INTRODUCTION
Proteins with plant and animal origins have been marketed and consumed as important protein sources for animal feed formulations, and also possess a unique ability to supply amino acids to livestock [1,2]. In terms of poultry diets, soybean meal is a major source of protein. However, soybean meal contains anti-nutritional factors like trypsin inhibitors, which lower nutrient availability and limit the amount of soybean meal that can be incorporated into animal diets. Proteins comprise an essential component of diets, and furthermore, proteins are implicitly required for the survival of the animals and humans that consume them. Proteins’ nutritional function is to supply appropriate levels of essential amino acids in animal diets. The nutritional consistency of a meal depends upon the meal in question’s ingredients, its digestion, absorption levels, and the utilization of amino acids within the meal consumed.
Major nutrients, like carbohydrates, fatty acids, and proteins, are conveyed to the small intestine via the transport system expressed within enterocytes. Proteins are deconstructed into smaller peptides or amino acids and then transported into the small intestine. Tiny peptides are transported to the small intestine via peptide transporter 1 (PEPT1), which is located within the cell membrane [3]. Amino acids are transported to the small intestine via various transporters like basic amino acid transporter (rBAT), sodium-dependent neutral amino acid transporter (b0,+AT1), cationic amino acid transporter 1 (CAT1), CAT2, Y + L amino acid transporter 1 (y+LAT1), y+LAT2, neutral amino acid transporter (B0AT1), and acidic amino acid transporter (ASC1) [4,5]. Additionally, myoblasts carry out a critical role in skeletal muscle formation in regards to broiler growth. Myoblast differentiation is a complex process presided over by myogenic regulatory factors (MRFs). These factors consist of basic helix-loop-helix (bHLH) proteins, like myoblast determination protein (MyoD), myogenin (MYOG), MRF4, and myogenic factor 5 (Myf5), which act in the manner of transcription activators due to their DNA-binding activity on muscle-specific genes. Over the course of differentiation, MRFs can bind to the promoter region of muscle-specific genes, and then subsequently induce the gene’s mRNA expression, which is employed as a substrate for purposes of protein synthesis. MyoD and MYOG are crucial in the regulation of the proliferation and differentiation of muscle satellite cells [6]. In the past, in vivo studies have demonstrated that the expression of a varied gene can convert the various nutritional regimes employed in the feeding of broilers, including MyoD and MYOG, that affect satellite cell proliferation and differentiation [7–9]. However, the variations within the expression of myogenic-specific genes mirroring satellite cell activity that influence the accretion of muscle mass in the broiler chicken following post-hatch muscle growth is predominantly mediated by satellite cells. Powell et al. and Harthan et al. have previously reported that sensitivity satellite cells possess to methionylcysteine (Met/Cys) amino acids, and also the significance of optimal nutrition during the course of proliferation and differentiation in order to maximize satellite cells’ activity within the biological process, both affect successive muscle mass accretion [10,11].
Typically, appropriate levels of protease are released from within the gastrointestinal tract in order to optimize the usage of feed protein among chickens [12,13]. However, a concrete body of reports have shown that large amounts of proteins and amino acids travel through the gastrointestinal tract without being thoroughly digested [14–16]. Therefore, exogenous protease has been added into broiler diets in order to aid the digestion of proteins into smaller peptides and amino acids [17–19].
Comprehending the details of the role of protease within expression levels of growth-related genes and amino acids transporters is crucial to understanding animal nutrition, including poultry. However, to our prior knowledge, the role of protease within the expression of growth-related genes, amino acid transporters, and performance parameters within broilers have not yet been thoroughly investigated. The aim of our team’s study was to investigate the broilers’ growth performance and nutrient digestibility by mixing in protease with a corn-soybean-based diet. In addition, we analyzed the effects of a protease supplemented diet on the growth-, muscle-, and amino acid transport-related genes within broilers.
MATERIALS AND METHODS
Our experimental procedure was approved by the Animal Protocol Review Committee of Dankook University (Approval Nubmer: DK-1-1527).
