INTRODUCTION
Hanwoo cattle, a native breed in Korea, are highly valued in the beef industry of Korea because of their unique palatability and high intramuscular fat content [1]. Compared to beef from other countries, Hanwoo beef has a lower connective tissue content and distinct flavor [2]. Despite the higher price, Korean consumers prefer Hanwoo beef over imported beef because of its freshness and Hanwoo beef is considered the highest quality meat in Korea [3].
Hanwoo beef is available in three basic sex categories in the Korean beef market: steers, cows, and bulls [4]. On the other hand, the proportion of each sex type that is slaughtered for meat production varies from year to year. For example, in 2020, cows comprised 46.2% (410,021 heads) of the total cattle slaughtered for meat, while bulls or steers comprised 53.8%. These proportions were 48.0% and 52.0%, respectively, in 2022 [5]. Korean consumers prefer high-quality grades of meat and Hanwoo steers (41.9%) outnumbered cows (18.2%) in terms of the proportion of animals with high grades (1++ grades) [6].
The carcass and meat quality attributes of cattle are influenced by gender. Previous study has shown that the tenderness of specific animal muscles varies according to their slaughter age and gender [7]. Older animals typically produce tougher meat than younger ones, while gender plays a role in muscle growth, meat color, lipid deposition, and other quality traits [8]. Meat from steers and heifers typically shows more marbling than meat from bulls, making them more tender and superior in terms of eating quality [9].
An increasing number of Korean consumers are becoming concerned over the high-fat content of Hanwoo beef, and the potential health risks of obesity and fat-mediated diseases [10]. These worries are supported by numerous health authorities and nutritionists’ long-standing claims that saturated fat in meat has detrimental effects on health. Considering consumer preferences, the Korean meat industry has recently introduced Hanwoo heifer meat as an alternative source of beef. Various studies have consistently demonstrated that heifer beef has higher eating quality traits, physicochemical composition and fatty acid profile [11,12]. The average price of heifer meat exceeds that of steer and bull meat of the same quality due to consumer preferences [6]. However, there is a lack of scientifically validated data on the quality of heifer meat from Hanwoo cattle. Furthermore, a comprehensive comparative study of the quality traits of Hanwoo meat obtained from cows, heifers, and steers at their respective slaughter ages has yet to be conducted. Consequently, there is a need for research to determine if there are any differences in physicochemical and quality traits among these types of beef. Therefore, this study aimed the scientific evidence regarding the disparities in the physicochemical properties, muscle fiber structure, fatty acid compositon and metabolites of Hanwoo steer, heifer, and cow striploins.
MATERIALS AND METHODS
Striploin muscles from Hanwoo steers (30–32 months old, n=3), heifers (24 ≤ months old, n = 3), and cows (≥ 38 months old, n = 3) were collected from Damyang, Jeollanam-do, Korea. The meat samples were vacuum-packaged after collection, transported to the laboratory, and then aged at 4°C for seven days. Subsequently, the meat was stored at −18°C until analysis. Before the experiment, the samples were defrosted for 24 h at 4°C.
The amount of moisture was estimated using the AOAC [13] technique. To begin, 3 g of sample were placed in a pre-weighed aluminum dish and dry at 104°C in a dry oven (WON-155,DAIHAN, Wonju, Korea) for 24 h. The dishes were weighed again after drying and cooling in a desiccator. The percentage difference between the weights before and after drying was used to compute the moisture content. The quantity of crude protein was calculated using the Kjeldahl technique (K-370, Buchi AG, Flawil, Switzerland) in accordance with the recommendations of AOAC [13] guidelines. With a little modification outlined by Mopuri et al. [14], the Folch approach was used to determine the fat content by following equation (1).
Hanwoo striploins were examined using Gas Chromatography (GC) in accordance with the AOAC 994.10 method with a minor modification, as stated by Dinh et al. [15]. Initially, a 1 g sample was placed in a 125 mL boiling flask and saponified with 2 mL of a KOH solution (50%) and 8 mL of 95% ethanol for 15 min. After cooling, 10 mL of toluene was added and mixed, then transferred to a separating funnel. The water layer was eliminated while toluene portion was washed using 1.0 N and 0.5 N KOH, followed by three washes with distilled water (DW). 5 g of Na2SO4 was added with washed toluene in a 25 mL test tube to eliminate moisture from the toluene. Then, 0.5 mL of internal standard (5α-cholestane in toluene, Sigma-Aldrich, St. Louis, MO, USA) added to 0.5 mL of toluene, resulting solution was subjected to the GC machine (HP 5890, Agilent Technologies, Santa Clara, CA, USA) with HP-5 column coupled with flame ionization detector (FID) detector. The cholesterol standards (Sigma-Aldrich) were prepared in toluene at concentrations of 0.099, 0.0495, 0.0099, 0.00495, and 0.002475 mg/mL.
