INTRODUCTION
Currently, the colonization of the microbiota in the gut of young chicks is the focus of many studies. Commercial hatcheries are a source of gut colonization for chicks after hatching, which can colonize during the growth stage [1]. Pathogens can grow and continuously colonize the gut of chicks because it is an empty ecological niche [2,3]. For decades, antimicrobial growth promoters (AGPs) have been used in poultry diets to improve feed efficiency and maintain intestinal ecosystem balance [4]. However, due to the emergence of bacterial resistance, imbalance in the gut microbiota, and increasing consumer concern about the negative effects of antibiotics, the use of AGP in chicken feed has been banned [5,6]. Schokker et al. [7] reported that post-hatch administration of AGP negatively affected the microbial colonization of broiler chicks at 14 days of age. These revealed problems indicated the need to search for a dietary supplement without AGP [8]. Early administration of dietary supplements after chick hatching is critical for promoting early growth and improving gut function and therefore could be an effective strategy [9,10]. Rapid colonization of the gut with commensal bacteria acts as an environmental factor that influences host physiology, metabolism, and gut health [11,12].
Gum Arabic is a soluble, indigestible dietary fiber naturally secreted from the tears of Acacia Senegal, a plant in the Fabaceae family [13,14]. Gum Arabic is used in many scopes of the food and pharmaceutical industries, especially in conventional medicine to treat a wide range of human diseases [15]. The action mechanism of gum Arabic has been studied in humans, rats, laying hens, and broilers [16,17]. They indicated that since gum Arabic is not broken down in the digestive system, commensal bacteria ferment it instead. This promotes the growth of probiotic bacteria that produce short-chain fatty acids (SCFAs) or other antibacterial compounds, which can improve gut health and consequently affect broiler performance [18,19]. Gum Arabic may inhibit pathogenic bacteria colonization and activate the production of cytokines to regulate immune responses [20]. On the other hand, gum Arabic fibers can be recognized by immune cell receptors, which enhances the host’s immunity [21]. This study hypothesized that the use of gum Arabic (Acacia Senegal) from the first day after hatching could potentially affect intestinal ecosystem parameters (microbiota, immune response, and histomorphological characteristics) and overall growth performance. The aim of the present study was to investigate the effects of gum Arabic supplementation on quantitative microbiota, SCFA concentration, immune-related gene expression, and small intestine morphology in broiler chicks during the early growth phase.
MATERIALS AND METHODS
The King Saud University in Saudi Arabia’s Scientific Research Ethics Committee gave its approval for the current study and the use of all chickens (KSU-SE-20-39).
Insoluble fiber, soluble fiber, hemicelluloses, cellulose, and lignin were analyzed according to the methods of AOAC International [22]. Following the method described by Vázquez-Ortiz et al. [23], the sugar composition of gum Arabic powder, including arabinose and galactose, was determined by High performance liquid chromatography (HPLC; Table 1).
Chemical composition | % |
---|---|
Insoluble fiber | 2.93 |
Soluble fiber | 80.22 |
Hemicelluloses | 1.73 |
Cellulose | 0.23 |
Lignin | 0.97 |
Sugar composition | |
Rhamnose | 8.4 |
Arabinose | 26.0 |
Galactose | 40.18 |
Glucuronic acid | 18.23 |
A total of four hundred thirty-two commercial male broiler chicks (Ross 308) were used from 1 to 24 days of age in this study. Chicks were weighed and then randomly assigned to six dietary treatments with twelve replicate cages of six chicks each. The base diet used was formulated to meet all the nutritional needs of the chicks in mash form during the two phases (starter and grower), according to the recommendations in the Ross 308 Management Guide (Table 2). Dietary treatments (T1 to T6) were supplemented with 0.0, 0.12, 0.25, 0.5, 0.75, and 1.0% gum Arabic powder to the basal diet, respectively. Chicks were raised in environmentally controlled battery cages under similar management and sanitation conditions. For the duration of the study, the chicks had ad libitum access to food and water for 24 hours each day.
Nutritional requirements in the diet was suggested according Management Guide recommendation Ross 308 strain.
Containing mixture supplied per kg of diets: Vit. A: 2,400,000 IU; Vit. D: 1,000,000 IU; Vit. E: 16,000 IU; Vit. K: 800 mg; Vit. B1: 600 mg; Vit. B2: 1,600 mg; Vit. B6: 1,000 mg; Vit. B12: 6 mg; Biotin: 40 mg; Folic Acid: 400 mg; Niacin: 8,000 mg; Pantothenic Acid: 3,000 mg; Cobalt: 80 mg; Copper: 2,000 mg; Iodine: 400 mg; Iron: 1200 mg; Manganese: 18,000 mg; Selenium: 60 mg; Zinc: 14,000 mg.
