INTRODUCTION
Since the birth of Dolly the Sheep, the first cloned mammal, in 1997 [1], successful cloning of dogs was reported in 2005 [2]. Over the past 15 years, canine somatic cell nuclear transfer (SCNT) technology has found diverse applications in reproductive biotechnology, including: 1) propagating specific dogs with elite working abilities [3,4], 2) preserving endangered canine species [5,6] and companion dogs [7,8], and 3) generating disease model dogs for studying disease mechanisms and therapies [9-11]. Despite its utility, dog cloning remains a formidable challenge due to difficulties in obtaining satisfactory results in in vitro oocyte maturation, estrus induction, and superovulation [12,13]. The challenges stem from the unique reproductive physiology of canines, such as ovulation at the germinal vesicle stage, mono-estrus, the hormonal environment, and the lack of reliable in vitro maturation (IVM) systems [14,15].
To overcome these obstacles, canine SCNT studies have utilized oocytes matured in vivo [16,17]. Matured oocytes are collected surgically by flushing oviducts at 72 h post-ovulation, with the timing of natural ovulation predicted based on serum progesterone concentration in the oocyte donor (ovulation occurs at 4.0–9.9 ng ⁄mL) [2]. Typically, six to 12 oocytes are collected from one female dog in natural estrus through oviduct flushing, yielding an average recovery rate of 93.8% [18]. However, not all oocytes recovered through this method undergo complete nuclear maturation, and at least 3% to a maximum of 33% of the oocytes remain immature, lacking the first polar body [19,20]. If immature oocytes can be matured in vitro, the availability of oocytes would be maximized, thereby enhancing SCNT efficiency.
Unlike other mammalian species, canine oocytes are ovulated in an immature state and are exposed to high levels of progesterone (P4) and estradiol (E2) in the bursa and oviduct. Nuclear maturation is completed in the oviduct over 48 h–72 h through exposure to P4 and E2 [14,15,21]. Various factors have been identified to influence the IVM of canine oocytes, with hormones playing an important role in the meiotic resumption in canine oocytes. The oocytes matured to the metaphase II (MII) stage significantly increased under supplementation with gonadotropins (such as follicle-stimulating hormone [FSH] and luteinizing hormone [LH]) and/or steroid hormones (such as E2 and P4) [22-26]. Interestingly, oocytes reaching the MII stage during IVM were higher in the estrus stage than in the anestrus and diestrus stage [18,22,27]. Nevertheless, the production of in vitro matured canine oocytes remains limited in quantity and quality, rendering them unsuitable for successful canine SCNT.
In this study, we hypothesize that in vivo-immature oocytes from female dogs in the estrous stage possess the sufficient ability to resume meiosis when subjected to IVM under appropriate hormonal conditions. We selected P4 and FSH as the hormones for this study, and their concentrations were determined based on previous studies [22-26], and aimed to determine the combination effect of these hormones (P4 + FSH) on the IVM of immature oocytes recovered from oviduct flushing of naturally ovulated female dogs. Additionally, we sought to evaluate the suitability of these hormonally treated oocytes as donors for canine SCNT.
MATERIALS AND METHODS
All chemicals and reagents were purchased from Sigma-Aldrich Chemical (St. Louis, MO, USA) unless otherwise specified.
The experimental animals consisted of 1 to 6 years old mixed-breed female dogs in the estrus stage, which were used as oocyte donors and surrogate mothers. The experimental procedures were approved by the Institutional Animal Care and Use Committee (IACUC) of Chungnam National University (Approval Number: CNU-01089). All dogs were individually housed in controlled temperature and ventilation conditions, provided a commercial diet once a day, and had access to water ad libitum.