2 g of sample was homogenized with 18 mL of DW before being filtered through Whatman No.1 filter paper (Whatman Grade-1-1001-110, 1001-125, GE Healthcare Life Sciences, Shanghai, China), and the pH was measured at ambient temperature by a calibrated pH meter (Seven ExcellenceTM, METTLER TOLEDO, Greifensee, Switzerland). Regarding color measurement, sliced meat was bloomed for 30 min, and then the CIE approach was used reported by Yim et al. [16]. After calibration, a Minolta chromameter (Model CR-410, Minolta, Osaka, Japan) was used to measure the surface color of the striploin.
WHC was determined with a slight modification of the method described by Kristensen & Purslow [17]. Initially, 5 g of the ground sample was put on 6×6 cm cotton paper (Aritaum 1/2 slim cotton pads 160EA, ARITAUM, Seoul, Korea) in a 50 mL conical tube (SPL Life sciences, SPL-50050, Pocheon, Korea), then centrifuged for 10 mins at 168×g. In order to measure the centrifugation loss, the sample weight before and after centrifugation was recorded, and equation (2) was used to compute the WHC.
Grilling is a preferred method for assessing cooking loss in meat products because it can cook the outer layers while retaining interior moisture quickly, creating a flavorful sear and promoting the Maillard reaction. Approximately 15 g of ground meat was shaped into a ball and then grilled on an electric grill (Nova EMG-533, 1,400 W, Evergreen Enterprise, Yongin, Korea) at 180°C–200°C for about 1 min until it reached an internal temperature at 72°C [18]. Then cooled for 10 min at ambient temperature before weighing. The cooking loss was calculated using equation (3).
A Warner-Bratzler machine (LC-500N0732, Ametek, Leicester, Leicestershire, UK) was used to determine the force of a meat sample. First, a 10 mm thick slice of meat was cut and grilled on an electric grill at approximately 180°C–200°C for 1 min. Cooled for 10 min then sliced with the dimension of 10 × 20 × 10 mm, parallel to the direction of muscle fiber. The Warner Bratzler shear force (WBSF) values were measured using a V-shaped shear blade to cut the meat cores, and the force required to make the cuts was measured in kilogram-force (kgf). The cell load was 45 N, and the crosshead speed was configured at 600 mm/min.
Soluble and insoluble collagen in the samples were measured using an indirect hydroxyproline assay method described by Kim et al. [19] with some minor changes. Briefly, 4 g of ground meat sample was initially pre-treated with 20 mL of 0.1 M sodium phosphate buffer (NaH2PO4 and Na2HPO4, pH = 7.0) to break down cross-links of collagens with connective tissues. To enhance the process, the mixture was heated at 70°C (water bath) for 30 minutes, with five intermittent shaking cycles. Afterwards, it was immediately chilled with ice water for five minutes, then centrifuged at 10,000×g for 15 minutes or at 4,000 rpm for 30 minutes. In order to measure the soluble and insoluble collagen, supernatant and precipitate were separated. 10 mL of supernatant (for soluble collagen) and precipitant were combined with 10 mL of deionized water (for insoluble collagen) were hydrolyzed for 6 hours at 100°C with 15 mL of 7 M H2SO4 with shaking every 30 minutes to produce free hydroxyproline. Following hydrolysis, the samples were chilled in ice water for 5 minutes before being combined with 1 mL of 1.41% chloramine-T hydrate oxidant solution that oxidized hydroxyproline to pyrrole-2-carboxylate. Following this, each sample was vortexed after adding 1 mL of Ehrlich’s color reagent, 17.5 mL of 60% sulfuric acid, and 32.5 mL of 2-propanol. During this step, pyrolle-2-carboxylate reacts with para-Dimethylaminobenzaldehyde (DMAB) to produce a chromophore. To enhance the reaction rate incubated for 20 min at 65°C (water bath), and then cooled by water for 3. The samples were centrifuged at 3,000×g for 15 mins, and an ultraviolet/visible (UV/Vis) spectrophotometer (OPTIZENTM POP V-VIS Spectrophotometer, K-Lab, Gunpo, Korea) was used to measure the absorbance at 558 nm. The blank solution was made by combining phosphate buffer and 7 M sulfuric acid (2:1.5), and 2 mL of it was mixed with 1 mL of the oxidant solution and 1.0 mL of the coloring reagent. The hydroxyproline levels were determined using a standard curve of 0, 0.6, 1.2, 1.8, 2.4, and 4.8 μg hydroxyproline/mL of H2O. The levels were multiplied by 7.52 and 7.25 for the soluble and insoluble collagen content of the samples, respectively. The soluble (4) and insoluble (5) collagen levels were calculated using the following equations.