Growth performance parameters were measured at starter and grower stages from 1 to 24 days. Daily weight gain, feed intake, and feed conversion ratio were calculated [24]. Production efficiency index (PEI) was evaluated using the following formula: PEI = (livability × live weight/age in days × feed conversion ratio) × 100 [25].
At 10 days of age, collection of caecal digesta samples (12 birds per gum Arabic) for analysis of SCFAs according to the method of Aljumaah et al. [26]. Internal standard (mixture of SCFAs) was used (Augsburg, Germany) for procedures of lactate, format, acetate, propionate, butyrate and total SCFA analysis by HPLC Agilent 1260 series. Inertsustain AQ-C18 HP column (4.6 mm × 150 mm i.d., 3 μm) was used for separation. The mobile phase consisted of 0.005 N sulfuric acid. The mobile phase was sequentially programmed in a linear gradient for flow rate from 0–4.5 to 23–25 minutes (0.8 mL/min). The diode array detector was tracked at 210 nm. An injection volume of 5 μL was used for each of the sample solutions. The temperature in the column was maintained at 55°C. Results of SCFA concentrations are expressed as mg SCFA per 1 g of cecal digesta.
Approximately 200 mg of caecal digesta (10 chicks) were collected for counting caecal bacteria according to Gharib-Naseri et al. [27] and Tajudeen et al. [28]. Total DNA extraction was performed using the QIAamp DNA Stool Mini Kit (Qiagen, Germantown, MD, USA) according to the manufacturer’s instructions. Using a Nanodrop spectrophotometer (Nanodrop 2000, Thermo Fisher Scientific, Waltham, MA, USA) and an agarose gel electrophoresis technique, DNA quantity and quality were determined. Extracted DNA from all samples was diluted in nuclease-free water to a concentration of 50 ng/μL. On the Applied Biosystems 7300 Real-Time polymerase chain reaction system (Applied Biosystems, Waltham, MA, USA), 5 bacteria (Table 3) were quantified using the Power SYBR® Green polymerase chain reaction master mix (Applied Biosystems, Thermo Fisher Scientific, Warrington, UK) according to the manufacturer’s instructions. For each target gene, every reaction was performed in triplicate. Thermal cycling was carried out in three stages as follows: one cycle at 50°C for 2 min, followed by 40 cycles at 95°C for 15 s, and finally, 60°C for 1 min. Using a standard curve generated for serially diluted pool DNA at a known concentration (from 102 to 1012 copies/g caecal digesta), quantification of the microbiota in each sample was determined [29]. The result of the quantification of the microbiota was expressed as Log10 per 1 g of caecal digesta.
Approximately one-centimeter-long tissue sections (10 chicks) were taken from the proximal upper part of the jejunum in RNAlater (Qiagen, Hilden, Germany) solution for quantification of gene expression according to Han et al. [30] and Elnagar et al. [31]. The ZymoQuick mRNA extraction kit from Zymo Research, California, USA, was used to isolate mRNA for each sample according to the manufacturer’s instructions. A Nanodrop spectrophotometer (NANODROP 2000, Thermo Scientific) was used to evaluate the absorbance at 260 nm and the 260/280 nm ratio to determine the amount and purity of extracted mRNA. The final concentration of extracted mRNA was diluted to 100 ng/μL for all samples. Subsequently, according to the instructions of the Applied Biosystems reverse transcription kit manufacturer, Thermo Fisher Scientific, UK, it was used to convert total mRNA into complementary DNA (cDNA). The cDNA sample was diluted (1:3) to reduce the template concentration. The quantitative polymerase chain reaction of the cDNA samples was performed by Power SYBR® Green polymerase chain reaction master mix (Applied Biosystems, Thermo Fisher Scientific) with the primers of the target genes (Table 3) using 7300 Real-Time polymerase chain reaction system (Applied Biosystems, Warrington, UK). The reaction for each target gene was performed in duplicate. The cycle threshold (Ct) was determined according to the amplification procedure. Relative quantification was calculated by the 2^-ΔΔ Ct method (2^- [Δ Ct for target gene (Ct value of target gene-Ct value of β-actin as housekeeper)-average Ct value for control sample]). Compared with the control treatment, a fold change in gene expression was calculated.
On day 10 of age, the small intestine of 12 chicks was sampled for each dietary treatment. The relative length and weight of the duodenum, jejunum, and ileum were measured as a percentage of the total small intestine. Small intestinal weight (SI) was expressed as a percentage of live weight. In addition, the weight to length ratio of the intestine was calculated based on its weight and length [32].
