INTRODUCTION
Global warming may affect the functionality and welfare of animals [1]. Prolonged exposure to high temperatures causes heat stress in animals [2], which in turn leads to a systemic increase in cortisol secretion in the body. As cortisol concentrations in liquid and non-liquid biomatrices vary in different animal species, detecting chronic stress using cortisol measurements is generally challenging [3,4]. Moreover, the collection of liquid biomatrices such as blood is a stressful procedure that can further increase cortisol release, potentially leading to unreliable results [5]. Thus, measurement of cortisol levels using non-liquid biomatrices, such as hair, and semi-solid biomatrices, such as earwax, can be explored as an alternative strategy for chronic stress detection [6,7]. As cortisol accumulates within non-liquid biomatrices over a period of weeks, a single measurement of cortisol levels from non-liquid biomatrices is sufficient for chronic stress detection, whereas multiple measurements are required for detection using liquid biomatrices [8].
In a recent study, earwax cortisol concentration (ECC) was used to measure chronic stress in humans [6]. Earwax has been reported to be a biomatrix that may reflect systemic cortisol concentration more accurately than other biomatrices such as hair, hoof, or nail, which can be contaminated by urine, feces, and saliva, or may have low concentrations of cortisol [6,9].
Earwax is produced and secreted naturally by ceruminous glands and has different physical characteristics, such as wetness, stickiness, yellow or brown color, and the presence of soft or dry substances in the external ear canal, which can be useful for diagnosing diseases and tracking physiological functions in animals [10,11]. Earwax is composed of organic compounds and offers several advantages, including the ability to track long-term changes in cortisol levels (weeks to months) and low external contamination. Additionally, earwax samples are simple to transfer and store, and sample collection does not affect the levels of body metabolites and is non-invasive and painless [10,11].
Cortisol, a lipophilic steroid hormone, is present in the plasma either bound to corticosteroid-binding globulin or free (not bound), with different deposition capacities in various biomatrices [9,12]. Recently, Herane-Vives et al. [6] conducted a study on cortisol extraction from human earwax. They mixed earwax samples in 500 μL of phosphate-buffered saline (PBS) to create a homogeneous solution. Next, they added 500 μL of diethyl ether (DE) to each sample and stirred it for one minute using a vortex. The resulting emulsion was stored at −20°C for 2 hours. Afterward, the liquid portion was transferred to a new 5 mL tube and a displacement method with nitrogen gas (N2) was employed to dry the solution. The dried samples were then resuspended once more in 500 μL of PBS. Finally, cortisol levels were measured from the resulting solution using an immunoassay technique. Selecting a suitable extraction method is important to increase cortisol efficiency. The first step of such extraction procedures involves the use of organic solvents, which separate the analyte (cortisol) from the biomatrix by disrupting the binding between the analyte and protein. Generally, organic solvents have diverse properties, such as polarity, volatility, and toxicity, which can affect the extraction yield and quality of the extracted cortisol. However, the methods used to extract cortisol from earwax have not been standardized, particularly with regard to the choice of extraction solvents. Thus, we hypothesized that the choice of organic solvents for cortisol extraction from earwax can influence the accuracy of stress measurements of the cattle. In this study, to consider the polarity of earwax, two organic solvents, methyl alcohol (MA), as polar and DE, were used for cortisol extraction from earwax to determine whether the type of extraction solvent used affects the extraction efficiency during determination of the ECC of Korean native cattle (Hanwoo). This study evaluated extraction methods involved in ECC determination in cattle and discussed some of the limitations of ECC measurements.
MATERIALS AND METHODS
The experimental procedures and methods were approved by the Institutional Animal Care and Use Committee (IACUC) of Kangwon National University, Chuncheon, Korea (KW-200520-1).
Hanwoo cattle (parity 3 and body weight 462 kg) housed at the livestock research farm of Kangwon National University, Chuncheon, Korea (37°56’24.1”N 127°46’57.1”E).
