INTRODUCTION
Various issues have emerged associated with improving livestock manure management (LM) due to the increasing demand for animal-sourced foods [1]. LM generally contains heavy metals (arsenic, copper, and zinc) that can be hazardous to humans and the environment. It also generates various harmful compounds (e.g., volatile fatty acids, alcohols, amines, hydrogen sulfide [H2S], and ammonia [NH3]) that can cause an unpleasant odor [2]. In particular, NH3 is produced at a higher concentration than other odorous gases. It is involved in air pollution as a precursor to secondary ultrafine dust [3].
Composting is the primary method of LM treatment applied on a farm [4]. However, the process of composting can have various adverse effects such as nitrogen loss and increase in greenhouse gas due to rapid degradation of nitrogenous organic matter and the presence of anaerobic space in feedstocks [5]. Previous studies have shown that nitrogen losses during composting can result in the production of NH3, nitrous oxide, and leachate, which can reduce the agricultural value of composted products, and contribute to increase in greenhouse gas emissions and unpleasant odor [6].
Probiotics have been shown to improve the environment of digestive organ microorganisms by reducing harmful microorganisms in the intestine, resulting in improved nitrogen utilization and reduced nitrogen excretion in pigs [7]. Li and Kim [8] have reported that supplementation of Saccharomyces cerevisiae (S. cerevisiae) can improve nitrogen digestibility in growing pigs. Other studies have suggested that supplementation including Bacillus subtilis (B. subtilis) can attenuate NH3 release by suppressing the urease-producing microorganisms in the gastrointestinal lumen by producing the protein-digesting enzyme such as subtilisin in pigs [8]. Wang et al. [9], have reported that S. cerevisiae can reduce the Methanobrevibacter spp. known to produce methanogen and methane. According to results of previous studies, supplementation containing Bacillus spp. might reduce NH3 emissions in pigs [8,10].
However, there are few studies on adding complex probiotics to swine diets and manure. Therefore, the objective of this study was to determine effects of single and complex probiotics in growing-finishing pigs and compost on growth performance, odorous gas emissions, blood profiles, and compost maturity.
MATERIALS AND METHODS
All experimental procedures received prior approval from the Animal Ethics Committee of Chungbuk National University (CBNUA-1740-22-02).
The probiotics used in the current study were kindly provided by a commercial company (Garam Co. Ltd., Eumseong, Korea).
A total of 64 crossbred growing pigs ([Landrace × Yorkshire] × Duroc) with an average initial body weight (BW) of 18.75 ± 0.33 kg and a birth of 63 days were used for 13 weeks in this study. All pigs were assigned to a completely randomized four treatment groups based on the initial BW. There were 4 pigs in a pen with 4 replicate pens for each treatment. Dietary treatments were as follows: 1) Control (CON; basal diet), 2) T1 (CON + 0.2% B. subtilis), 3) T2 (CON + 0.2% S. cerevisiae), 4) T3 (CON + 0.2% B. subtilis + 0.2% S. cerevisiae). The probiotic used in this study such as B. subtilis and S. cerevisiae contains 2.0 × 1010 CFU kg−1 and 3.0 × 1010 CFU kg−1, respectively. All diets were formulated to meet or exceed the NRC [11] requirement (Table 1). The diet was divided into two phases: the grower phase (0–6 weeks) and the finisher phase (7–13 weeks). Each of the pigs had ad libitum access to water. A nipple drinker and single-sided stainless steel automated feeder were placed with each pen.
Provided per kilogram of complete diet: vitamin A, 11,025 U; vitamin D3,1,103 U; vitamin E, 44 U; vitamin K, 4.4 mg; riboflavin, 8.3 mg; niacin, 50 mg; thiamine, 4 mg; d-pantothenic, 29 mg; choline, 166 mg; and vitamin B12, 33 µg; Cu (as CuSO4 · 5H2O), 12 mg; Zn (as ZnSO4), 85 mg; Mn (as MnO2), 8 mg; I (as KI), 0.28 mg; and selenium (as Na2SeO3 · 5H2O), 0.15 mg.
BW was recorded on initial, 6, 9, and 13 weeks to calculate average daily gain (ADG), average daily feed intake (ADFI), and feed conversion ratio (FCR). The ADG was calculated by subtracting the BW of the previous time point from the BW of the current time point and dividing it by the period. ADFI was calculated by subtracting the remaining feed amount from the initial feed amount and dividing it by the period, and FCR was calculated by dividing feed intake by ADG.
