecal collection. Lactobacillus spp. (de man, rogosa and sharp [MRS] agar + 0.200 g/L NaN3 + 0.500 g/L L-cystine hydrochloride monohydrate), coliforms (violet red bile agar), and Clostridium spp. (tryptose sulfite cycloserine agar, Oxoid, Hampshire, UK) were counted. For microbial groups, anaerobic conditions were generated using a Gaspak anaerobic system (BBL, No. 260678, Difco, Detroit, MI, USA). CFUs were used to determine microbial populations and transformed into log10.
At the end of Phase 1 and Phase 2, the nutrient digestibility test was performed to determine the apparent digestibility of dry matter (DM), gross energy (GE), and crude protein (CP). The two selected pigs were placed in individual cages (one pig per cage) on day 7 in phase 1 and on day 28 in phase 2 to collect fecal samples. A 2.5 g kg−1 chromium oxide (indigestible marker) was added to the diet of the selected pigs. Fecal samples were collected from days 12 to 14 in phase 1 and from days 26 to 28 in phase 2. The drying (60°C, 72 h) process was performed directly after sample collection in a forced air-drying oven and then powdered with a mill (Thomas Model 4 Wiley Mill, Thomas Scientific, Swedesboro, NJ, USA) using a 1 mm screen. Diet and feces analyses of DM and CP were conducted using the AOAC [25] method. A bomb calorimeter (Model 1261, Parr Instrument, Moline, IL, USA) and a spectrophotometer (Jasco V-650, Jasco, Tokyo, Japan) were used to determine the Cr2O3 concentration.
Six pigs close to the average weight were selected for each treatment for blood sampling via the jugular vein on days 14 and 28. The serum was harvested through centrifugation at 25,000×g for 15 min at 4°C. The collected serum samples were frozen immediately at −80°C for biochemical analyses. The antioxidant status of the serum and liver tissues was determined from the levels of superoxide dismutase (SOD), total antioxidant capacity (TAC), and glutathione peroxidase (GSH-Px) using an ELISA microplate reader and assay kits (Cayman Chemical, Ann Arbor, MI, USA). All assays were conducted according to the manufacturer’s instructions.
The data from the current experiment were statistically analyzed using SAS software (SAS Institute, Cary, NC, USA) and the general linear model (GLM) procedure. A CRD was used, and the pen was considered as the experimental unit for all parameters. Tukey’s multiple range test was used for means separation at p < 0.05.
RESULTS
The average particle size of nano-ZnO particles was 271.2 ± 39 nm compared with 711.1 ± 88 nm for ZnO. Experiment 1: The effects of dietary ZnO source and concentration are presented in Table 2. During phase 1, pigs in the N500 and IN2500 treatment groups showed higher ADG and ADFI than those in the control treatment group. There was no difference in FCR among treatment groups. Fecal score and diarrhea incidence were lower in the N500 and IN2500 treatment groups. In phase 2, there was no treatment effect on ADG, ADFI, FCR, and fecal score. However, there were significantly lower diarrhea incidences in the N500 and IN2500 treatment groups than in the control treatment. The overall result showed a greater ADG in pigs in the N500 and IN2500 treatment groups, and only the IN2500 treatment group showed a higher ADFI than the control treatment. The overall FCR was not affected by treatment. Experiment 2: During phase 1, pigs in the N300 treatment showed greater ADG and ADFI than those in the N150 treatment (Table 2). There was no difference in FCR among treatment groups. The fecal score was lower in the N300 and IN2500 treatment groups than in the N150 treatment group. In phase 2, there was no difference in ADG, ADFI, FCR, and fecal scores among the treatment groups. The overall results showed no difference in ADG, ADFI, and FCR.
The in vitro effects of nano-sized ZnO and ZnO on targeted pathogens after 24-h exposure are presented in Fig. 1. Listeria monocytogenes growth was inhibited when exposed to 300 and 500 ppm of ZnO from h 3 to h 24 of incubation. Moreover, the population of Listeria monocytogenes in the N500 and N300 treatment groups decreased linearly from h 0 to h 24. The same trend was observed after incubation with ZnO and E. coli. There was a significant increase in the number of E. coli in the N500 and N300 treatment groups throughout the 24-h experiment. The population of Salmonella typhimurium varied slightly among the treatment groups, and significant differences were observed at h 9 with a lower population in the N500 treatment group and the lower populations in the N500 and N300 treatment groups at h 12 and 24.