Earwax samples were prepared prior to the assay as described previously [10]. For safety and to minimize injury during earwax sampling, Hanwoo cattle were head-locked (rope restrained) in the feed space. A surgical spoon (curette) was carefully inserted into the ear canal, but not to go too deep to avoid injury or damage to the ear canal or eardrum. The curette should be inserted to the junction with the horizontal ear canal. Once inserted to appropriate depth, gently rotate the curette to collect earwax (approximately 0.5 g) from both the left and right ears of Hanwoo. The procedure might need to repeat three to four times to collect a sufficient volume of earwax sample. The samples were then placed into Eppendorf tubes (1.5 mL) to prevent contamination during handling and stored in a freezer (−21°C) until further analysis in the laboratory (Fig. 1).
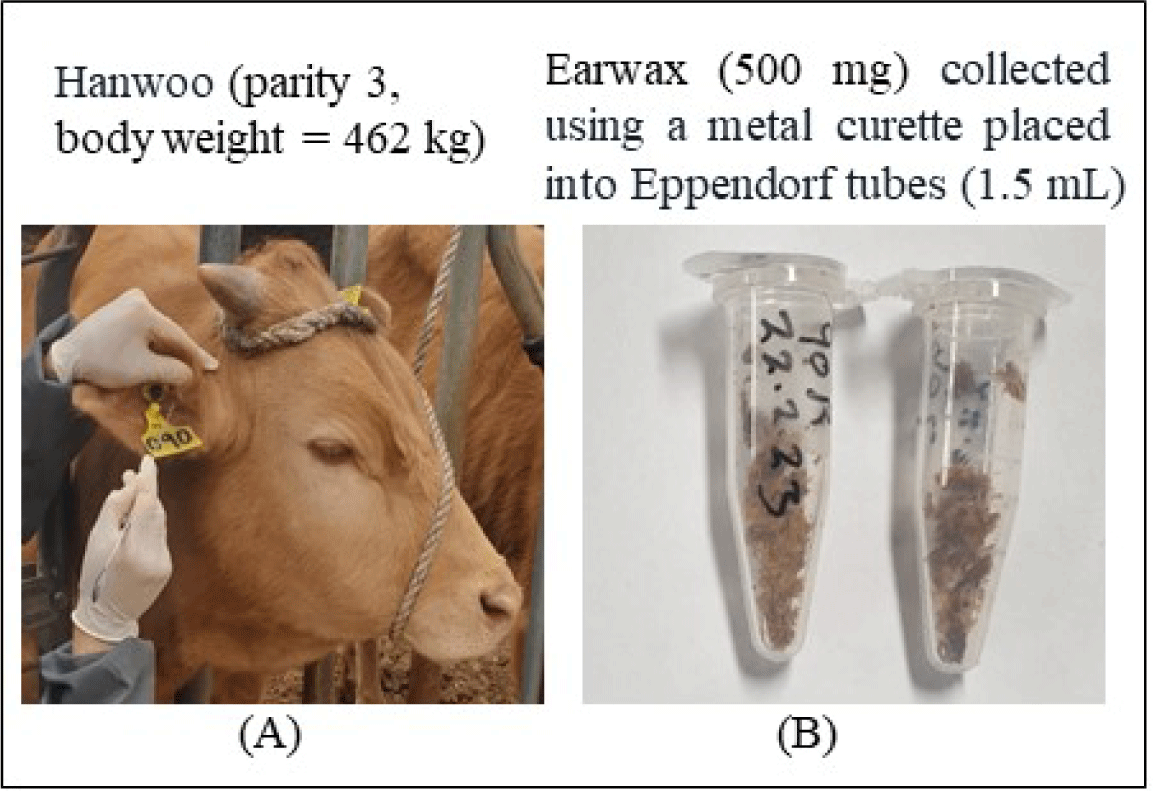
The ECC was measured as described previously with modifications [6,13]. The experimental design and extraction of earwax cortisol using two organic solvents are shown in Fig. 2. In this study, the earwax sample was divided into four portions, each weighing 25 mg. Two portions were placed in separate 5.0 mL polypropylene tubes and then suspended in assay diluent buffer (ADB); the remaining two portions were not suspended in ADB. This procedure was repeated five times (25 mg; n = 5). Subsequently, 1.0 mL of extra pure MA, (99.5%; Daejung Chemicals & Metals, Siheung, Korea) and 1.0 mL of extra pure DE (99%; Samchun Pure Chemicals, Pyeongtaek, Korea) were added to each tube containing the portions with ADB (mixture of organic solvents and ADB). Additionally, 1.0 mL of MA and 1.0 mL of DE were added directly to each tube containing