Powdered protease (activity, 10,290 U/g) was generously provided by a commercial company (Advanced Enzymes, Maharashtra, India). In the first step, 600 healthy 1 d-old male Ross 308 broiler chicks with an average body weight (BW) of 46.7 ± 0.31 g were randomly assigned to four dietary treatments. Each treatment was comprised of 10 replicates, with 15 birds per replicates, in order to measure their growth performance and nutrient digestion ability for a 35 day long trial duration. The following corn-soybean meal based dietary treatments were utilized: 1) CON, basal diet; 2) T1, basal diet + 0.03% protease; 3) T2, basal diet + 0.06% protease; and 4) T3, basal diet + 0.09% protease. All broiler chicks were kept in stainless steel pens (1.75 × 1.55 m). All of the broiler chick diets were formulated following the nutritional requirements of broilers outlined by the Nutrient Requirements of Poultry [20] (Table 1). The experimental diets were administered in two phases (phase I from days 0–18 and phase II from days 19–35). Chicks were raised in a room heated to 33 ± 1°C for the first 3 days, and the temperature was lowered gradually until the room reached 24°C, maintaining humidity around 60% for the remaining duration the experiment. The experimental diets and broiler drinking water were supplied ad libitum. Based on the outcomes of these experimental treatments, broiler chick tissue was harvested from the CON and T3 groups for the purpose of gene expression analysis following the feeding trials.
The BW and feed intake (FI) of the broiler chicks studied were recorded at days 1, 18, and 35 after post-hatching on a cage basis in order to analyze the body weight gain (BWG) of the broiler chicks studied. The feed conversion ratio (FCR) was reached based on FI divided by the BWG.
At the conclusion of the experiment, the nutrient digestibility of dry matter (DM), crude protein (CP), energy, Ca, and P were all determined utilizing chromic oxide as an indicator [21]. 7 days before, 0.2% Cr2O3 was added to all broiler chick diets and fecal matter was harvested during the fourth week. All excreta were pooled by cage and combined. The fecal samples were subsequently dried using an electric oven heated to 50°C for a period of 72 hrs. Following the oven drying, fecal samples were ground into a fine powder in order to pass through a 1 mm sieve and then kept in a freezer cooled to −20°C until further analysis was conducted. After the collection of fecal matter, the broiler chick DM, CP, energy, Ca, P, and amino acids of all feed and excreta samples were analyzed. The chromium in the samples was investigated under UV absorption spectrophotometry (UV-1201, Shimadzu, Kyoto, Japan).
Following the final phases of experiment, the breast muscle, liver, and small intestine tissue of the broiler chicks were all isolated and total RNA was extracted using a TRIzol reagent (Invitrogen, Carlsbad, CA, USA). cDNAs synthesis from total RNA (1 µg) was conducted with the Maxima First Strand cDNA Synthesis Kit (Life Technologies, Carlsbad, CA, USA). The primer sequence was created according to the Primer3 program (http://frodo.wi.mit.edu/) (Table 2). The condition of quantitative real-time polymerase chain reactions was conducted according to the standard operating protocol and carried out with a 7500 Fast Real-Time PCR System (Applied Biosystems, Foster City, CA, USA). In the procedure, the system was set at 94°C for 30 seconds for the purposes of denaturation, set again at 59°C–61°C for 30 seconds for the annealing process, and then set at 72°C for 30 seconds for extension. A single peak melting curve from the amplicon was taken for expression analysis and glyceraldehyde-3-phosphate dehydrogenase (GAPDH) was utilized as an endogenous control for normalization purposes. The quantitative expression value was calculated based upon the 2ΔΔCt method [22].
All data were analyzed as a complete, randomized block design and ANOVA (ANOVA) was carried out following GLM procedures of SAS (SAS Institute, Cary, NC, USA). Our team employed a completely randomized design. Data were presented as the mean and standard errors of means. In addition, linear and quadratic polynomial contrast were performed on the effects of growing protease levels on growth performance and nutrient digestibility in broiler chicks. When variations existed between the dietary protease levels, multiple comparisons were conducted using a Tukey’s post hoc t-test for the purpose of statistical analyses (p < 0.05). A Student’s t-test was employed to determine the statistical differences in quantitative reverse transcriptase polymerase chain reaction (qRT-PCR) between the treatment and the control groups in RNA analysis. Results were considered significant at *p < 0.05 and **p < 0.01.
RESULTS
The effect of protease on growth performance is displayed in Table 3. Protease supplementation in poultry diets significantly increased the BWG of broilers over days 1–18 and 19–35, and also during the broiler chicks’ overall growth period (d 1–35), as the dietary protease levels increased from 0% to 0.09% (linear; p < 0.05). Additionally, protease supplementation led to significantly lower FCR than compared to the FCR of the CON group, with no supplementation over days 19–35, and also overall (d 1–35; linear; p < 0.05). No difference in FI was observed between the various treatment effects.