In experiment periods, fresh fecal samples are collected at 6 and 13 weeks using rectal massage after each treatment. Fresh fecal and feed samples were stored in a freezer at −20°C after collection immediately. The stored fecal samples were dried at 70°C for 3 days and then crushed on a 1 mm screen at the end of the experiment. Chromic oxide was analyzed immediately after supplementation of 0.2% as an indigestible marker that was added to the pig’s diet the apparent total tract digestibility (ATTD) of crude protein (CP), dry matter (DM), and gross energy (GE). Chromium levels were analyzed with ultraviolet absorption spectrometry (UV-1201, Shimadzu, Kyoto, Japan) using a method used by Williams et al. [12]. The procedures utilized for the determination of DM (method 930.15), and CP (method 999.03) were conducted with the methods of AOAC [13], and GE using a bomb calorimeter (Parr 6400 Bomb Calorimeter, Parr Instrument, Moline, IL, USA).
Calculating the ATTD used the following formula:
Nf = nutrient concentration in feces (DM %), Nd = nutrient concentration in diet (DM %), Cd = chromium concentration in diet (DM %), and Cf = chromium concentration in feces (DM %).
At 6 and 13 weeks, blood samples from the anterior vena cava of 4 pigs per treatment. Blood samples were collected into vacuum tubes containing K3EDTA (Becton, Dickinson and Co., Franklin Lakes, NJ, USA) for complete blood count analysis, and nonheparinized tubes for serum analysis, respectively. After collection, serum samples were centrifuged (3,000×g) for 15 min at 4°C. The white blood cell (WBC), and red blood cell (RBC) levels were determined using an automatic blood analyzer (ADVIA 120®, Bayer Lab, NY, USA). The blood urea nitrogen (BUN), creatinine, and total protein levels were measured using a chemistry analyzer (Cobas C702, Roche, Munich, Germany).
Compost was used by mixing sawdust with manure obtained from pigs. The manure was collected at the 6 and 13 weeks. Compost was prepared by adding sawdust to swine manure at a ratio of 4:1 (swine manure: sawdust) for adjusting moisture content (MC) at the beginning of the experiment. Each compost was stored in a plastic box with air holes. Each compost was mixed weekly to supply oxygen. Compost maturity was evaluated using a maturity analyzer (CoMMe-100, E&A TECH, Dangjin, Korea), according to maturity analyses specified in the fertilizer quality inspection and sampling standards in Korea. MC was adjusted to be around 50% for all samples before they were analyzed for maturity score according to methods described by Song et al. [14]: score 1, immature (barely progressing in compost maturity); score of 2, initial maturity (an initial state in which maturity progressed); score of 3, the middle of maturity (compost maturity in which a longer stay was required); score of 4, the latter part of maturity (compost was almost mature); score of 5, maturity completion (compost was mature). The samples collected at 6 weeks were ripened for 14 weeks, and the samples collected at 13 weeks were ripened for 13 weeks.
The feces (150 g) that collected for 2 pigs each treated by a rectal massage at 6 and 13 weeks. The samples were mixed with 150 g of collected feces 100 g of sawdust, and 50 g of urine to analyze gas emissions. About samples were stored in a 4.2 L plastic box at room temperature 26°C and fermented for 72 h. The plastic boxes with small holes sealed with plaster were used for analyzing fecal NH3, H2S, and acetic acid (CH3COOH) emissions of samples. The samples with plastic boxes are shaken 20 s to break down any crust formation before the measurement. NH3 concentrations were determined within the scope of 5.0–100.0 ppm (No.3La, detection tube, Gastec, Kanagawa, Japan), H2S concentrations were determined within scope of 2.0–20.0 ppm (No.4LK, detection tube, Gastec), and CH3COOH concentrations were determined within the scope of 2.5–10.0 ppm (No.81L, detection tube, Gastec, Kanagawa, Japan).