earwax portions without ADB and sealed with Parafilm. Subsequently, all tubes were vortexed for 1 min to homogenize the earwax samples with MA, after which a tube rotator (, MX-RL-Pro, Scilogex, Rocky Hill, CT, USA) was used for continuous shaking for 24 h. The samples were then centrifuged for 10 min at 5000 rpm (VS-5000N, Vision centrifuge, Daejeon, Korea), after which the supernatant was transferred to additional clean tubes and allowed to evaporate in an incubator at 38°C. The samples in the DE-containing tubes were shaken and homogenized, and then allowed to sit for 5 min to facilitate phase separation. The supernatant was then poured into new tubes, and DE extraction was performed an additional two times to increase recovery. Subsequently, the supernatant was collected in the same tubes and placed in an incubator for evaporation. Prior to cortisol analysis using enzyme-linked immunosorbent assay (ELISA), the dried extracted samples were stored at −21°C.
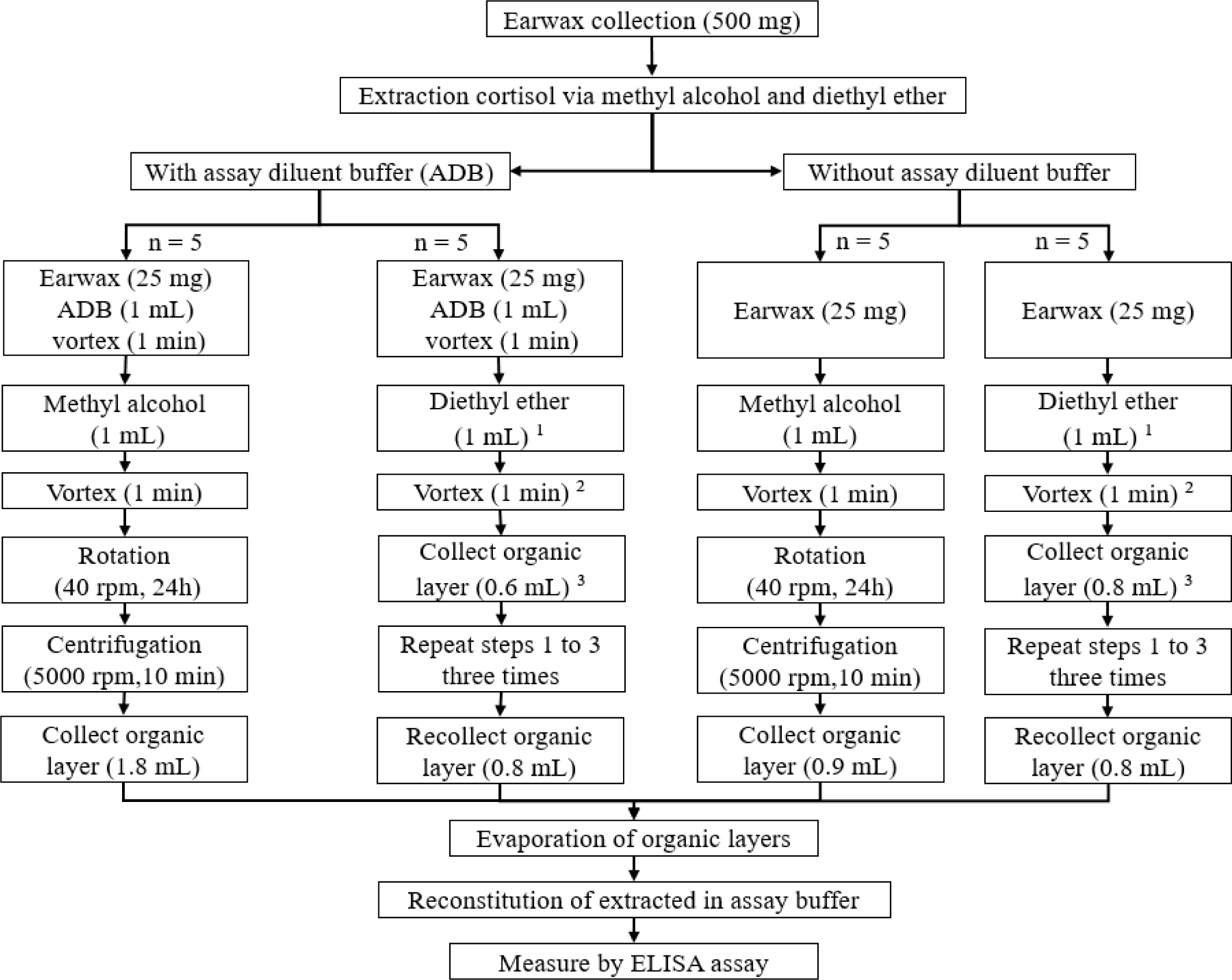
The weight of the earwax samples and the volume of the supernatant solution collected for evaporation are crucial because they enable the determination of the cortisol content in picograms per milligram (pg/mg).
The ECC was assayed using a commercial colorimetric competitive ELISA cortisol kit (ADI-900-071, Enzo Life Sciences, Farmingdale, NY, USA). The kit is designed to detect cortisol in a variety of biomatrices at concentration ranges of 156–10,000 pg/mL. The dried, extracted earwax samples were thawed at room temperature (22°C–24°C) and 0.4 mL of assay buffer of ELISA kit was added to each sample. Each sample was then thoroughly vortexed and centrifuged at 1,500×g for 15 min using refrigerated microcentrifuge