The effects of protease upon nutrient digestibility are summarized in Table 4. CP, DM, and energy digestibility were significantly increased in broiler chickens supplied with diets supplemented with protease levels of 0% to 0.09% (linear; p < 0.05). In contrast, the digestibility of calcium or phosphorus was not significantly affected by protease supplementation (p > 0.05). Additionally, the digestibility of a variety of amino acids, like lysine, methionine, cysteine, threonine, isoleucine, leucine, histidine, and tryptophan, displayed a significant increase with rising dietary protease supplementation in broiler chicken diets (linear; p < 0.05).
To determine the effect of 0.09% protease on the expression of growth-related genes, the expression of insulin-like growth factor 1 (IGF1), IGF2, GH (growth hormone), and leptin (LEP), were all examined within the sampled broiler liver. The expression of IGF1 (p < 0.05), IGF2 (p < 0.01), GH (p < 0.05), and LEP (p < 0.05) were significantly increased via dietary protease supplementation (Fig. 1).
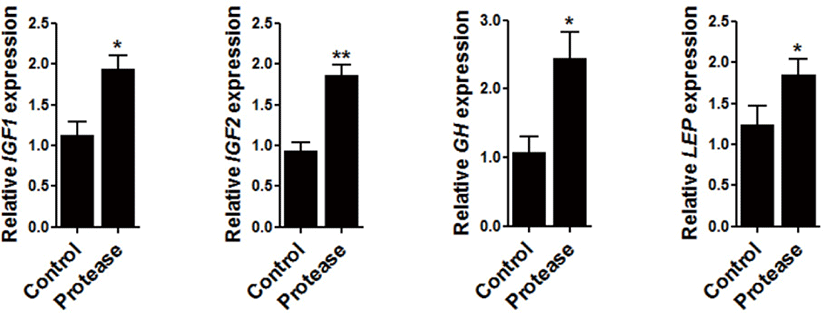
To determine the regulation of 0.09% protease in the expression of muscle development related genes, the expression of myostatin (MSTN), myogenic factor 4 (MYF4), MYOG, and myogenic differentiation 1 (MYOD1) were all examined in the muscle tissue of the broiler chickens. The expression of MYOD1 (p < 0.01) and MYOG (p < 0.01) were significantly increased via dietary protease supplementation (Fig. 2).
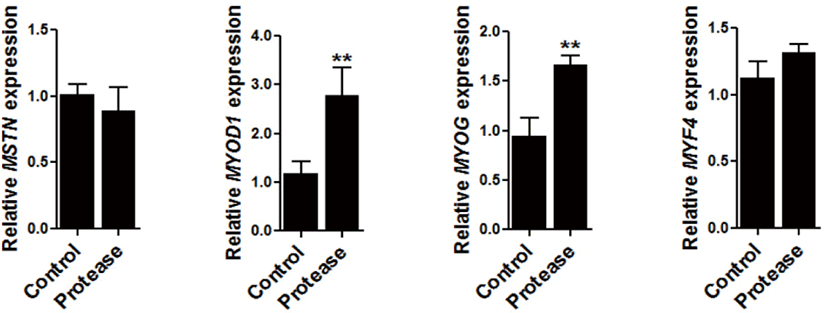
To investigate the effect of 0.09% protease on the expression of genes related to the amino acid transport system within broilers, the expression of amino acids transporter genes was examined in the small intestine of the sampled livestock. It was found that protease supplementation elevated the expression of SLCA19 (B0AT1) in the duodenum (p < 0.05), the jejunum (p < 0.05), and the ileum (p < 0.01; Fig. 3). Furthermore, protease supplementation heightened the expression of SLC7A1 (CAT1), SLC7A6 (y+LAT1), and SLC7A9 (b0,+AT) in the duodenum (p < 0.05) and within the jejunum (p < 0.05). Furthermore, protease supplementation increased the expression of SLC7A7 (y+LAT2) in the duodenum (p < 0.05), the jejunum (p < 0.01), and the ileum (p < 0.05), alongside raising the expression of SLC7A2 (CAT2) in the duodenum (p < 0.05), the jejunum (p < 0.05), and the ileum (p < 0.05), and finally, the expression of SLC15A1 (PEPT1) in the jejunum (p < 0.05) and the ileum (p < 0.05).