The samples of fresh fecal were collected by rectal massage at 6 and 13 weeks from 4 pigs in each treatment by rectal massage. The samples were immediately packaged in plastic bags and transferred to the laboratory freezer (−20°C) for the duration of the experiment. To count the number of Lactobacillus and Escherichia coli (E. coli), 1 g of samples from each treatment were diluted with 9 mL of 1 % peptone broth (Becton, Dickinson and Co) and homogenized. In 6-fold to 4-fold dilution (1 % peptone solution) samples were used to analyze the viability of E. coli on MacConkey agar plates (Difco Laboratories, Detroit, MI, USA) and Lactobacillus on de Man, Rogosa, and Sharpe agar plates (Difco Laboratories) respectively. E. coli were incubated at 37°C for 24 h and Lactobacillus were incubated for 48 h.
This study used swine manure and sawdust as raw materials for composting. Swine manure from CON of experiment 1 was obtained. The manure was collected from all CON replicates and mixed. The compost was prepared with the same method as experiment 1. The compost was matured in a 12 L plastic box and mixed weekly to provide oxygen and ripening at ambient temperature for 12 weeks. There were 6 replicates for each treatment. Experimental treatments were as follows: 1) CON, normal compost without probiotics; 2) T1, spray B. subtilis 10 g per 3.306 mm2; 3) T2, spray B. subtilis 40 g per 3.306 mm2; 4) T3, spray S. cerevisiae 10 g per 3.306 mm2; 5) T4, spray S. cerevisiae 40 g per 3.306 mm2; 6) T5, spray B. subtilis 5 g + S. cerevisiae 5 g per 3.306 mm2; 7) T6, spray B. subtilis 20 g + S. cerevisiae 20 g per 3.306 m2. In this study, 2.0 × 1010 CFU kg−1 of B. subtilis and 3.0 × 1010 CFU kg-1 of S.cerevisiae were used.
The collected compost samples were determined before and after drying at 105°C for 24 h to analyze for MC according to methods and calculating formula described by Singh et al. [15].
Calculating the MC used the following formula:
Ww = weight of the sample before drying, Wd = weight of the sample after drying.
Initially, the samples were agitated and collected 300 g on 1, 4, 8, and 12 weeks, respectively. About 300 g samples were stored in a 4.2 L plastic box at room temperature 26°C and fermented for 72 h. The plastic boxes with small holes sealed with plaster were used for analyzing H2S, NH3, and methyl mercaptan (CH3SH) emissions of samples. The samples with plastic boxes are shaken for 20 s to break down any crust formation before the measurement. H2S concentrations were determined within scope of 2.0–20.0 ppm (No.4LK, detection tube, Gastec), NH3 concentrations were determined within scope of 5.0–100.0 ppm (No.3La, detection tube, Gastec), and CH3SH concentrations were determined within scope of 2.5–70.0 ppm (No.71, detection tube, Gastec).
All data excluding compost maturity were analyzed with the PROC General Linear Models procedure of SAS 9.4 software (version 9.4, SAS Institute, Cary, NC, USA). The maturity score was analyzed with a Chi-square test using the FREQ procedure of SAS. The GraphPad Prism 8 software (GraphPad Software, San Diego, CA, USA) was used to visualize the maturity score and MC. Tukey’s multiple range test was used as post-hoc test was used to analyze the differences between means and a p < 0.05 was considered statistically significant for analysis.
Results
The effects of supplemental probiotics on growth performance are presented in Table 2. There was no significant difference (p > 0.05) in ADG, ADFI and FCR in 0–6 weeks and 6–13 weeks among treatments. In BW, there was no significant difference (p > 0.05) at initial, 6 weeks and 9 weeks, respectively. However, T3 showed significantly higher (p < 0.05) BW than CON in 13 weeks, significantly higher (p < 0.05) ADG than CON and T2 in 9–13 weeks, and significantly lower (p < 0.05) FCR than the other groups for 9-13 weeks and the overall experimental period (0–13 weeks), respectively. Additionally, T3 showed significantly higher (p < 0.001) ADG than other groups in the overall experimental period.
The effects of supplemental probiotics on nutrient digestibility are presented in Table 3. There was no significant difference (p > 0.05) in DM, GE, and CP at 6 weeks among treatments. Also, there was no significant difference (p < 0.05) in GE, and CP at 13 weeks among treatments. However, T3 showed significantly higher (p < 0.05) DM than CON at 13 weeks.
The effects of supplemental probiotics on blood profile are presented in Table 4. There was no significant difference (p > 0.05) in total protein, creatinine, WBC, and RBC at 6 weeks among treatments. Probiotics did not affect the total protein, BUN, creatinine, WBC, and RBC at 13 weeks. However, supplementation of probiotic groups was significantly lower (p < 0.05) BUN than non-supplementation of the probiotic group at 6 weeks.