machine (Gyrozen 1730R, Gyrozen, Seoul, Korea). Subsequently, all samples (0.1 mL) were run in triplicate in a 96 well-plate to improve assay accuracy and reliability, and absorbance was measured using a microplate reader (SpectraMax absorbance reader, Molecular Devices LLC, San Jose, CA, USA) at a wavelength of 405 nm to determine the color intensity in each well. Cortisol concentration was expressed in pg/mg, according to previous studies on cortisol extraction from Hanwoo cattle hair [14].
Differences between the organic solvent extractions (MA and DE) of earwax cortisol were determined using the general linear model procedure of variance, according to a factorial arrangement (2 × 2). Statistical analysis was conducted using SAS software (version 9.4, SAS Institute, Cary, NC, USA). The mathematical model was as follows:
Where: Y is the observation of ECC. μ is the overall mean, A is the fixed effect of MA. B is the fixed effect of DE, AB is the interaction effect between MA and DE, and ε is the error term, which includes all sources of variability not accounted for by the factors in the model. This model allows us to examine the main effects of each independent variable (A and B), as well as the interaction effect (AB) between the two independent variables. The main effects represent the impact of each independent variable on the dependent variable, while the interaction effect assesses whether the combined effect of both independent variables is different from what would be expected based on their individual effects, and statistical significance was set at p < 0.05.
RESULTS
The combination of the organic solvents (MA and DE) with ADB showed the feasibility of extracting and quantifying earwax cortisol (Fig. 3).