Tissues (almost 2 cm) from the middle part of the duodenum, jejunum, and ileum of 12 chicks were collected for each dietary treatment at 10 days of age. According to the procedure indicated by Daneshmand et al. [33], histological sections were prepared. After sectioning, tissue was fixed (10% buffered formalin) for 72 hours, dehydrated (70%–95% ethyl alcohol) for 60 minutes, and embedded using paraffin wax (Tissue-Tek VIP 5 Jr, Sakura, Tokyo, Japan). 5 μm-long sections were cut with a rotary microtome (RM 2255, Leica Biosystems, Nußloch, Germany) and then stained with eosin, hematoxylin, and Alcian blue on slides (CV5030, Leica Biosystems). Histometeric parameters of the small intestine such as villus length (VL), width (W), crypt depth (CD), goblet cells (GC), epithelial thickness (ET), and lamina propria thickness (LPT) were measured (five villi per section) using a light microscope (Nikon, Tokyo, Japan) and image analysis software (AmScope digital camera with attached Ceti England microscope) [34]. In addition, the villus surface area (SA=2π × (W/2) × VL), height of villus length to crypt ratio (VL/CD), and density of goblet cell /100 µm of villus area (GC100) were recorded [35,36].
SAS software [37] was used to analyze all data using one-way variance. A comparison of dietary treatments (T2 to T6) with a based diet (T1) was determined when p < 0.05 is the threshold for statistical significance according to Dunnett’s test. In addition, regression analysis was used to determine whether the dietary treatments produced linear or quadratic responses. The SEM was included in the data presented.
RESULTS
The effects of treatments on the overall performance of male broiler chicks are presented in Table 4. According to Dunnett’s test, the results show that daily weight gain was higher on days 1–5 and 6–10, when chicks received gum Arabic supplementation of 0.12% (T2) compared to T1 (p < 0.05). In contrast, chicks received gum Arabic supplementation (T2 to T5) had higher daily weight gain on days 11–17 compared with T1 (p < 0.05). T2, T3, and T5 dietary treatments on days 1-5, T6 on days 6–10, T2, T3, and T6 on days 11–17, and T2 on days 18–24 had lower daily feed intake (p < 0.05). Feed conversion improved in all dietary treatments during the study phases (p < 0.05), except for T5 and T6 on days 6–10 and 18–24, which had no effect compared to T1. Chicks receiving gum Arabic at T2, T3, and T4 had a higher production efficiency index than T1 during starter and grower stages (p < 0.05). Additionally, a quadratic response of dietary treatments on daily weight gain, feed conversion, and production efficiency index and a linear response on daily feed intake with increasing dietary supplementation was observed (p < 0.05), except for 1–5 and 18–24 with quadratic response.
Dietary treatments from T1 to T6 supplemented by 0.0%, 0.12%, 0.25%, 0.50%, 0.75%, and 1.0% of gum Arabic, respectively.
The effects of treatments on short-chain fatty acids (SCFA) in the caecum of male broiler chicks are presented in Table 5. T2, T3, T5, and T6 had higher concentrations of lactic acid, acetic acid, butyric acid, and total SCFA compared to T1 (p < 0.05 by Dunnett’s test). Dunnett’s test also revealed that chicks fed T2, T5, and T6 had higher formic acid concentrations, and that T2 to T6 had higher propionic acid concentrations compared to T1, with the exception of T5 (p < 0.05). In addition, a linear response of dietary treatments on concentrations of lactic acid, acetic acid, butyric acid, propionic acid, and total SCFA was observed, as well as a quadratic response on formic acid concentration with increasing dietary supplementation (p < 0.05).
Dietary treatments from T1 to T6 supplemented by 0.0%, 0.12%, 0.25%, 0.50%, 0.75%, and 1.0% of gum Arabic, respectively.
The effects of treatments on quantification of the caecal microbiota of male broiler chicks are presented in Fig. 1. When chicks received T3 to T6, quantification of Lactobacillus spp. was increased compared to T1 (p = 0.017 by Dunnett’s test). While Bacteroides spp. was reduced in chicks receiving T3 and T5 compared to T1 (p = 0.036). In addition, Bifidobacteria spp., Clostridium spp. and Escherichia coli (E. coli) showed no effect in chicks receiving dietary supplements compared to T1 (p > 0.05). In addition, there was a linear response to treatments in Lactobacillus spp. and Bacteroides spp. (p < 0.05), but other quantifiable bacteria did not respond linearly or quadratically to treatments (p > 0.05).