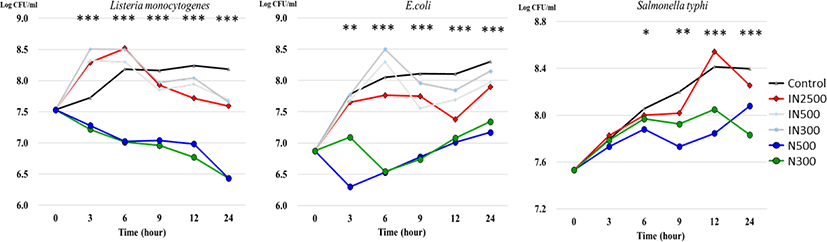
Experiment 1: The effects of ZnO concentration and source on intestinal microbiota are presented in Table 3. In phase 1, there was no difference in the number of Lactobacillus spp. However, the colonization of coliforms (p < 0.05) and Clostridium spp. (p < 0.01) significantly decreased in pigs fed the N500 and IN2500 diets compared with those fed the control diet. During phase 2, the number of Lactobacillus spp. and coliforms did not change among the treatment groups. The IN2500 pigs showed lower (p < 0.05) counts of Clostridium spp. than the N150 and control treatment groups. Moreover, there were lower (p < 0.05) populations of Clostridium spp. in the N500 and N150 treatment groups than in the control treatment group. Experiment 2: In phase 1, there was no difference in the number of Lactobacillus spp. However, the colonization of coliforms (p < 0.05) and Clostridium spp. (p < 0.05) decreased in pigs fed the N300 diet compared with those fed the N150 diet (Table 3). During phase 2, the treatments did not affect the number of Lactobacillus spp. and coliforms. The N300 pigs showed lower (p < 0.05) counts of Clostridium spp. than the N150 pigs. There was no difference in the colonization of Clostridium spp. between the IN2500 and N150 treatment groups.
The effects of dietary ZnO source and concentration on antioxidant capacity are shown in Fig. 2. In phases 1 and 2, the total plasma antioxidant capacity was greater in the IN2500 and N500 treatments than in the control (p < 0.05). In phase 1, the superoxide dismutase activity was greater in pigs fed the IN2500 diet than those fed the control diet. In phase 2, the IN2500 and N500 treatment groups showed a greater SOD activity than the control and N150 treatments. There was no difference in the activity of GSH-Px in either phase.
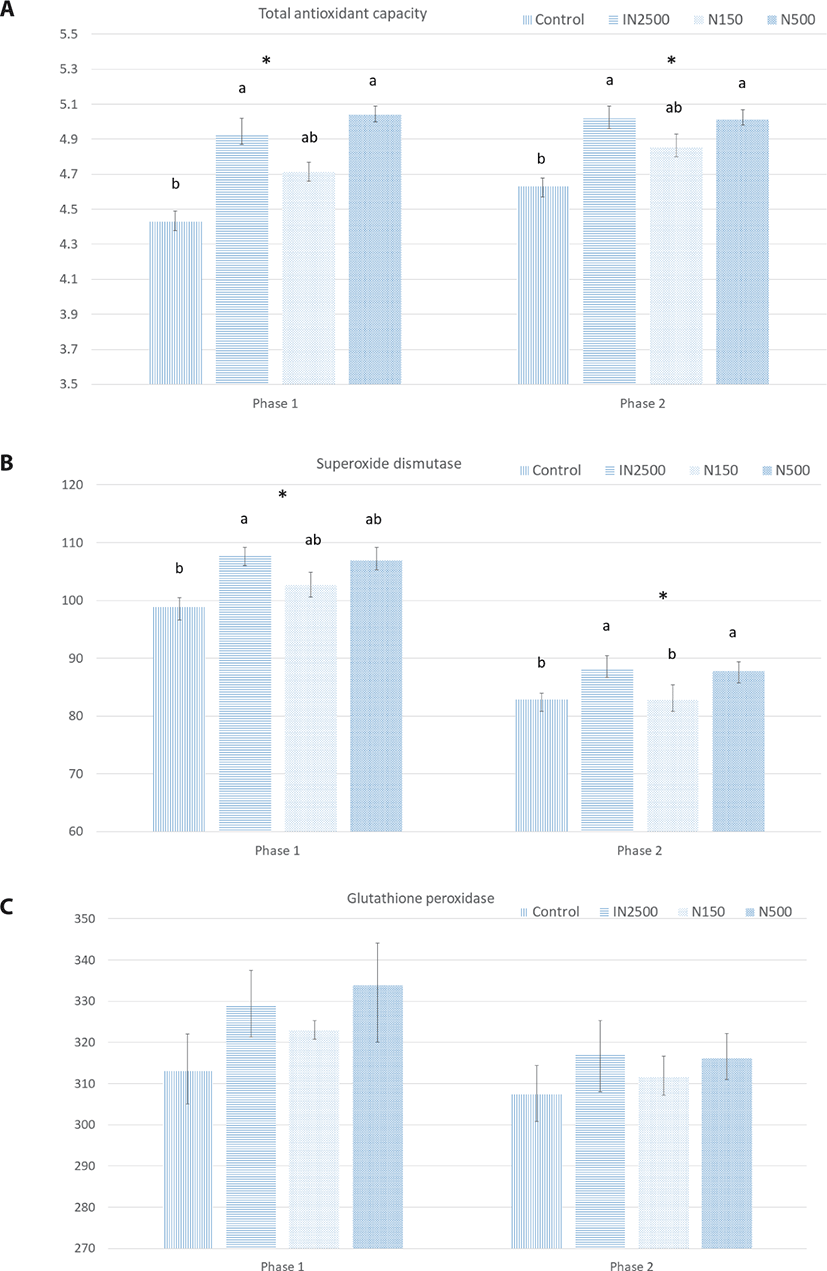
Experiment 1: The effects of ZnO on nutrient digestibility are presented in Table 4. There was no difference in the digestibility of DM, CP, ether extract (EE), and GE in phase 1. In phase 2, there was no difference in digestibility of DM, EE, and GE. However, the digestibility of CP was greater (p < 0.05) in pigs fed the IN2500 and N500 diets compared with the control treatment. Experiment 2: There was no difference in the digestibility of DM, CP, EE, and GE in phase 1 (Table 4). In phase 2, there was no difference in the digestibility of DM, EE, and GE.
DISCUSSION
In the current study, the improvement of ADG in the first phase emphasizes the benefits of ZnO supplements during the early weaning compared to supplementation from 2 weeks onward. The first week after weaning is critical in the pig’s life due to the change of diet from milk to solid form [11,14]. Additionally, the insignificant difference between the In2500 treatment and N300 or N500 treatment groups shows that a low level of nano-ZnO is a better option than a high level of regular ZnO. The positive influence of ZnO on ADG in this study is in agreement with previous reports where supplementation with ZnO as regular [4,9,11,26] or nano [13,19] forms increased ADG in pigs. The reduction in the number of pathogenic bacteria, including E. coli and Clostridium spp., may be associated with