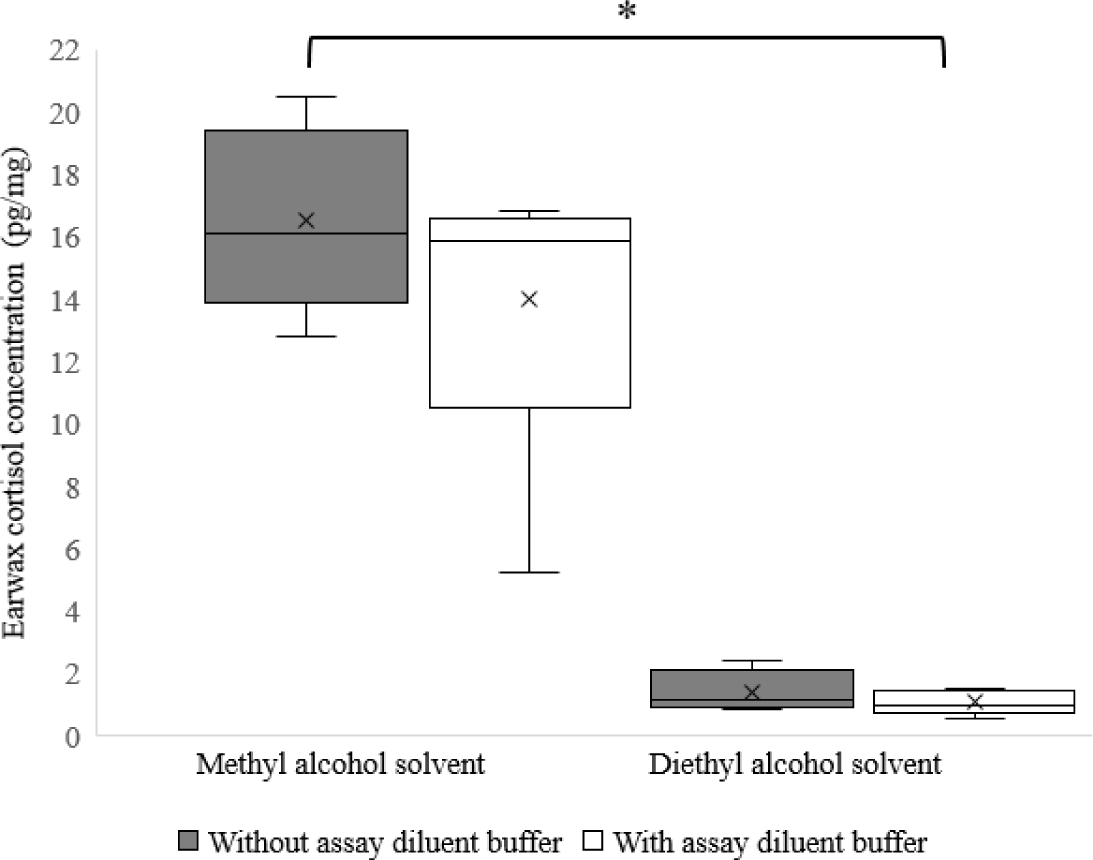
In this investigation, our examination of the interaction between organic solvents and ADB in the extraction of earwax cortisol revealed no statistical effects. The inclusion of ADB in the MA-based extraction process resulted in a significant lower yield of earwax cortisol (p < 0.05) when compared to the MA extraction without ADB. Conversely, no difference (p > 0.05) was shown in the extraction yield of earwax cortisol when ADB was included in the DE-based extraction process (Fig. 3). Furthermore, the extraction yield of earwax cortisol using the MA method was significantly higher (p < 0.05) than that achieved with the DE method (Fig. 3).
DiSCUSSION
Among the biomarkers of stress, cortisol is a major glucocorticoid hormone used to monitor stress conditions in animals. Variety biomatrices, such as blood, urine, saliva, hair, nails, feces, and earwax have been used to measure cortisol concentration [6,9,15,16]; however, as cortisol concentration varies due to the structure and properties of different biomatrices, solvent extraction is used to separate cortisol from biomatrices. The range of cortisol extraction yields can change based on the solvent extraction types, biomatrices, and animal species [17]. Earwax consists of various polar and nonpolar components, such as cholesterol, wax esters, triacylglycerols, and other metabolites [11,18]. Because earwax is a mixture of ceruminous and sebaceous gland secretions with high lipid content [19], cortisol can be transported or diffused from sweat and sebaceous glands to the skin surface of the ear canal [15]. Additionally, as earwax is secreted by apocrine glands [20], earwax production can potentially increase when animals are under stress or during inflammation. The choice of solvents used to extract analytes (the substance of interest) from complex biomatrices depend on the research objectives and available facilities. MA and DE are frequently used for cortisol extraction from liquid and non-liquid biomatrices, and in the present study, both solvents were used to extract cortisol from cattle earwax.