Preparation of donor cell was performed as previously described [28]. The donor cell was acquired from a 16-year-old male Pomeranian through a post-mortem skin biopsy for the establishment of fibroblasts. Muscle tissues were washed three times and minced in phosphate-buffered saline ([PBS] Gibco, Thermo Fisher Scientific, Waltham, MA, USA). The tissue pieces were washed twice by centrifugation at 400×g for 5 minutes and cultured in a culture medium composed of Dulbecco’s modified Eagle’s medium ([DMEM] Gibco) supplemented with 20% (v/v) fetal bovine serum ([FBS] Gibco) and 1% penicillin-streptomycin. After being cut into small pieces, the cells were cultured at 39°C in a humidified atmosphere of 5% CO2 and 95% air. When the cells reached confluence after 5–7 days, they were harvested after treated with 0.25% trypsin-EDTA (Gibco) for 2 minutes. The established cell line was frozen in 10% DMSO in FBS and stored in liquid nitrogen at −196°C until further use. Cells from passages 5–7 were trypsinized before SCNT.
The estrus stage in female dogs was determined based on serum progesterone (P4) concentration analysis using the Vet chroma™ progesterone kit (Anivet, Chuncheon, Korea). The day of ovulation was regarded when the P4 concentration exceeded 8 ng/mL. At 72 hours after assumed ovulation, all oocytes of the oocyte donors were recovered using the oviduct flushing method through a surgical procedure. Briefly, the female dogs were initially anesthetized with 6 mg/kg ketamine and xylazine and maintained under anesthesia with 2% isoflurane. The fimbria of the oviduct was accessed through the bursal slit and cannulated using an inverted flanged bulb steel needle (18 gauge, 7.5 cm). A 24-gauge intravenous (IV) catheter (Angiocath™ Plus, Becton Dickison Korea, Seoul, Korea) was inserted into the isthmus of the oviduct near the uterotubal junction. Hepes-buffered TCM-199 (Gibco) supplemented with 10% FBS, 2 mM NaHCO3, 5 mg/ml Bovine serum albumin (BSA) and 1% penicillin-streptomycin was introduced into the oviduct through the IV catheter. Following the surgery, Cumulus-oocyte complexes (COCs) were promptly transported to the laboratory. A total of 282 oocytes were flushed from 25 oocyte donor dogs. Among these, 164 mature oocytes were used for SCNT, while the others were used for IVM.
The cumulus cells were removed from the retrieved COCs by repetitive pipetting in TCM-199 containing 10% FBS and 0.1% hyaluronidase. After the removal of cumulus cells, the oocytes were analyzed under a stereoscopic microscope. Oocytes with the first polar body in the perivitelline space were classified as mature, while oocytes lacking the first polar body were classified as immature. A total of 282 oocytes were collected from 25 dogs. Among them, 164 oocytes were classified as mature and used for SCNT, while Among the oocytes recovered from tubal flushing, 98 oocytes without the first polar body were classified and subjected to IVM. Immature oocytes were cultured for 24 h in IVM medium in the presence or absence of hormones (P4 + FSH).
For the IVM of in vivo-immatured oocytes, two types of culture medium were used as follows: 1) Non-treated (Control) group: TCM-199 containing sodium bicarbonate, 10% FBS, and 1% penicillin-streptomycin solution; 2) Hormone-treated group: TCM-199 containing 0.2 mM sodium pyruvate, 10% FBS, 5 μg/mL FSH (folltropin-V®, Bioniche, Belleville, Canada), and 2 ng/mL P4. Approximately 15 immature oocytes were cultured in a 40 μL drop of each medium at 39°C under 5% CO2 in 95% humidified air for 24 hours. The extrusion of the first polar body (MII) was evaluated under the stereomicroscope to assess IVM.