According to the methods described by Yoon et al. [20], CS content was assessed. The test solution was made by adding 100 mL of DW in 0.3 g of the sample and mixing thoroughly. Then 4 mL of this solution was diluted to 20 mL with DW, and filtered using Whatman No. 4 filter paper. Then, ice-cold water was used to chill a test tube holding 5 mL of a 1% (w/v) sodium borate sulfuric acid solution. 1 mL aliquot of the sample solution, standard solution, and DW (control) were added carefully to the sodium borate sulfuric acid solution, mixed under cooling conditions, and heated in a water bath (90°C, 10 min), followed by immediate cooling in ice water. The test tube was then filled with 0.2 mL of 0.125% (w/v) carbazole solution in absolute ethanol, stirred, and heated at 90°C for 15 minutes before cooling to room temperature. Finally, glucuronolactone was used to create a standard curve, and 530 nm absorbance was measured to measure CS content by following equation (6).
Where, A = Absorbance of the sample, B = Absorbance of standard, C = γ -glucuronolactone, D = Sample weight, 1.1023 = molecular weight (MW) of glucuronic acid / MW of γ-glucuronolactone, and 2.593 = MW of CS / MW of glucuronic acid.
The samples were divided into dimensions of 1 × 1 × 0.2 cm and were affixed to aluminum stubs using double-sided carbon tape. The meat cuts were pre-fixed and post-fixed with 2.5% glutaraldehyde and 1% osmium tetroxide, respectively, at 4°C for 4 and 2 h under the dark condition. The samples were then immersed in phosphate-buffered saline (PBS) and washed using ethanol gradients ranging from 30% to 99%, with air drying allowed in between each wash. Then, 10 mA of platinum coating was applied for 1 minute. Using a SEM (JSM-7500F, Hitachi, Tokyo, Japan), the samples were photographed.
The technique outlined by O’Fallon et al. [21] was used to determine the fatty acid composition of Hanwoo striploin. Initially, 0.7 mL of 10 N KOH and 6.3 mL of methanol were added to 1 g of the sample, which was then heated at 55°C (water bath) for 1.5 h while being frequently shaken. The mixture was cooled, 0.58 mL of 24 N H2SO4 was added, and it was then heated for 1.5 hours under the same circumstances. 3 mL of hexane was added to the mixture once it had cooled, and it was vortexed for two minutes before being centrifuged at 1,500×g. Upper clear fatty acid methyl esters were run by GC (Agilent 7890, Agilent Technologies). The amount of fatty acids was represented as a percentage of the total number of fatty acids tested.
The Kim et al. approach was used to extract and analyze the metabolic profile [22]. Initially, 20 mL of 0.6 M perchloric acid was used to extract 5 g of material, and the resultant homogenate was then centrifuged at 3,500×g for 20 mins. The supernatant was filtered and adjusted pH to 7.0 then lyophilized. Then diluted with D2O and 1 mM 3-(trimethylsilyl)propionic-2,2,3,3-d4 acid in 20 mM phosphate buffer (pH 7.4) prior to nuclear magnetic resonance (NMR) (Bruker 850) examination. 3-(trimethylsilyl)propionic-2,2,3,3-d4 acid (TSP) was as standard and spectra were analysed by Topspin 4.0.8.
The data were analyzed using SPSS version 20 statistical software. A homogeneity of variances test was performed to conduct the appropriate statistical test. Robust tests and one-way ANOVA were used to analyze the effects of gender on meat quality attributes. The mean values and SD of the results are presented. The Tukey’s honest significant difference (HSD), a least significant difference (LSD), and the Games-Howell test were used for the post hoc analysis. The significant difference between the means was defined as P-value p < 0.05.
RESULTS
Fig. 1 illustrates the composition of moisture, fat, protein, and cholesterol content in striploin from the Hanwoo steer, heifer, and cow. The findings show that there are no appreciable variations in the moisture and protein content (p > 0.05). Contrarily, steer striploin had a much greater fat level (14.61%) than cow striploin (10.66%), and it also had a higher cholesterol content (41.92%) than cow (34.66%). Heifers contained 12.05% fat and 37.02% cholesterol, respectively.