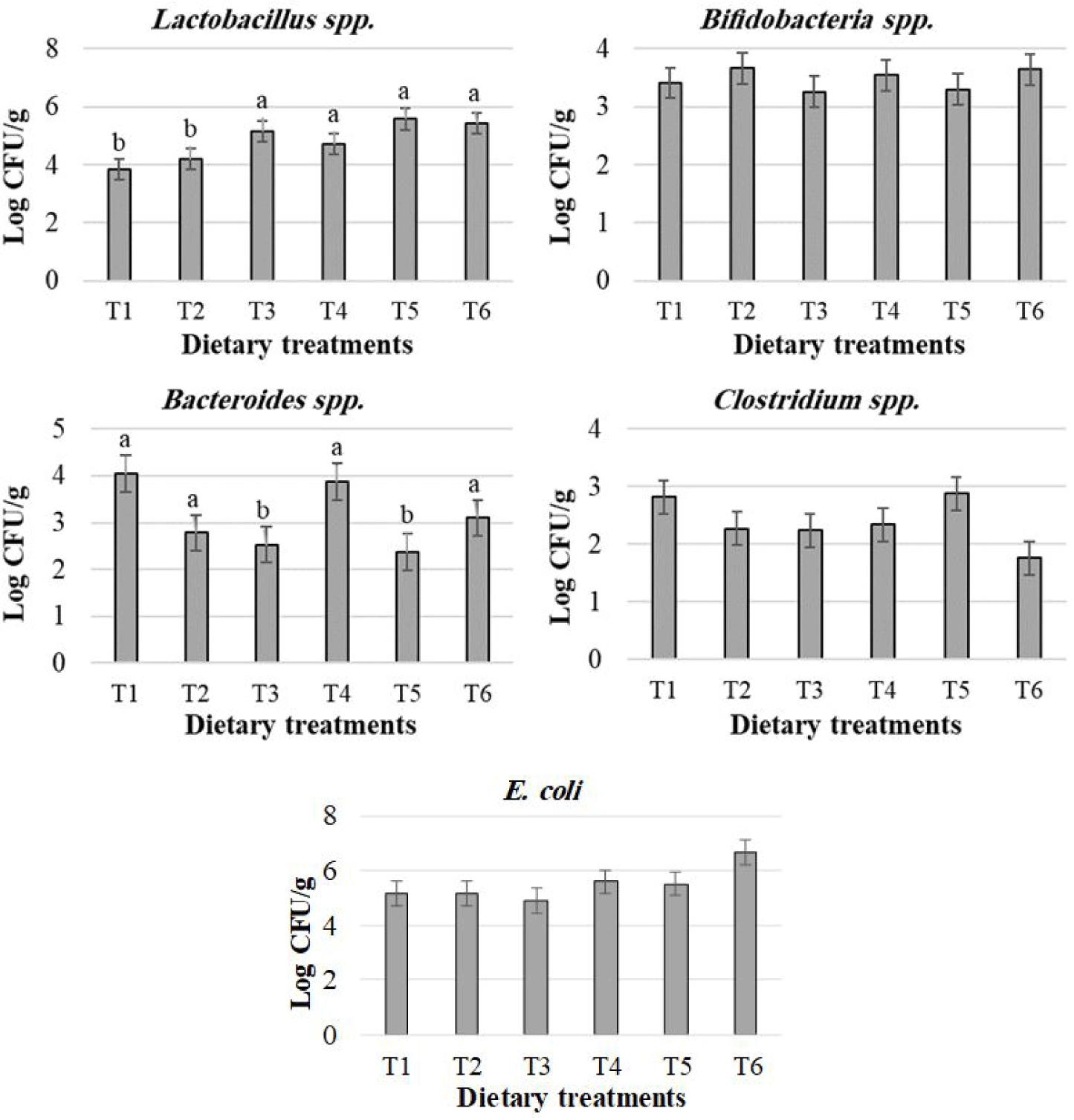
The effects of treatments on pre-inflammatory cytokines expression in male broiler chicks are presented in Fig. 2. When chicks received T2 to T6 compared to T1, fold change in interleukin (IL)-1β and tumor necrosis factor (TNF)-α expression was reduced (p < 0.05 by Dunnett’s test). In contrast, IL-12 and interferon (INF)-Y expression was increased in chicks receiving T6 compared to T1 (p < 0.05) as determined by Dunnett’s test. In addition, there was a quadratic response to IL-1β, IL-12, and TNF-α (p < 0.05), but expression of INF -Y showed no linear or quadratic response to dietary treatments (p > 0.05).