SCNT was performed as previously described [2]. Briefly, enucleation of mature oocytes were perfomed through removing the first polar body and MII chromosomes by aspiration and assessed by staining with Hoechst 33342 (bisbenzimide). After enucleation, injection of donor cells was performed. A donor cell with a smooth cell surface membrane was selected and transferred into the perivitelline space of an enucleated oocyte. Following injection, the oocyte donor cell complexes were equilibrated in fusion medium containing 0.26 M mannitol, 0.1 mM HEPES, 0.5 mM MgSO4, and 0.05% BSA, and then fused by two pulses of direct current (32 V for 15 μs) using an Electro-Cell Fusion apparatus (NEPA gene, Chiba, Japan). The success of fusion was confirmed after 30 minutes, and activation was performed using 10μM calcium ionophore (product No. C7522, Sigma-Aldrich) for 4 minutes, followed by 1.9 mM 6-dimethylaminopurine in PZM-3 for 3 hours and 30 minutes. The fused embryos were surgically transferred into the oviducts of estrus-synchronized surrogate mothers. Pregnancy was confirmed by ultrasonic diagnosis 30 days after embryo transfer.
Statistical analyses were performed using SPSS (version 26.0.0.0, IBM, USA), and significant differences in oocyte maturation were analyzed using the t-test, while data related to pregnancy rate and birth rate were analyzed using the chi-squared test. The significance level was set at p < 0.05.
RESULTS
We investigated the impact of the IVM medium in the presence or absence of the hormone combination (P4 + FSH) on the nuclear maturation of canine in vivo-immature oocytes. As shown in Fig. 1, nuclear maturation rates in the hormone-supplemented group (94.92 ± 3.15) were significantly (p < 0.05) higher than those observed in the control group (61.01 ± 4.23).
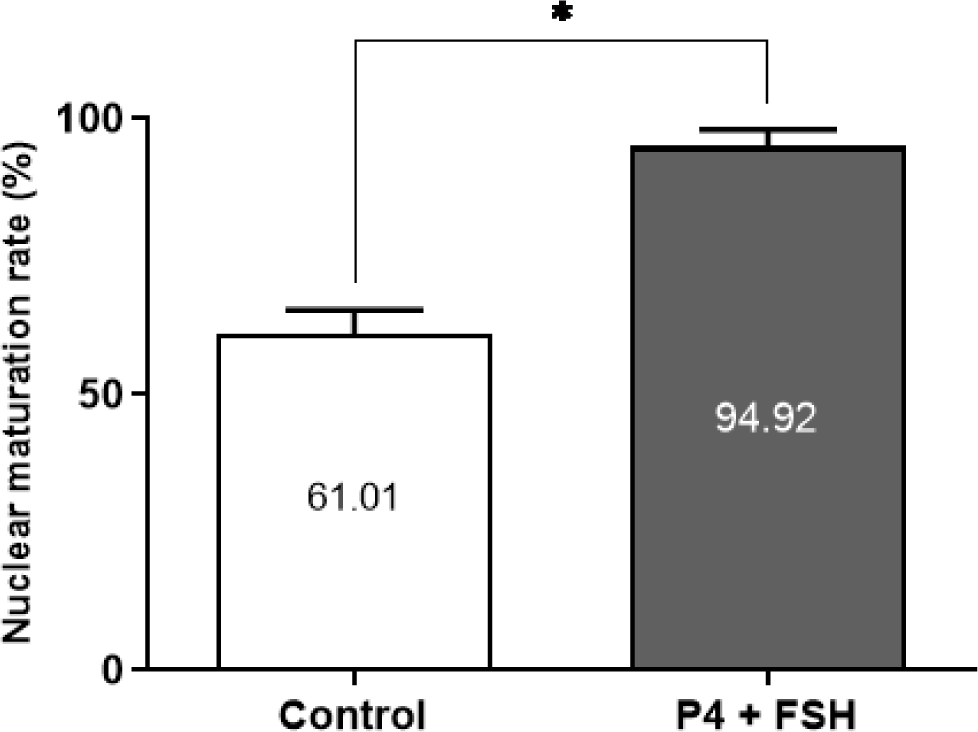
The results of the comparison of fusion rates between SCNT utilizing in vivo and in vitro matured oocytes are shown in Table 1. Among the 116 reconstructed embryos, 85 (73.28%) successfully fused in the in vivo group, whereas 14 out of 17 reconstructed embryos (82.35%) successfully fused in the in vitro group. No significant difference in the fusion rate was observed between the two groups.