an increase in growth rate. It has been confirmed that supplementing with 2,500 ppm ZnO improved the growth rate of weanling pigs [11,27,28], whereas a lower dietary supplementation of ZnO has not been beneficial [29]. Several studies have reported using pharmacological ZnO [2,12,30] or nano-ZnO [8], without any significant effect on the growth performance of weaned pigs. Apart from dietary ZnO levels, particle size can be a determinant factor in the effectiveness of pathogenic control. The use of a proper nano method may govern the effectiveness of ZnO nanoparticles, as the aggregation of nanoparticles decreases the efficiency of the digestion process. Furthermore, the improved antioxidant status could also explain the increased overall ADG. Zinc is an important anti-stress mineral, and its antioxidant role in improving growth performance can be explained by its contribution to the production of antioxidant enzymes, including GSH-Px and SOD [4,20,31]. Weaning stress increases lipid peroxidation and consequently decreases glutathione peroxidase activity [15,31]. Therefore, the antioxidant role of Zn increases its requirement after weaning, compared to the other stages.
Using non-antibiotic supplements to decrease intestinal pathogen colonization is an effective approach to increase gut health and growth performance of weaned pigs. Among the options, ZnO is still one of the most important [11,14]. Recently, nanoparticles have received increasing attention because of their ability to be used at lower doses [19]. However, previous studies have shown that a 2,500 mg ZnO/kg feed has to be added to the weaned pigs’ diet to reduce diarrhea incidences [12,15], and lower doses of nano-ZnO are not adequate to control diarrhea [30]. This is in contrast with our results. The health of pigs during the transition period (i.e., from milk to a solid diet) can be determined by the frequency of diarrhea incidences [6,8]. The inability of the intestine to absorb essential nutrients increases the opportunity for pathogen growth, which may be the main cause of diarrhea in weaned pigs [8,13,14]. The reduction of diarrhea incidences and fecal scores in the In2500 and N500 treatment groups confirmed the positive effects of high ZnO supplementation in the diet to maintain gastrointestinal tract health in weaned pigs [8,12,19]. In the current study, the decrease in E. coli and Clostridium spp. suggests that the antibacterial role of ZnO protects the intestine from pathogenic damage, which is indicated by the decreased fecal scores and diarrhea incidences. E. coli is one of the most common bacteria that triggers diarrhea incidences in piglets during the post-weaning period [13,19,32]. Additionally, Clostridium spp. is opportunistic bacteria that spread via the fecal-oral route and produces several cytotoxins, which increase inflammation and diarrhea by binding to epithelial cell receptors [33,34]. These toxins damage the tight junctions, allowing the toxins to leak into enterocytes [34], and could be responsible for diarrhea incidences in weaned piglets. Supplementation of ZnO at a dose of 2,500 mg/kg diet or nano-ZnO at a dose of 300 g/kg diet was beneficial in controlling post-weaning diarrhea and E. coli colonization.