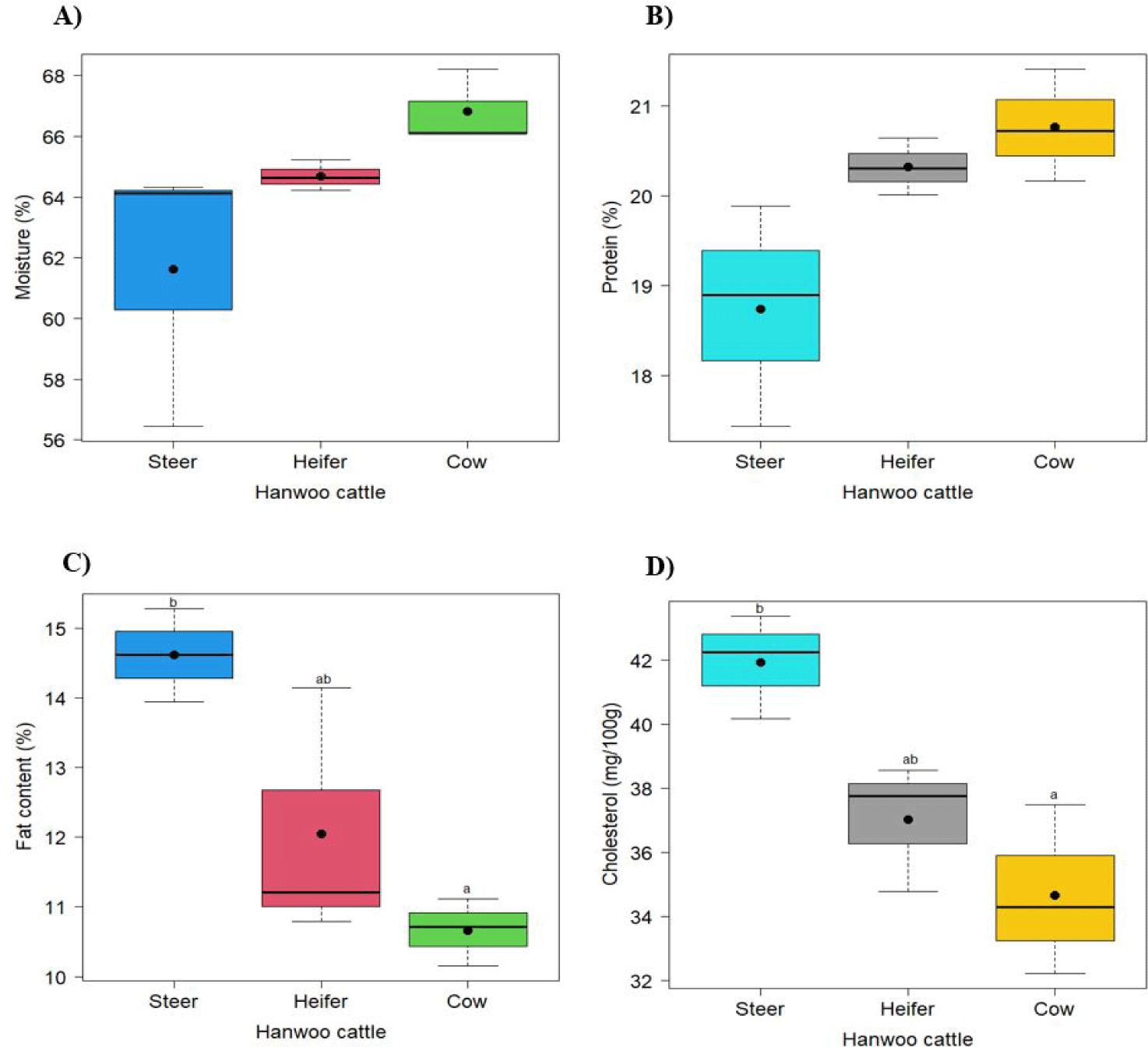
The pH and WHC were similar in the meat groups (Table 1). On the other hand, cow striploin had the highest pH (5.63) and WHC (79.78%) compared to steer and heifer. In terms of the shear force (kgf), the cow had the lowest value (2.50), while the steer (4.09) and heifer (3.45) had higher values. The lightness, redness, and yellowness showed no significant differences between steer and heifer meat. The cow had lower values than the steer and heifer. The highest cooking loss was observed in steer striploin (27.81%), while cow (20.04%) and heifer (24.41%) had lower values. The soluble collagen (mg/100 g) was significantly higher in heifer (3.63) than in steer (2.54) and cow (2.47). In contrast, cow had higher insoluble collagen levels than steer and heifer. The chondroitin content in steer (1.19%) was significantly higher than in the heifer (0.22%) and cow (0.29%). Furthermore, the muscle fiber area was observed to be significantly greater in steer (1,545.23 μm2) compared to the heifer, which had the lowest value (998.73 μm2) (Fig. 2).