Group1) | No. of oocyte donor dogs | No. of oocytes | No. of embryos | Fusion rate (%)2) | |
---|---|---|---|---|---|
Injected | Fused | ||||
In vivo | 15 | 164 | 116 | 85 | 73.28 |
In vitro | 2 | 20 | 17 | 14 | 82.35 |
The influence of different oocyte sources on the pregnancy rate with canine cloned embryos was also examined. Following embryo transfer, pregnancy was detected in three out of eight recipients that received cloned embryos in the in vivo group (37.5%) and in one out of two recipients that received cloned embryos in the in vitro group (50%) (Table 2). Notably, the number of offspring was significantly (p < 0.05) higher in the in vitro group (28.57%) than that in the in vivo group (8.23%). Among the three pregnant recipients in the in vivo group, six live puppies and one dead puppy were delivered, and the bodyweight of live pups were 112, 109, 121, 124, 103 and 121 g, respectively. Whereas the pregnant recipient in the in vitro group delivered three live puppies and one dead puppy, and the bodyweight of live pups were 124, 126 and 111 g, respectively. The necropsy of the dead puppies showed no specific pathological findings. All cloned dogs grew up healthy. Fig. 2 shows one -year-old cloned pups derived from the in vivo group (Fig. 2A) and the in vitro group (Fig. 2B).
Group1) | No. of transferred embryo | No. of recipients | No. of pregnancies (%)2) | No. of offspring (%)3) |
---|---|---|---|---|
In vivo | 85 | 8 | 3 (37.50) | 7 (8.23)a |
In vitro | 14 | 2 | 1 (50.00) | 4 (28.57)b |
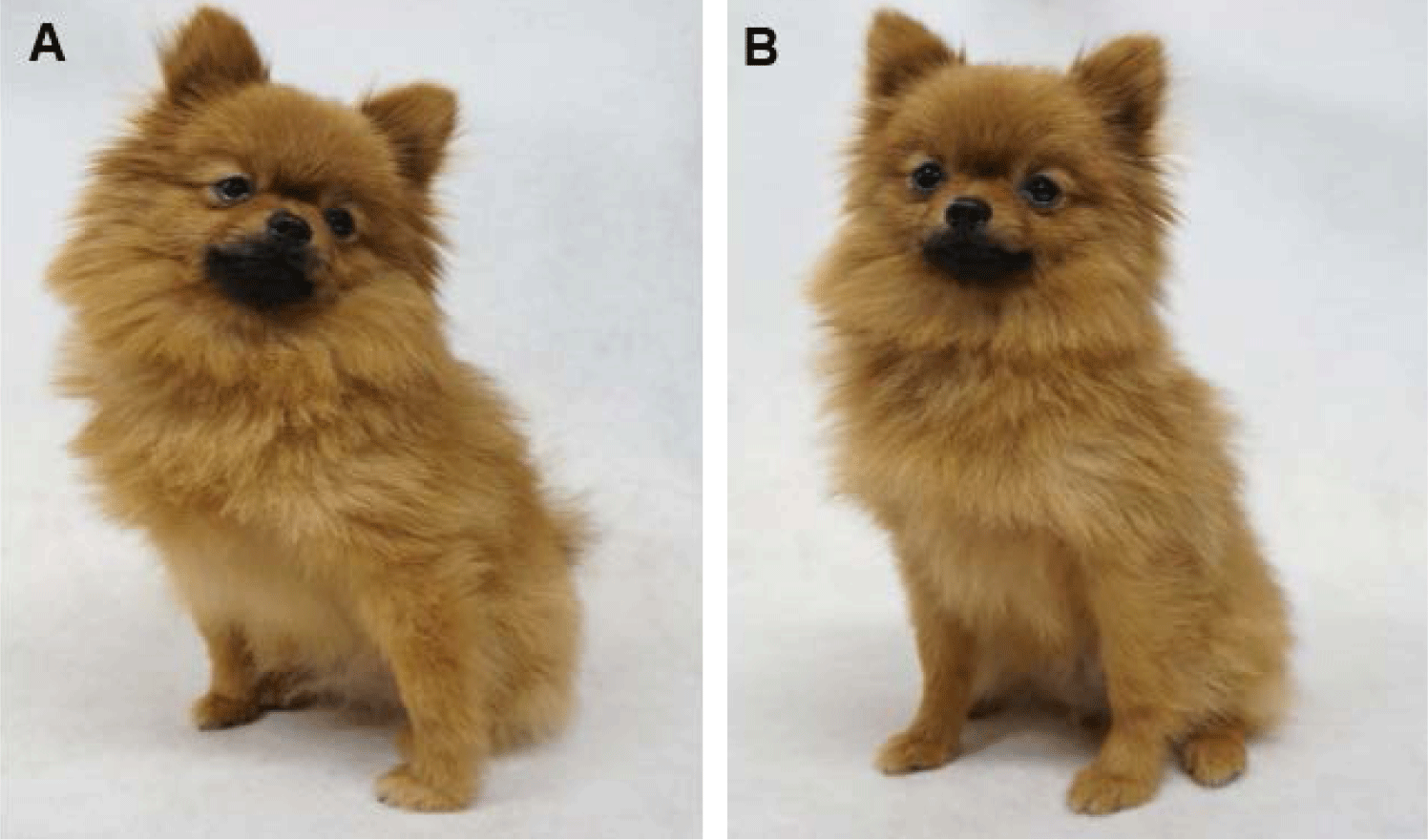
DISCUSSION
This study aimed to investigate the effect of hormone supplementation (P4 and FSH) in the IVM medium on immature oocytes obtained via oviduct flushing of naturally ovulated female dogs and assess their suitability for SCNT as oocyte donors. Our findings indicate that the groups supplemented with hormones exhibited significantly higher maturation rates than those exhibited by the control groups.