The exact reason for the higher extraction yield of cortisol using MA is currently uncertain; however, a possible explanation may be that the structure of earwax, consisting of a mixture of multiple metabolites of different polarities, results in a high earwax cortisol extraction yield. Another potential explanation for the high extraction yield of earwax cortisol with MA may be due to the solvent properties of MA, such as its polarity and lower molecular weight, which facilitates more efficient extraction of earwax cortisol [8]. The use of MA simplifies extraction as only a single extraction procedure is necessary compared to the multiple extraction procedures required when using DE as the solvent. A simple extraction step is preferable over multiple extraction steps, which may increase the extent of variation. In this study, better cortisol recovery was achieved with MA than with DE. MA and DE have been used as solvents for cortisol extraction in biomatrices such as hair, wool, feces, milk, saliva, serum [9,21–24]; however, Herane-Vives et al. [6], used the PBS to homogenize earwax samples before extracting cortisol using DE. In this study, the ECC showed significant variation in the MA solvent method when suspended in ADB, however, the DE solvent method showed low variation when suspended in ADB. Thus, if the study aims to extract a larger amount of ECC, it is recommended to use MA. However, using MA can lead to higher fluctuations.
In this study, the extraction solvents were evaporated using an incubator at 38°C instead of using laboratory evaporators (e.g., vacuum centrifugal evaporators, nitrogen evaporators); consequently, the evaporation of the mixture of organic solvents with ADB was a time-consuming step during the extraction process owing to the different boiling points of the mixtures and the sample volumes. In this study, using ADB had an impact on the results. It is better to use the MA solvent method without ADB for extracting cortisol from earwax samples. As, cortisol, a steroid hormone, is hydrophobic, dissolves in a large volume of solvents, and may be denatured or destroyed when evaporated to dryness [25]. The optical densities of some earwax samples containing a mixture of DE and ADB were outside the range of the standard ELISA curve, resulting in data errors and those samples were not included in the analysis. It was confirmed that suspending earwax in a high percentage of MA causes the attraction of polar molecules in earwax, resulting in a high ECC with minimal variation in yield. Owing to its nonpolar properties, DE performed poorly during the extraction of earwax cortisol in this study. While, MA is used for steroid hormones extraction, it has shortcomings related to safety, health, and environmental concerns. Researchers should explore alternative solvents, extraction methods, and non-extractive techniques to improve the overall efficiency and safety of the extraction procedure. Although analysis of cortisol from earwax can be used to monitor long-term physiological conditions in animals, it is necessary to validate and optimize the extraction protocol to achieve high extraction efficiency. Moreover, the sample collection procedure must also be considered. For instance, ECC analysis is not possible for cattle or calves that do not provide earwax samples. Furthermore, frequent or improper collection of earwax may lead to injury and infection in cattle.
CONCLUSION
This study describes the collection of earwax samples from cattle and the extraction of cortisol using two types of organic solvents: MA and DE. The choice of solvent affected the concentration of cortisol extracted from the earwax. The results indicate that MA is a preferable solvent for obtaining a high ECC, and the extraction procedure using this solvent was simple, with lower solvent consumption. While, earwax cortisol can be considered as an indicator of chronic stress in Hanwoo cattle, it is important to note the difficulties in collecting and preparing the earwax sample, as well as the safety concerns for both animals and technicians involved in the process. Further research is needed to validate the optimal choice of solvents and precise quantities of earwax necessary for cortisol extraction to achieve standard proportion of the solvents and earwax for the extraction procedure.