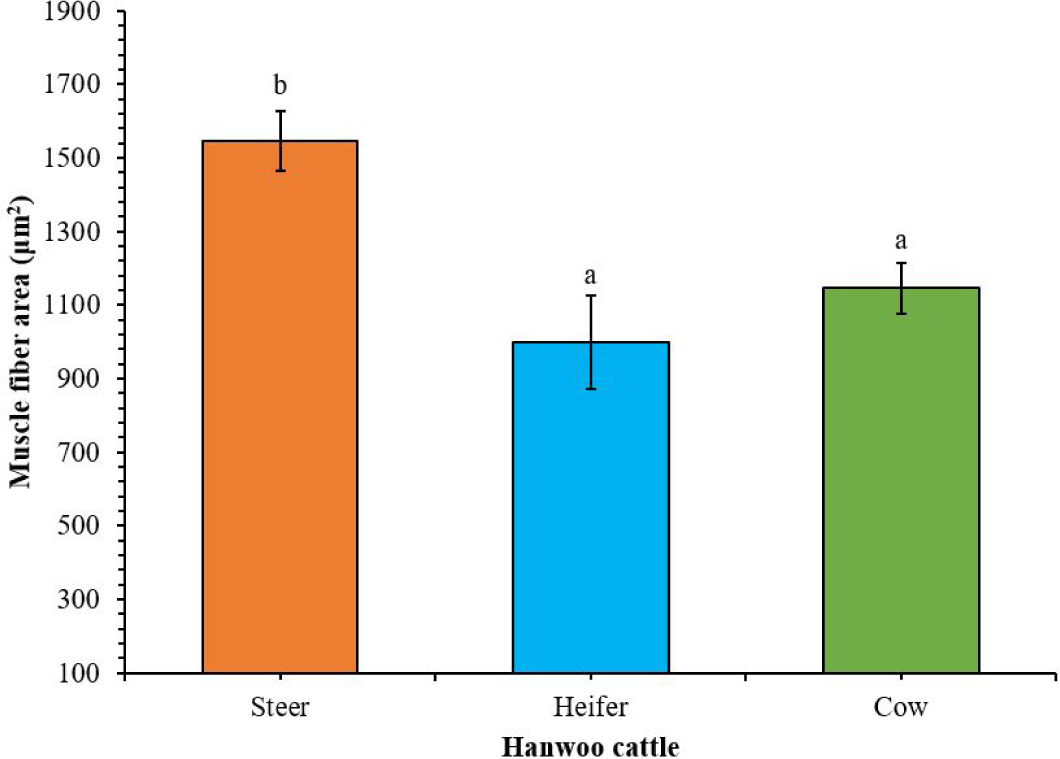
Hanwoo striploin fatty acid content is shown in Table 2. The research found 14 fatty acids in the striploin, the three most abundant of which are oleic acid, palmitic acid, and stearic acid. The overall UFA and MUFA content were higher (p < 0.05) in cow meat than in the other genders, but the saturated fatty acid (SFA) concentration was similar across all meat varieties. Additionally, cow striploin had a considerably greater total unsaturated fatty acid (UFA) content than steer and heifer (p = 0.013). In terms of lipid indices, the atherogenic index (AI) in cow striploin was substantially lower than in steer and heifer (p = 0.045).
Table 3 displays the findings of the NMR analysis conducted on Hanwoo striploin, which discovered a number of metabolites, including amino acids, bioactive substances, products linked to energy metabolism, products connected to nucleotides, and other components. When compared to steer, the levels of the amino acid metabolites glycine, isoleucine, methionine, phenylalanine, tyrosine, valine, and dimethylglycine (DMG) were considerably greater in cows (p < 0.05). Similar to this, cows had greater levels of the bioactive metabolite carnitine (92.42 mg/100 g) than steers (55.95 mg/100 g) and heifers (78.38 mg/100 g). However, heifers (178.78 mg/100 g) had much greater levels of carnosine than steers (42.56 mg/100 g) or cows (41.13 mg/100 g). Inosine was found in considerably higher concentrations in the heifer (23.23 mg/100 g) and cow (25.95 mg/100 g) than in the steer (14.07 mg/100 g).
Partial least squares-discriminant analysis (PLS-DA) was used to compare the metabolite differences between the three types of striploin groups (Fig. 3A). The analysis showed a high cumulative explained variation (R2 = 0.93) and predictive ability (Q2 = 0.70), suggesting that the dataset effectively differentiated striploin groups and could potentially be used to predict gender using the quantified metabolomic information. Furthermore, the variable importance in projection (VIP) score highlighted 13 metabolites (DMG, phenylalanine, carnitine, glycine, methionine, inosine, tyrosine, niacinamide, isoleucine, lactate, leucine, valine, and creatine) with a concentration exceeding 1 mg/100 g in meat, highlighting their importance in discriminating between the different meat groups in PLS-DA analysis (Fig. 3B). The dendrogram of heatmap analysis also showed that heifer and cow striploin were distinct from steer regarding their metabolite contents (Fig. 3C).