The effects of supplemental probiotics on the maturity score of pigs (Exp 1) are presented in (Fig. 1. The chi-square test showed no difference (p > 0.05) in the maturity score among treatments. However, supplementation of probiotic groups was significantly higher (p < 0.001) average maturity score than non-supplementation of the probiotic group at 6 weeks. Moreover, T3 showed a significantly higher (p < 0.001) average maturity score than other groups at 13 weeks.
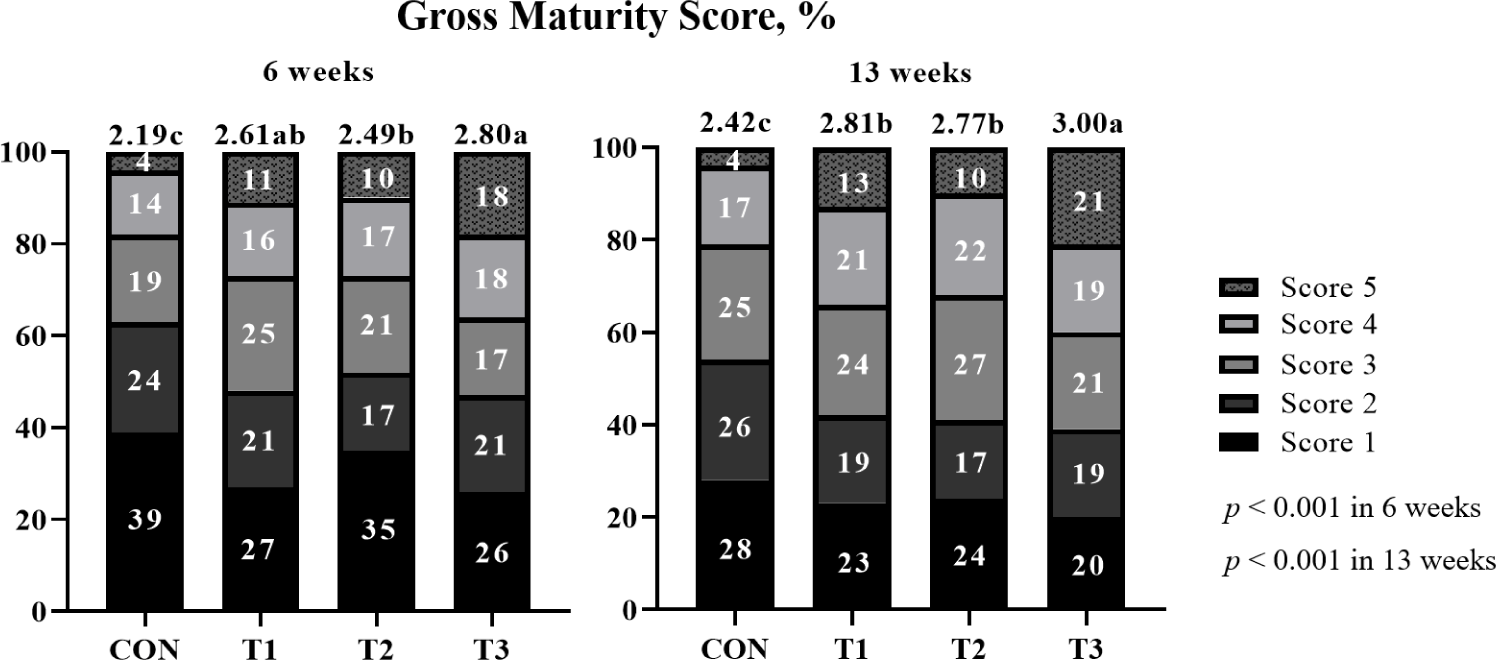
The effects of supplemental probiotics on gas emissions are presented in Table 5. There was no significant difference (p > 0.05) in H2S, NH3, and CH3COOH at 6 weeks among treatments. Also, there was no significant difference (p > 0.05) in NH3 and CH3COOH at 13 weeks among treatments. However, T3 showed significantly lower (p < 0.05) H2S than CON at 13 weeks.