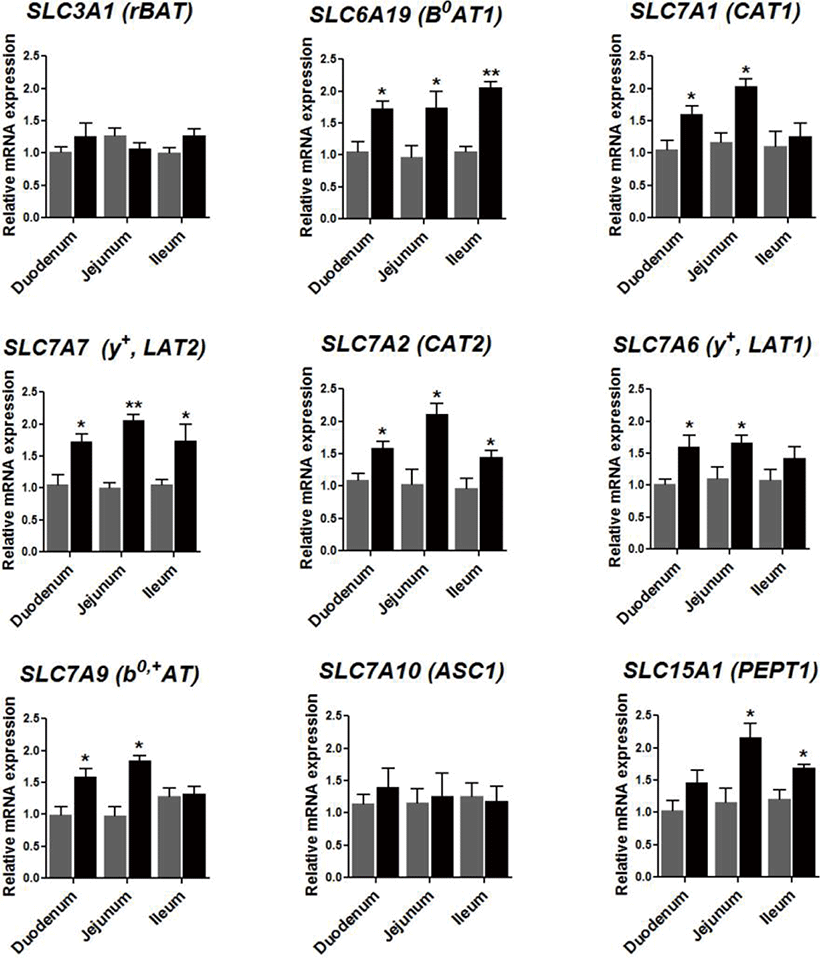
DISCUSSION
Our study demonstrated that dietary protease supplementation increased and decreased the BWG and FCR, respectively, in broiler chickens. Our findings complement the existing data from previous studies, which display increases in the growth performance of pigs [23–27] and chickens [28,29] following dietary protease supplementation in the aforementioned livestock.
Proteases play a critical role in multiple physiological factors regarding dietary protein degradation, protein turnover, cell division, blood coagulation, the transport of polypeptide hormones, and the activation of zymogens [30]. In particular, proteases can optimize feed protein implementation in poultry livestock [17]. In addition, a previous study has demonstrated that proteases employed as feed additives could serve to supplement the effects of endogenous pepsin and pancreatic enzymes via the augmentation of hydrolysis and solubilization of protein in vitro [31]. These effects were confirmed in vivo, where improved protein and fat digestibility coupled with greater growth performance have been identified [31]. Similarly, Ding et al. [32] have reported that supplementation with 300 mg/kg of protease in diets significantly elevates the activity of trypsin within the pancreas and 21 d villus height and/or crypt depth ratio within the duodenum, the jejunum, and the ileum.
Within the present study, CP, DM, and amino acid (lysine, methionine, cysteine, threonine, isoleucine, leucine, histidine, and tryptophan) digestibility and energy retention were linearly raised in broilers using protease supplemented diets when compared with broilers fed non-protease supplemented diets. This response in the sampled broilers is in agreement with findings discovered by Freitas et al., whose team observed higher CP digestibility using corn-soybean-based diets supplemented with increased levels of protease in 42 day old broilers [18]. In addition, Angel et al. have found that exogenous protease supplementation at 0.01%−0.08% improves the Arginine, Threonine, Isoleucine, Asparagin, Lysine, Histidine, Serine, and Cysteine of apparent amino acid digestibility in broiler chickens [17]. Meanwhile, plant protein, like soybean meal, is rich in anti-nutritional factors, in particular protease inhibitors that can suppress the activity of the proteolytic enzymes trypsin and chymotrypsin [33]. A reduction in the activity of these enzymes may lower the protein digestibility and amino acid availability of vegetable-based diets. Therefore, including protease in livestock feed could effectively attenuate the considerable negative effects of unbalanced amino acids and anti-nutritional factors contained in feed materials that primarily consist of plant proteins. In the current study, broilers supplied with protease supplemented diets demonstrated an enhanced BWG and reduced FCR, which could possibly be attributed to higher DM, CP, amino acid digestibility, and energy retention.