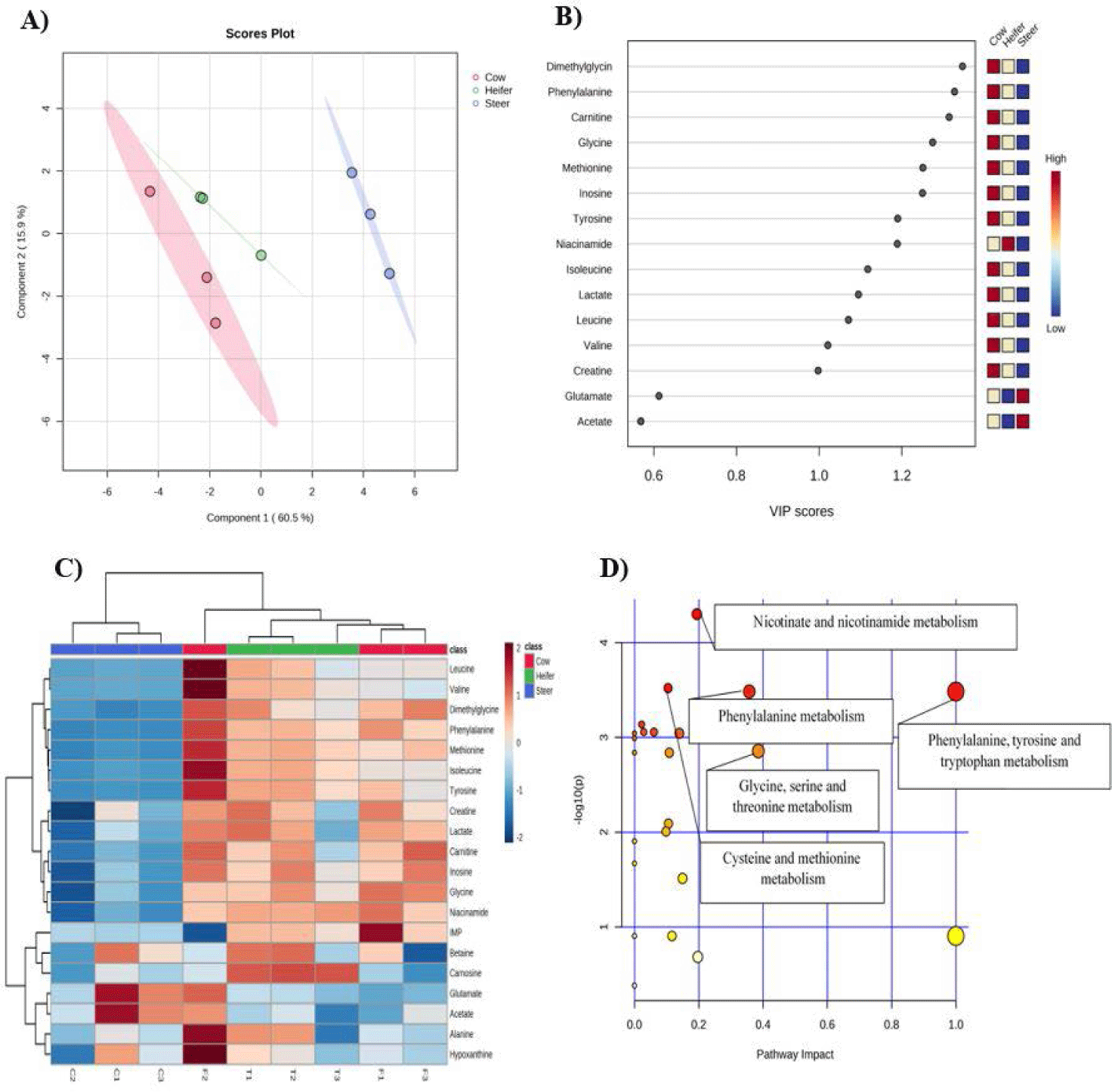
Furthermore, a Kyoto Encyclopedia of Genes and Genomes (KEGG) library-based pathway analysis was conducted to determine the interacting relationship between the metabolomic pathways (Fig. 3D). Pathway analyses with zero points-of-impact scores were disregarded because they did not affect the metabolomic differences. Five pathways were selected based on the VIP scores and pathway analysis: phenylalanine, tyrosine, and tryptophan metabolism; nicotinate and nicotinamide metabolism; phenylalanine metabolism; glycine, serine, and threonine metabolism; and cysteine and methionine metabolism.
DISCUSSION
The moisture, protein, fat, and cholesterol content of the Hanwoo striploin from steer, heifer, and cow were measured. There are no differences in moisture and protein contents among the groups, which could be attributed to using the same part and grade of meat. Cho et al. [4] reported, similar results for moisture and protein in Hanwoo steer and cow Longissimus thoracis (LT) muscles. On the other hand, their findings revealed a higher fat content in both types of meat compared to the present study. In contrast, Hanwoo, Holstein, and Danish Friesian cows exhibited lower fat content than the present findings [2]. Numerous factors, including genetics, diet, age, gender, and muscle location across the studies, can influence the variation in fat content. In the present study, the cholesterol content was significantly higher in steer than in cow. The cholesterol content was correlated with the fat content because meat with a higher fat content tends to have higher cholesterol values [23]. Elevated cholesterol levels have been associated with metabolic diseases, such as stroke, heart disease, atherosclerosis, and hypertension [24].