The effects of supplemental probiotics on fecal microflora are presented in Table 6. There was no significant difference (p > 0.05) in Lactobacillus at 6 weeks among treatments. Also, there was no significant difference (p > 0.05) in E. coli at 13 weeks among treatments. However, T1 and T3 showed significantly lower (p < 0.001) E. coli than CON and T2 at 6 weeks. Moreover, T3 showed significantly higher (p < 0.05) Lactobacillus than CON and T2 at 13 weeks.
The effects of supplemental probiotics on MC of compost are presented in (Fig. 2. Supplementation of probiotic groups was only numerically decreasing (p > 0.05) MC compared to the non-supplementation group for 6 to 12 weeks.
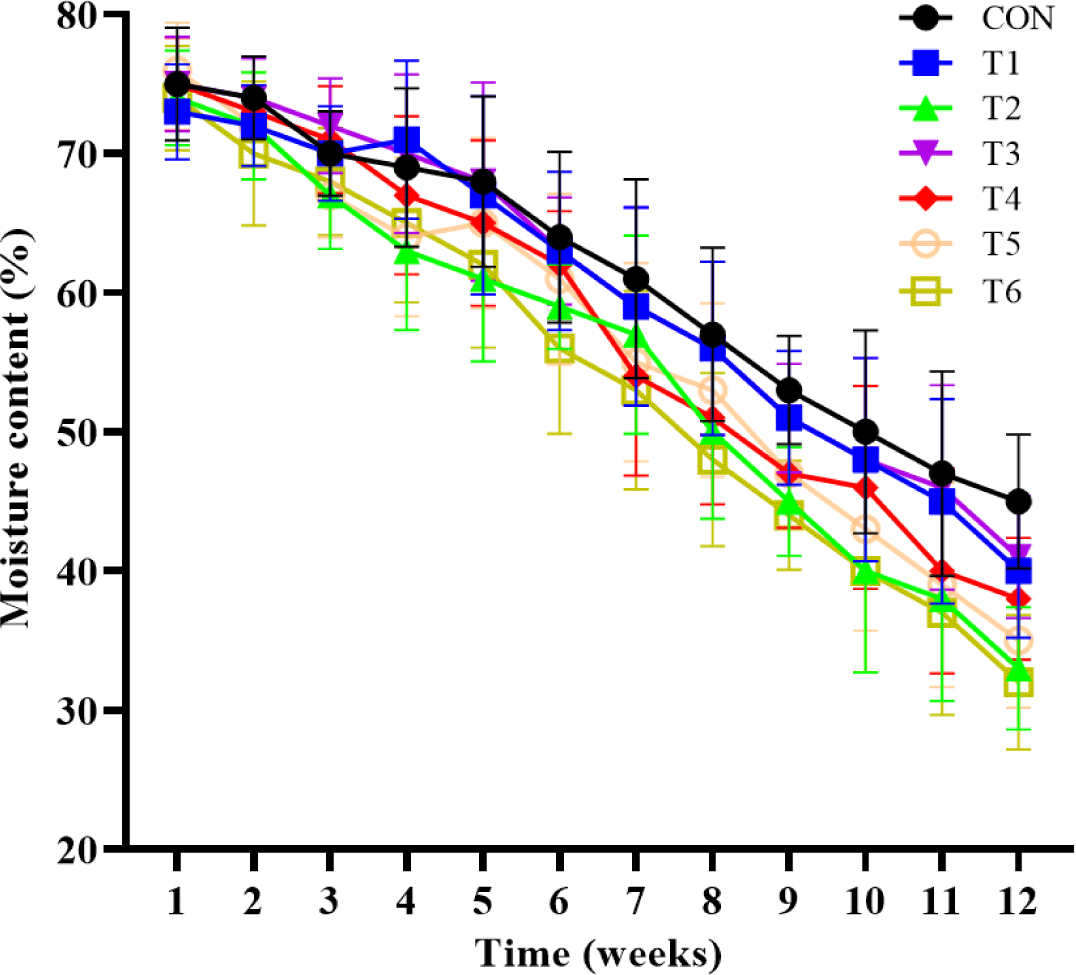
The effects of supplemental probiotics on MC of compost are presented in (Fig. 3. Supplementation of probiotic groups was only numerically decreasing (p > 0.05) MC compared to the non-supplementation group for 6 to 12 weeks.