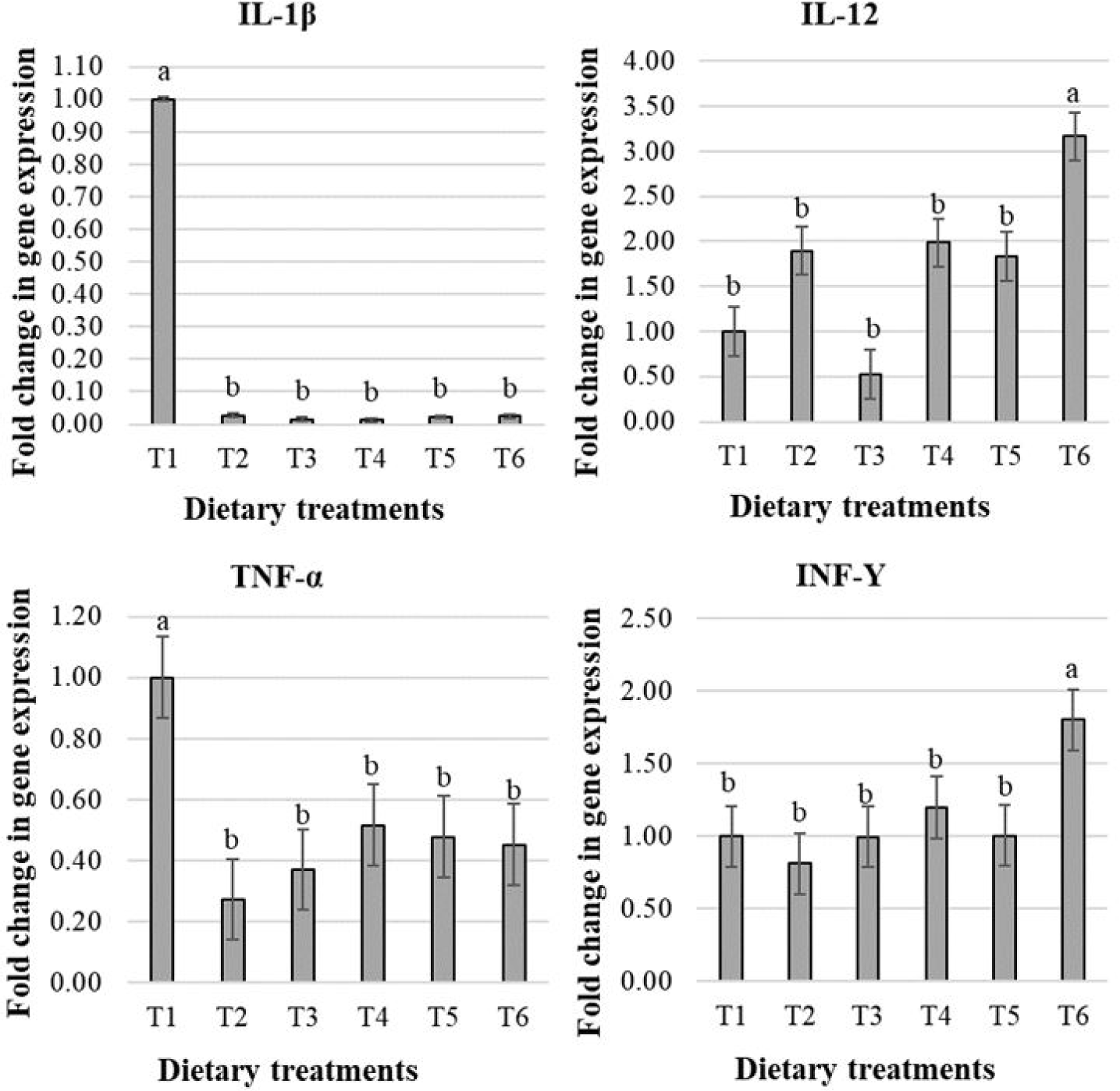
The effects of treatments on mucin-2 protein (MUC-2) expression in male broiler chicks are presented in Fig. 3. The fold change in MUC-2 expression was increased in chicks receiving T2 and decreased in chicks receiving T6 compared to T1 (p < 0.05 by Dunnett’s test), and a quadratic response with dietary treatments was observed (p < 0.05).
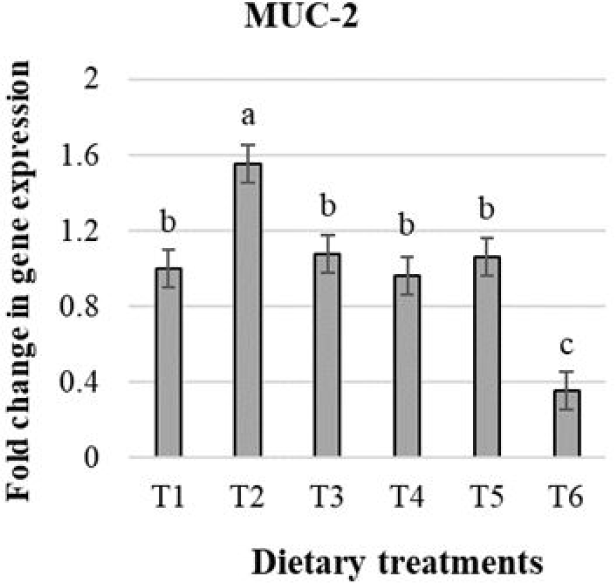
The effects of treatments on the expression of secretory SIgA in male broiler chicks are presented in Fig. 4. According to Dunnett’s test, chicks receiving T4 and T5 had higher SIgA expression than T1 (p < 0.05), and a linear response with dietary treatments was observed (p < 0.05).