The GH/IGF axis is an important modulator of body and muscle growth within vertebrates. Within this system, circulating pituitary GH stimulates the production of IGF-1, a potent mitogen that is mainly responsible for cell growth, and IGF-2. Leptin, the protein product of the obese gene, LEP, has been implicated in several neuroendocrine loops, including the GH/IGF-1 axis. GH, IGF1, IGF2, and LEP are all primary regulators of growth and body composition [34–36]. In the current study, protease treatment increased the expression levels of IGF1, IGF2, GH, and LEP in the liver; however, the direct relationship between protease supplementation and increased IGF1, IGF2, GH, and LEP expression in the liver remains unclear. In a large number of previous reports, the dietary supplementation of essential amino acids, like methionine and leucine, increases the expression of IGF-1 in the liver by stimulating the synthesis and release of GH [37–39]. In addition, Takenaka et al. [40] have demonstrated that the dietary restriction of a single essential amino acid (leucine, lysine, methionine, and tryptophan), or amino acid-free diet, lowers the production of IGF-I within the blood of rats. In addition, Rosebrough and McMurtry [41] noted a decrease in plasma IGF-1 in broilers consuming a low protein diet, which was raised to normal levels via the supplementation of dietary protein. These results are consistent with another study [42] that observed an interaction between GH and amino acids to control IGF1 mRNA expression in a synergistic fashion. We hypothesize that the increase in IGF1, IGF2, GH, and LEP expression in our study after protease supplementation in broiler diets may be caused by improvements in the digestibility of amino acids. However, more research is necessary to identify the direct relationship between protease supplementation and the expression of IGF1, IGF2, GH, and LEP in the liver of broilers.
Liu et al. [43] have previously reported that dietary methionine supplementation can affect muscle cell differentiation and decrease myostatin gene expression in broiler chickens. In addition, Wen et al. [44] have shown that high methionine diets increase the mRNA expression of the MRFs, MRF4 and Myf5, and MEF2A (Myocyte Enhancer Factor 2A) and MEF2B (Myocyte Enhancer Factor 2B) in fast-growing broilers. Furthermore, these diets increase and decrease MEF2A and myostatin mRNA expression, respectively, within slow-growing broilers. Our results are inconsistent with results reported in Liu et al. and Wen et al.; these research teams have reported that broilers supplied with methionine supplements exhibited decreased myostatin gene expression [43,44]. Taken all together, modifications in MRF expression indicate that satellite cell activation, myogenic determination, and muscle cell differentiation may be affected by the bioavailability of proteins, especially methionine.
According to previous reports, nutrient digestibility is directly related to the expression of intestinal nutrient transporters [45–47]. Improved energy digestibility via laminarin, fucoidan, and zinc oxide supplementation is accompanied by increased intestinal glucose transporter expression [46]. Similarly, Drummond et al. have demonstrated that an increase in essential amino acid availability upregulates skeletal muscle amino acid transporter expression, which may constitute an adaptive response that is necessary to improve amino acid intracellular delivery [48]. In addition, dietary lysine-induced alterations to CP digestibility are accompanied by changes in PEPT1, b0,+AT, and CAT-1 gene expression [45]. Osmanyan et al. [49] have reported that increases in PEPT1 and b0,+AT mRNA expression within the jejunum is correlated with increases in digestible amino acids and protein for broiler supplementation from 100% to 114%. In the current study, protease supplementation increased the expression of amino acid transporters within the duodenum, the jejunum, and the ileum of broilers. Therefore, an upregulation of the intestinal amino acid transporters, including SCL6A19, SLC7A1, SLC7A7, SLC7A2, SLC7A6, SLC7A9, and SLC15A1, may be related to the absorption of peptides and amino acids. Further work is required to determine the expression of glucose-related transporters, such as GLUT1 and GLUT2, due to the fact that protease supplementation increased digestibility, including metabolizable energy, in the present study.
CONCLUSION
Supplying broilers with protease in their diets exerted positive effects on the growth performance of broiler chickens. As measured, increased expression levels of growth-related genes and the amino acids transporters indicated that nutrient utilization was heightened with protease. The expression of glucose-related transporters can now be investigated in future research as a marker of improved energy retention.