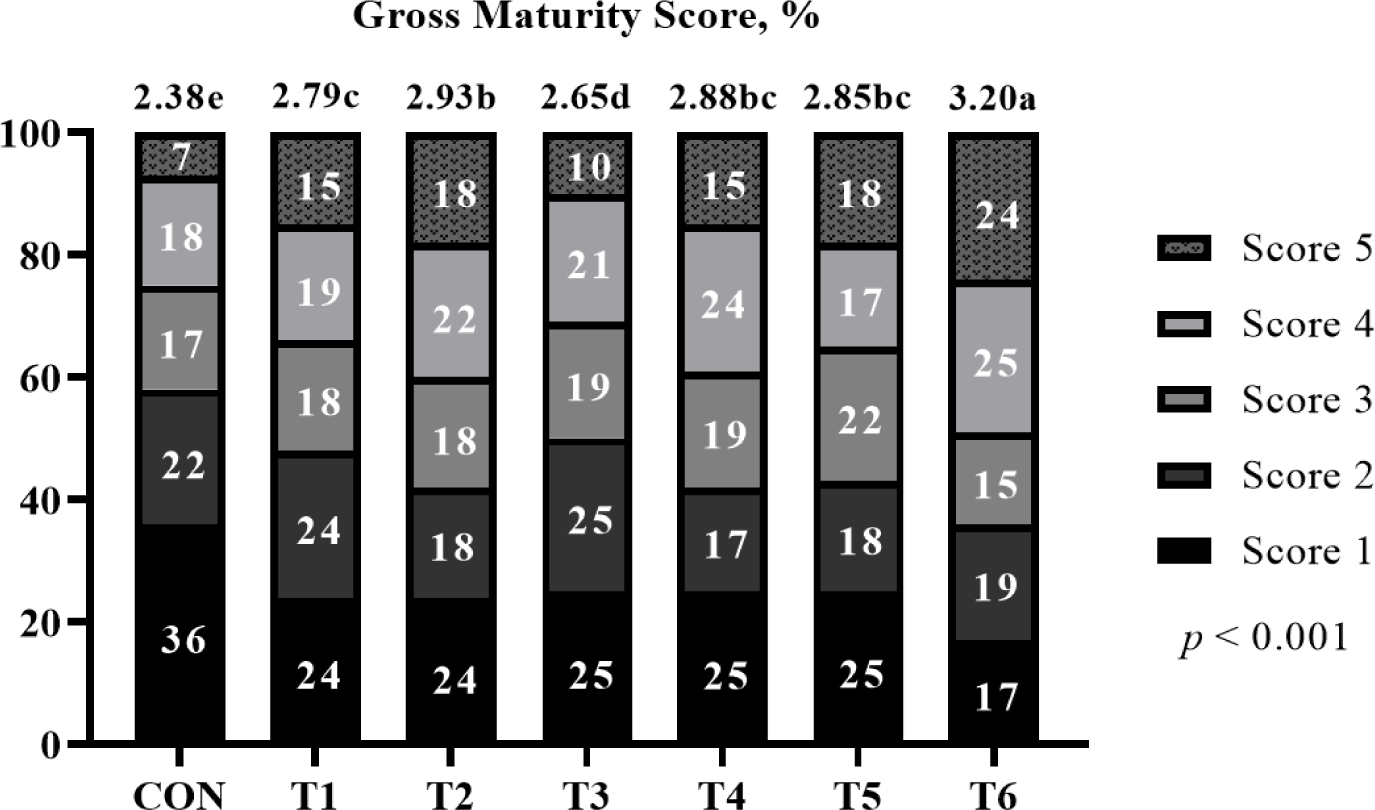
The effects of supplemental probiotics on gas emissions of compost are presented in Table 7. There was no significant difference (p > 0.05) in H2S and CH3SH emissions of compost during the overall measurement period among treatments. Although there was no significant difference in NH3 emission at 8 and 12 weeks among treatments, T6 showed significantly lower (p < 0.05) NH3 emissions than other groups at 1 week and 4 weeks, respectively.
CON, normal compost without probiotics; T1, spray Bacillus subtilis 10 g per 3.306 m2; T2, spray Bacillus subtilis 40 g per 3.306 m2; T3, spray Saccharomyces cerevisiae 10 g per 3.306 m2; T4, spray Saccharomyces cerevisiae 40 g per 3.306 m2; T5, spray (Bacillus subtilis 5 g + Saccharomyces cerevisiae 5 g) per 3.306 m2; T6, spray (Bacillus subtilis 20g + Saccharomyces cerevisiae 20 g) per 3.306 m2.