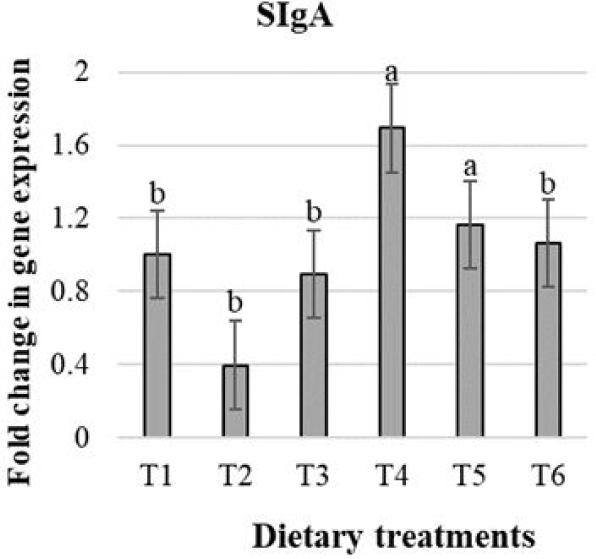
The effects of treatments on small intestine morphology in broiler chicks are presented in Table 6. The ratio between weight and length of small intestine was higher in T2 than in chicks receiving the basal diet (T1; p < 0.05), and a quadratic response was observed (p < 0.05). Furthermore, the histomorphology of other small intestinal fragments was not affected by treatments (p > 0.05 by Dunnett’s test) and showed no linear or quadratic response (p > 0.05).
Dietary treatments from T1 to T6 supplemented by 0.0%, 0.12%, 0.25%, 0.50%, 0.75%, and 1.0% of gum Arabic, respectively.
The effects of treatments on small intestinal histometry of broiler chicks are presented in Table 7 and Fig. 5. In duodenal tissue, VL, SA, and VL/CD were higher in T2 to T6, while LPT was lower compared to T1 (p < 0.05 by Dunnett test). Villus width (W) in T3, GC in T2 and ET in T5 were increased compared to T1 (p < 0.05). Furthermore, there was no linear or quadratic response (p > 0.05) for ET, but there was a quadratic response to VL, W, SA, VL /CD, and LPT, as well as a linear response to GC and GC100 (p < 0.05).
Dietary treatments from T1 to T6 supplemented by 0.0%, 0.12%, 0.25%, 0.50%, 0.75%, and 1.0% of gum Arabic, respectively.
Means that do not share a common superscripted with control treatment (T1) within a row for each parameter has a significant effect, as determined by the Dunnett test (p < 0.05).
SEM, standard error of means for diet effect; GA, gum Arabic levels effect; L, linear response; Q, quadratic response; VL, length; W, width; SA, villus surface area (mm2); VL/CD, villus length/crypt depth; GC, goblet cells; GC/100, goblet cells / 100 µm villi area; ET, epithelial thickness; LPT, lamina propria thickness.