Although gender and slaughter age showed no influence on the pH and WHC of striploin (Table 1), the study findings showed that cow exhibited a higher WHC (79.78%) than the previous study (63.52%) by Cho et al. [4]. The WHC is associated with tenderness and flavor, which correlates with the pH levels, intramuscular fat, and cooking loss of meat [25]. The meat shear force value is important because it provides valuable information about the tenderness of the meat, influencing consumer preference, cooking methods, and overall palatability [26]. In the present study, cow showed a significantly lower shear force among the meat types, possibly due to the contractile state of myofibrillar and proteolysis during aging. In the present study, steer striploin has a comparatively high fat content, and the muscle fiber area was larger. A previous study showed that meat with high muscle fiber area content tends to have high shear force values [27]. This is because broader or wider muscle fibers require more force to cut, resulting in an increased WBSF value.
Gender status plays a crucial role in the physicochemical quality and color characteristics of beef muscles [28]. In this study, the L* value was significantly higher in steer and heifer striploin, possibly due to the higher fat content. Joo et al. [27] also reported similar findings on Belgian Blue bulls and Hanwoo steer, respectively. In this study, the redness and yellowness of the meat were significantly higher in both steer and heifer. This variation in color features could be related to the age of the animals slaughtered, as steers (30–32 months) and heifers (24 months) were slaughtered at a younger age than cows (≥–38 months). On the other hand, a contrasting result was reported by Mueller et al. [29], who reported no significant variation in color traits, including lightness and redness, between meat obtained from steers and heifers. Cooking loss is a significant physicochemical property of meat that plays a role in ensuring meat tenderness and optimizing meat quality. The research findings suggested that cooking loss was notably higher in steer meat compared to heifer meat, while cow meat demonstrated the lowest value. This observation is consistent with the WHC of the meat, where cow meat exhibited the highest WHC, while steer meat had the lowest. The differences in cooking loss among various beef types can be attributed to the chemical composition and marbling variations. This aligns with Ozawa et al. [30], who reported that Japanese black steer meat, which possesses higher levels of intramuscular fat, displayed less cooking loss.
Collagen and chondroitin offer a range of health benefits, including maintaining healthy bones, skin, joints, hair, and nails. Both also exhibit anti-inflammatory properties and aid in wound healing [31]. According to Torrescano et al. [32], collagen is associated with meat tenderness, one of the major constituents of connective tissue. Collagen fibrils and bundles form intricate structures in the endomysium, surrounding the individual muscle fibers like a fine mesh. In the perimysium, collagen fibrils and bundles develop into larger bundles with striations that run longitudinally, circularly, or obliquely around groups of muscle fibers [33]. When collagen is subjected to heat, it transforms into gelatin, increasing collagen solubility and improving meat tenderness. The soluble collagen content in meat had a negative correlation with shear force, as reported by Chang et al. [34]. Hopkins et al. [35] reported different results; they did not observe any correlation between sensory tenderness, shear force, and collagen concentration in lamb meat. In the present study, the chondroitin content in steer was significantly higher than in heifer and cow striploin. This difference might be due to variations in the age at slaughter and gender differences. CS is an effective treatment for osteoarthritis. Clinical study has shown that orally administered CS can enhance joint function and alleviate discomfort in patients with osteoarthritis [36].
The meat flavor is influenced significantly by the fatty acid composition in muscle tissues. This study showed a significant difference in the amount of elaidic acid among the groups, with the highest and lowest levels in steer and heifer meat, respectively. This outcome is consistent with Lee et al. [37], who reported that Hanwoo steer meat has a higher elaidic acid content than meat from female cattle. Elaidic acid is produced in ruminant meat and milk by biohydrogenation in the rumen, and this natural trans-fatty acid has weaker impacts on human health [38]. However, a previous study has reported that elaidic acid is positively associated with an increased risk of cancer and a higher risk of depression [39]. A significant difference in oleic acid and MUFA was noted among the striploin types (p < 0.05). In the present study, the cow had the highest oleic acid and MUFA, and the steer had the lowest amount. According to Hwang and Joo [40], oleic acid and MUFA are positively associated with meat flavor and savory taste, whereas polyunsaturated fatty acids (PUFAs) are negatively correlated. The taste and flavor of meat are significant qualities that increase consumers’ preferences. Therefore, a higher oleic acid concentration can enhance the taste of meat, making it more enjoyable for consumers. The findings revealed that among the groups, oleic acid was the most prevalent fatty acid, followed by palmitic acid. This observation aligns with the results reported by Jayasena et al. [41] and Cho et al. [4].