DISCUSSION
In addition to promoting the growth of beneficial bacteria, probiotics may also produce microbicidal substances that have effects against harmful microbes and gastrointestinal pathogens [16,17]. Furthermore, probiotics can improve growth performance by improving digestion, absorption, and uptake of nutrients in pigs [18]. Especially, Bacillus spp. can produce various digestive enzymes to degrade complex carbohydrates in feed and improve feed utilization [19]. Previous studies have indicated that supplementation of B. subtilis and B. licheniformis can increase ADFI and ADG [20] and decrease FCR [21] in pigs. The addition of Lactobacillus acidophilus, S. cerevisiae, and B. subtilis can also increase ADG [22] in pigs. Likewise, the results of this study revealed that the inclusion of complex probiotics such as B. subtilis and S. cerevisiae in the diets of growing-finishing pigs increased ADG and ADFI, while decreasing FCR. These study results agree with previous studies showing that complex probiotics have enhanced benefits in the gastrointestinal tract by integrating effects of different strains compared with a single probiotic [23].
The improved growth performance after adding probiotics might be related to enhanced nutrient digestibility by improving the gastrointestinal tract [24]. The mechanism of probiotics involves production of antimicrobials that can affect the composition and function of microbial communities, thus promoting overall gut health [25]. Previous studies have indicated that supplementation of complex probiotics (B. subtilis, Clostridium butyricum, B. liceniformis, and B. coagulans) can improve DM and nitrogen digestibility in growing-finishing pigs that addition of complex probiotics (B. subtilis and S. cerevisiae) can improve DM and GE digestibility in growing pigs [26,27]. Similarly, in this study, dietary addition of probiotics to pigs improved DM digestibility in the grower phase. However, another study has suggested that Supplements containing complex probiotics (B. subtilis, B. licheniformis, and S. cerevisiae) has no effect on nutrient digestibility in growing pigs [28]. Such inconsistent results on nutrient digestibility might be due to different probiotic species and dose levels.
In the current study, there was no significant difference in blood profile including WBC, RBC, creatinine, or total protein after supplementing probiotics to diets. However, there was a significant decrease in BUN concentration in groups supplemented with probiotics. BUN concentration might be used as a method for quantifying nitrogen utilization in livestock [29]. In addition, Otsuka et al. [30] have reported that increased BUN concentration is associated with an increase in feed intake. However, another study has revealed that high concentrations of BUN-impaired kidneys are harmful to pigs [31]. Probiotics can increase the efficiency of nitrogen utilization, improve nitrogen utilization, and increase BUN concentrations in pigs [32,33]. On the other hand, other studies have demonstrated that supplementation of probiotics has no effect on blood profiles of growing pigs [24,34]. These results were probably due to feed intake time or amount and gender differences.
Immature manure can generate odorous gas and cause civil complaints in nearby livestock facilities [35]. Its solutions include reducing nitrogen excretion in urine and feces and supplying feed additives to improve gastrointestinal microbial manipulation [18]. Scheuermann [36] has reported that supplementation of Lactobacillus in growing pigs can increase nitrogen retention and reduce nitrogen content in manure. In addition, Ramons et al. [37] have revealed that a reduction of nitrogen content can accelerate maturity period. Similarly, the present study showed that manure composting of dietary supplementation probiotics pigs accelerated the maturity period. These results might reduce BUN to enhance pig intestinal N retention. However, there have been few studies that have measured the maturity of manure excreted after feeding probiotics to pigs, so more research is needed.
High levels of noxious gases such as NH3, volatile sulfur, and volatile organic compounds can negatively affect animal health and performance. They, not only affect the health of workers but also cause environmental pollution [18]. Volatile sulfur-degrading properties of Bacillus spp. and increased absorption of nutrients in the gut by S. cerevisiae can reduce the substrate for microbial fermentation and decrease emissions of these gases [38,39]. Prior studies have reported that addition of Bacillus-based can reduce H2S emissions in growing pigs [24] and sows [40]. At the end of the experiment, H2S emissions were reduced in groups supplemented with probiotics. Supplementation of complex probiotics significantly decreased H2S from 4 weeks. This indicates that Bacillus-based complex probiotics might have potential to reduce gas emissions in pigs and improve air quality of swine farms efficiently with positive effects on pigs.