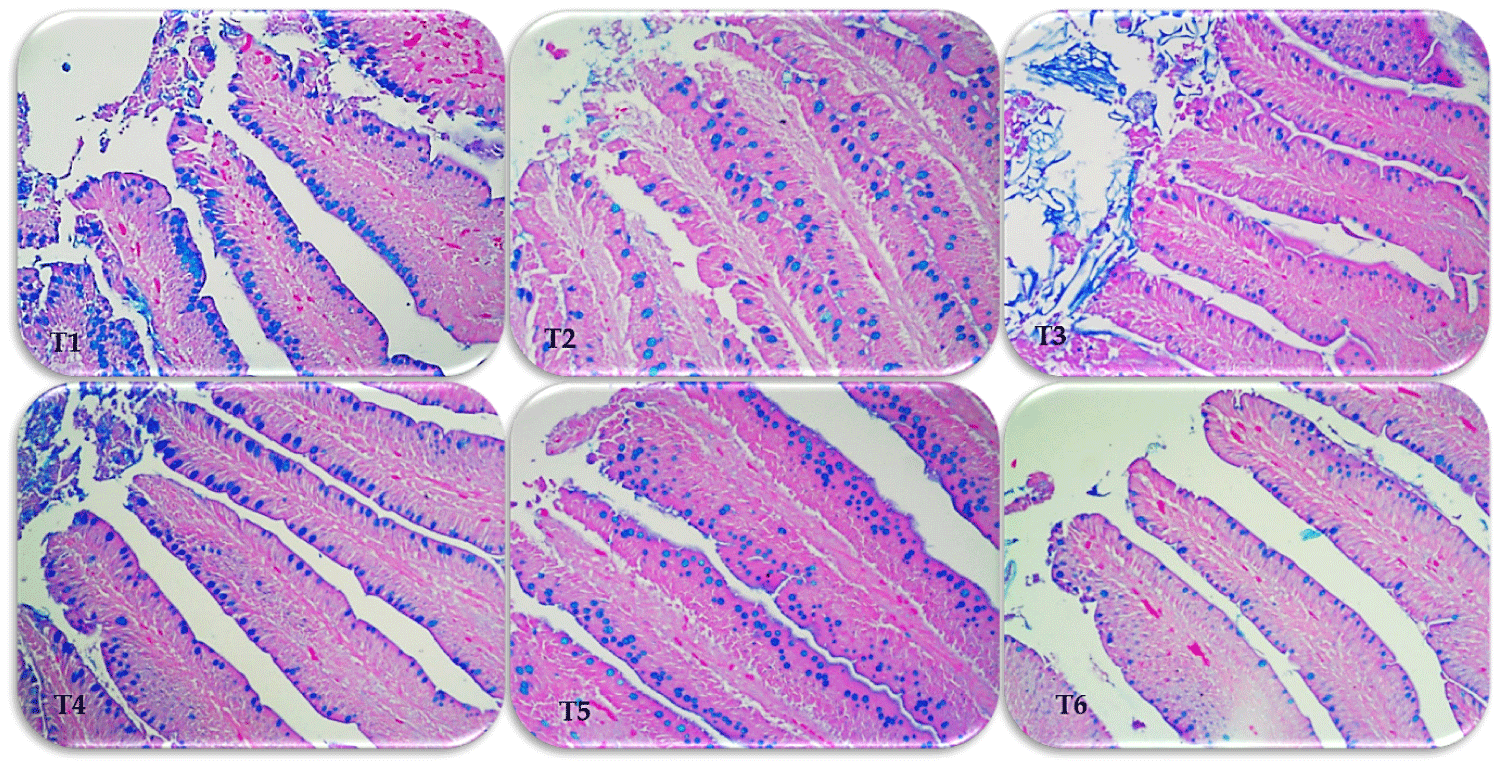
In jejunum tissue, chicks fed T2 to T6 showed higher VL, SA, VL /CD, and GC compared with tissue from chicks fed T1 (p < 0.05 by Dunnett’s test). In addition, W and ET of jejunum tissue were increased when broiler chicks were fed T2 and T4, as well as LPT, which was higher at T2 and lower at T6 than at T1 (p < 0.05). Furthermore, there was a quadratic response with treatments in all histometric measurements (p < 0.05).
In ileum tissue, chicks fed T2 to T5 had higher VL, SA, and LPT, as well as T2, T3, and T5 had higher W and GC compared with tissue from chicks receiving T1 (p < 0.05 by Dunnett test). In addition, VL/CD and ET of ileum tissue were increased in chicks fed T2 and T5 compared to T1 (p < 0.05). Furthermore, there was a quadratic response to VL, W, SA, GC, and LPT while linear response to VL/CD and ET with treatments (p < 0.05).
DISCUSSION
Modification of the gut microbiota content has an important impact on gut development, physiological functions, and SCFA production in chicks, especially in the post-hatching period [11]. Gum Arabic is a soluble and indigestible dietary fiber in the small intestine of chicks. Therefore, soluble dietary fiber can stimulate the metabolic activities of commensal bacteria to produce SCFAs through a fermentation process, which potentially has a positive effect on host health and thus improves broiler growth performance [18,19]. The current results show that dietary supplementation with gum Arabic improves daily weight gain, feed conversion ratio, and production efficiency index compared to control group (T1). These results are in agreement with those of Tabidi & Ekram [38], who showed that the addition of gum Arabic (0.6%) to the basal diet improved the overall performance of broilers. However, daily feed intake was lower at T2, T3, and T5 (1 to 5 days old) and at T6 (6 to 10 days old). According to Dreher [39], gum Arabic can reduce feed intake by increasing satiety. Administration of 10% gum Arabic for 15 weeks decreased feed intake in mice [40]. Production efficiency index is often used as an expression of the economic status of broiler production [41]. Thus, a higher production efficiency index indicates better performance when chicks receive gum Arabic.