The improvement in the apparent total tract digestibility (ATTD) of CP was in line with the study of Oh et al. [19] and Lee et al. [11], who reported increased CP digestibility in weaned pigs fed a pharmacological dose of ZnO. The increased CP digestibility in the IN2500 and N500 treatment groups might be associated with the manipulation of gut microflora, which resulted in lower colonization by Clostridium spp. and E. coli. Previous studies reported that increased villus height in the intestine [19] and decreased E. coli populations were responsible for improving CP digestibility in weaned pigs fed ZnO. Suppression of pathogenic intestinal microbiota and increased antioxidant levels may further improve gut integrity to utilize more nutrients.
Several environmental and physiological factors affect intestinal development in neonates. In a healthy system, digestive enzymes hydrolyze the nutrients along the small intestine. The undigested nutrients flow to the large intestine and stimulate fermentation by anaerobic bacteria [5]. The current study showed that the population of gut-friendly bacteria such as Lactobacillus spp. was unaffected by the treatments. Simultaneously, the colonization of coliforms and Clostridium spp. were reduced in the In2500, N500, and N300 groups. Previous studies reported that a high dose of ZnO in weaned pig diets reduced the population of Clostridium spp. and coliforms [11,19] and increased the population of Lactobacillus spp. [11]. They stated that the increase in the Lactobacillus spp. population is likely due to the decreased colonization of E. coli and Clostridium spp. and the increased opportunity to proliferate in a less competitive environment [11]. Supplementation with ZnO increases hydrogen peroxide production and subsequently decreases the pH of the intestinal lumen [35]. The proliferation of E. coli and Clostridiumspp.can be controlled by an acidic environment, whileLactobacillus spp. can tolerate an acidic milieu [36]. In contrast, several studies have shown no anti-pathogenic effects when using pharmacological doses of ZnO [12]. The environmental and physiological conditions of weaned pigs may affect the efficiency of dietary ZnO. Moreover, using 50, 150, 250, and 1,000 mg ZnO/kg in weaned pigs did not affect the number of clostridial clusters, while supplementation with 2,500 mg ZnO/kg significantly reduced theClostridium spp. population [19,37]. Therefore, doses lower than 2,500 mg ZnO/kg cannot be recommended unless nano-ZnO is used because the decreased particle size increases the antibacterial efficiency of ZnO [19,38]. The nano-sized particles have a higher surface area, which increases the active surface compared to the regular particles [27,30,39]. Therefore, it can be presumed that nano-ZnO at lower doses is as effective as pharmacological doses of ZnO in preventing pathogen colonization.
The decrease in the production of antioxidant enzymes during weaning stress increases intestinal oxidative injury [4,26]. The enzymes, including SOD and GSH-Px, are essential for alleviating the overproduction of free radicals during stress [39,40]. It has been shown that supplementation with 3,000 mg ZnO/kg restored the plasma antioxidant capacity of weanling pigs by increasing SOD and GSH-Px activities and reducing Malondialdehyde (MDA) [26]; however, they reported no change in GSH-Px activity. Although the increased activity of SOD in the plasma was in line with the TAC, the GSH-Px activity was not affected in the current study. Zinc contributes to the production of several antioxidant enzymes and the regulation of the redox status by reducing the ratio of oxidized glutathione to reduced glutathione, which reduces apoptosis in the small intestine [41]. SOD plays an important role in protecting cells from exposure to reactive oxygen species during stress [4]. In this study, increased TAC in the plasma of pigs treated with In2500 and N500 may be related to the higher activity of SOD that increases the potential of scavenging free radicals, lipid peroxides, and hydroperoxide control [39]. This preserves the antioxidant factors and increases the TAC. Several studies have reported that SOD production can be compromised under stressful conditions [4]. These results suggest that dietary supplementation of nano-ZnO at 500 ppm can be as beneficial to the antioxidant status of weanling piglets as supplementation with 3,000 ppm ZnO.
CONCLUSION
Compared with the control treatment, the N500 diet increased growth performance. Additionally, it decreased the fecal scores and coliform and Clostridium spp. populations in weanling pigs. The N300 diet showed a growth performance comparable to the In2500 treatment, while the N150 treatment showed a compromised growth performance and intestinal microbiota. The present data suggest that using nano-ZnO in doses ranging from 300 to 500 ppm can be beneficial.