Interestingly, the eicosadienoic acid (20 : 2) levels were significantly higher in steer than in cow. Eicosadienoic acid affects the metabolism of PUFAs and hamper the response of macrophages to inflammatory stimulation [42]. Cow striploin exhibits a significantly higher quantity of UFAs compared to the other two groups (p < 0.05), which have a beneficial influence on better health. On the other hand, it contains a lower amount of SFAs. Previous studies suggested that SFAs contribute primarily to hyperlipidemia and hypercholesterolemia. These disorders, in turn, have been associated with the development of diabetes and cardiovascular disease [43]. Consequently, the consumption of lower SFAs is recommended for better health outcomes. The AI is used as a marker to assess the impact of fats on cholesterol levels and is associated with the risk of atherosclerosis [44]. In this study, the AI was significantly lower in cow than in the other two groups (p < 0.05). Lower AI reduces plaque formation in blood vessels and prevents cardiovascular diseases [45].
Scientific evidence showed that steers have hormonal alterations that may have a direct effect on body fat accumulation and the distribution of fatty acids in muscle tissues [46]. This also reflects in our study where fatty acids in steer and heifer did not differ significantly. These results align with those of Mueller et al. [29], who similarly found no appreciable variations in most fatty acids between steer and heifer striploins. Only the beef from cows and steers showed significant differences. These findings concur with those of Jayasena et al. [41] and Lee et al. [37], who reported comparable patterns in the fatty acid content of beef muscles from Hanwoo steers and heifers. Previous study has shown that the production system, including feeding diets and regimes, significantly affects beef muscle fat deposition and fatty acid profiles [47]. Unfortunately, there was no documentation of the diets and feeding schedules for the groups in the present study.
According to Ramalingam et al. [48], meat metabolites enhance the taste, flavor, nutritional value, and potential health effects of meat. These metabolites encompass diverse molecules, such as amino acids, fatty acids, vitamins, minerals, organic acids, and other bioactive compounds. The composition of meat metabolites can differ according to various factors including the animal species, breed, diet, age, and processing methods used. Among the amino acids, only betaine was lower in cow striploin. All the other metabolites had higher levels in cow than in steer and heifer. This may be due to the higher age of cow at slaughter (≥ 38 months). In addition, this study showed that alanine was the predominant amino acid in meat, and there was no significant difference in its concentration among the groups (p > 0.05). According to Kato et al. [49], alanine influences the sweet taste of cooked meat. Cow meat had significantly higher glycine and isoleucine levels (p < 0.05). Protein synthesis and collagen production are two metabolic processes involving glycine. In addition, glycine affects the tenderness and juiciness of meat [50]. Isoleucine plays a role in the growth and intramuscular fat content. It is also used to treat obesity and diabetes [51].
Methionine, phenylalanine, tyrosine, valine and DMG are higher in steer than heifer and cow striploin. These amino acids impart a bitter taste to meat [37]. Regarding bioactive metabolites, carnosine is significantly higher in heifer meat, whereas carnitine is higher in cow meat. Muscle enzymes produce carnosine during the breakdown of proteins, and it strongly affects the flavor. Carnitine has a high correlation (r = 0.9764) with the redness color of meat and has been found in higher amounts in Hanwoo meat [52]. It is also necessary for the synthesis of energy and the metabolism of fatty acids. Its deficiency causes various illnesses, such as cirrhosis, diabetes, heart disease, starvation, and endocrine disorders [53]. In contrast to steer, cow striploin has higher creatine, a substance formed from lipid metabolism, which is consistent with Cho et al. [4]. Among the nucleotide-related metabolites, inosine was detected in higher concentrations in cow striploin and lower concentrations in steer striploin. During cooking, inosine interacts with amino acids and peptides, resulting in the formation of taste umami flavor in the meat [4]. Niacinamide, or vitamin B3, plays multiple roles in improving meat quality. It also acts as an antioxidant, flavor enhancer, and improves the WHC of meat [54].
CONCLUSION
Cow meat has a higher moisture and protein content compared to steer and heifer meat, but less fat and cholesterol. In comparison to the other two varieties of meat, cow meat has much lower values for shear force, lightness, redness, yellowness, cooking loss, and soluble collagen. In contrast to heifer and cow meat, steer meat has much more chondroitin and muscle area. In terms of the fatty acid composition, cow meat has a lower AI than steer and heifer striploin but much more UFAs and MUFAs. Furthermore, the abundance of water-soluble metabolites is higher in heifer and cow meat. It can be inferred that the quality traits of the Hanwoo striploin are significantly influenced by gender. Further extensive research is required to comprehensively compare the quality traits of Hanwoo steer, heifer, and cow meat.