The metabolites of the gut microbiota (SCFAs), which include lactate, format, acetate, propionate, and butyrate, play critical role in maintaining the structural and functional integrity of the gut [42]. According to the current study, broiler chicks fed the diet treatments (T2, T3, T5, and T6) had higher concentrations of lactate, acetate, propionate, butyrate, and total SCFA in their cecum. These results may be indicative of the ability of gum Arabic to ferment and produce SCFA during the starter phase (10 days). The type of dietary fiber and the degree of fermentation in chicks may have an effect on SCFA concentrations [43]. In a study by Teng and Kim [21], gum Arabic was reported to improve gut health by stimulating lactobacilli in young chicks. Lactobacillus spp. have antipathogenic bacterial properties [44]. This property might be the reason why the administration of gum Arabic (T2, T3, T4 and T6) decreased the number of Clostridium spp. but had no significant effect compared to the control group (T1). Menconi et al. [45] reported that SCFAs have antimicrobial properties by penetrating the cell membrane of gram-negative bacteria and lowering pH. Al Alawi et al. [46] reported that the antibacterial activity of gum Arabic may be due to a high concentration of nonpolar components. The aqueous extract of gum Arabic inhibited Clostridium spp. [47]. Bacteroides spp. have strong metabolic activity by efficiently fermenting indigestible polysaccharides to SCFA, thus protecting the host from pathogen infection [48]. Gum Arabic promotes the growth of bifidobacteria in the human intestine [49]. However, some Bacteroides species have been reported to encode sugar-degrading enzymes in gum Arabic in vitro [50]. Moreover, administration of gum Arabic increased the quantity of Bifidobacteria and Bacteroides in human intestine and simulation models, respectively [16]. However, our results showed that gum Arabic had no effect on the quantity of Bifidobacteria spp. and E. coli.
Furthermore, we discovered that the expression of IL-1 and TNF- (T2 to T6), whereas IL-12 and INF-Y was increased in T6. MUC-2 expression was reduced in chicks receiving T6 and increased in T2, while chicks receiving T4 and T5 had higher SIgA expression. Kogut [20] reported that prebiotic fibers may include gum Arabic can act as non-pathogenic antigens by being recognized by immune cell receptors that positively influence host immunity. Prebiotics increased MUC gene expression, which is related to mucin secretion [51]. In our results, the greater number of GCs by gum Arabic could increase mucin expression and synthesis, which plays a critical role as the first line of defense. Mucin can prevent the invasion of pathogens into epithelial cells [52]. In a previous study, feed supplementation with prebiotics (0.2% mannan oligosaccharide [MOS]) increased gene expression of IL-12 and IFN-Y in broilers [53]. Prebiotics can strengthen intestinal barrier function by increasing the number of GCs and immunoglobulin A (IgA)-secreting cells, as shown by Shao et al. [54]. Important immunoglobulin known as secretory sIgA acts as the first line of defense against any pathogenic bacteria on the intestinal mucosa [55]. Kamal et al. [56] found that gum Arabic decreased inflammatory biomarkers in humans. In addition, gum Arabic decreased TNF-α expression in rats [57].
A healthy small intestine with a balanced microbiota is necessary for enhanced growth performance and feed utilization [58,59]. On the other hand, the intestine has a large surface area and shallow crypts for maximum absorption [60]. The most used histometeric indicators for assessing the growth and the intestine health in broiler chickens are VL and VL/CD [10,61]. However, the VL is associated with active cell mitosis, and the VL/CD height ratio to increase absorptive capacity and epithelial cell turnover may indicate proliferative activity the villi in addition to the CD height [62,63]. Our results showed that from T2 to T5, ileum histometric parameters (VL, W, SA, VL /CD, GC, ET, and LPT) increased. In principle, a greater VL, SA, and VL/CD ratio could improve intestinal structure, digestion, and nutritional absorption, making this technique a useful method to improve performance and intestine development. In a study by Lan et al. [64] reported that gum Arabic could quantitative change microbiota and improve intestinal structure, thereby enhancing growth performance. Moreover, gum Arabic can improve the integrity of intestinal epithelial in broilers as suggested by Liu et al. [65].
CONCLUSION
Chemical composition results confirmed that gum Arabic contains soluble fiber (galactose, arabinose, glucuronic acid, and rhamnose), which could be used as a feed additive for broilers. Therefore, we conclude that administration of gum Arabic resulted in improvements in overall performance, fermentation metabolites, and a change in microbiota and immune response with improved histomorphometry in the intestine of young chicks. Further studies are needed to determine the possible mechanism of gum Arabic and confirm the optimal level of gum Arabic at different growth stages of broilers.