E. coli and Lactobacillus are representative intestinal pathogens and beneficial bacteria, respectively. Moreover, these bacteria are associated with gastrointestinal conditions, health status, and immune system [41]. Prior studies have shown that S. cerevisiae can decrease the level of potential pathogens in the intestinal lumen and generate antibacterial substances and that Bacillus can generate some effective enzymes (such as α-amylase, α-galactosidase, β-glucanase, β-mannanase, cellulase) to improve the intestinal condition [42,43]. In this study, adding complex probiotics increased Lactobacillus but decreased E. coli. Similarly, previous studies have reported that supplementation of probiotics can increase the counts of gastrointestinal lactobacillus but decrease the counts of E. coli [26,44]. The present result was consistent with Balasubramanian et al. [26] showing that continuous feeding of probiotics could maintain beneficial intestine microbiota by generating organic acids and hydrogen peroxide, thereby preventing pathogenic bacteria activation into the intestine and excreting antagonistic activity.
In compost, there are beneficial microorganisms that take charge of regular composting process and potentially harmful microorganisms for humans and the environment. These deactivations of harmful microorganisms and beneficial microbiome development are important goals of composting [45]. In addition, previous studies have reported that providing sufficient quantity of probiotics as beneficial microorganisms could enhance microbial enzyme activity and offset effects of pathogenic microorganisms [46,47].
In addition to microorganisms, factors that affect composting include porosity, aeration, moisture, and temperature [48]. Especially, low values of MC, an important environmental parameter during composting, can cause premature dehydration known to arrest biological processes, resulting in biologically unstable compost, while high values of MC will halt composting activity due to creation of anaerobic conditions caused by water logging [49]. Besides, moisture is related to heat capacity in compost [50]. It can influence metabolic activities of probiotics [51]. Lee et al. [52] have reported that microorganisms produce heat during enzymatic catabolism of substrates and synthesis of cell material. Therefore, we hypothesize that reducing MC during composting due to microorganisms could generate heat as they decompose organic material. However, in this study, single and complex probiotics (B. subtilis, S. cerevisiae) supplementation only numerically decreased MC compared to the non-supplemented group. Since no study has reported the relationship between probiotics and MC of compost, further research is needed.
Normal composting involving aerobic decomposition proceeds with the following phases: (i) fermentation, (ii) acid formation, (iii) thermophilic activity, and (iv) temperature decline [53]. Shortening the thermophilic activity phase during the degradation phase can delay the maturity period [54]. Prior studies have suggested that adding B. subtilis can prolong the thermophilic phase by increasing high-temperature-resistant bacteria [55]. Xu and Li. [56] have shown that inoculating B. subtilis, B. licheniformis, Phanerochaete chrysosporium, Trichoderma koningii, and S. cerevisiae into compost can promote compost maturity. Results of this study agree with those of previous studies showing that supplementation of B. subtilis and S. cerevisiae can reduce the maturation time of composting [57]. For this reason, complex probiotics such as B. subtilis can be used to accelerate the maturation.
Accelerating compost mature period can improve several problems such as greenhouse gas emissions, including loss of nitrogen via NH3 volatilization, and leaking of inorganic/organic pollutants from compost substrates [58]. In general, the genus Bacillus grows by assimilating ammonium nitrogen during composting, which causes NH3 emissions reduction [59]. A prior study has suggested that supplementation of Bacillus-based probiotics can reduce emissions of gases such as NH3, H2S, CH3COOH, CO2, and CH3SH [60]. In addition, supplementation of S. cerevisiae can reduce NH3 by 10.2% and amine gas by 45.5% in swine manure [61]. In this study, supplementation of probiotics decreased NH3 emissions compared with non-supplementation. However, other odorous gas emissions (CH3SH and H2S) were not affected by supplementation of probiotics. These inconsistent results are attributed to temperature and humidity.
CONCLUSION
This study indicates that supplementation of probiotics at the complex probiotics are more improved on growth performance, nutrient digestibility, blood profile, compost maturity, gas emissions, and fecal microflora in pigs and on MC, compost maturity, and gas emissions in compost than single and non-supplementation. Therefore, these results revealed that complex probiotics (S. cerevisiae and B. subtilis) had positive effects in pigs and compost, respectively. However, supplementation of complex probiotics in compost rarely investigates so more studies are needed.