P4 is an intra-follicular steroid hormone that plays critical roles in ovulation, implantation facilitation, and pregnancy maintenance [29]. In canines, P4 production is initiated within the follicle before ovulation, and its levels rise above 1–3 ng/mL during the onset of the preovulatory LH surge and further increase after ovulation [30,31]. Willingham-Rocky et al. reported that supplementation with 200 ng/mL of P4 showed an increased meiotic resumption or maturation rate between groups, though not significantly [26]. Kim et al. reported a positive effect on the percentage of oocytes progressing to the MII stage in oocytes obtained during the follicular stage supplemented with 0.5–2 μg /mL of P4 [22]. Similarly, Vannucchi et al. reported that supplementation with 20 μg/mL P4 in the maturation medium positively affected meiotic resumption /but was insufficient to complete it [25]. Hu et al. found that a combination of 1.0 IU/mL FSH, 5 IU/mL P4, and 5 IU/mL E2 in the supplementation group led to a higher maturation rate of oocytes than that in the control group [24]. In this study, we expected that the immature oocytes after ovulation would have already been sufficiently affected by P4. Therefore, P4 was supplemented to the IVM medium at the minimum concentration maintained after ovulation.
FSH is widely used in IVM protocols for various mammalian species. FSH supplementation induces oocyte maturation [32,33] and is involved in cumulus cell expansion [34]. Hewitt and England reported that the supplementation of gonadotropins such as FSH and LH in the maturation medium for canine IVM resulted in a high maturation rate, though not statistically significant, with 1 μg/mL of FSH supplementation [35]. Kim et al. found that supplementing the maturation medium with 1 IU of FSH improved the maturation rate of canine oocytes [36]. Additionally, Lee et al. reported that supplementing various concentrations of FSH (ranging from 0.5 to 50 ug/mL) in the IVM medium significantly enhanced the expansion of cumulus cells, with the 5 μg/mL group showing the highest result for canine oocytes [23]. Hence, we adopted a supplement concentration of 5 μg/mL for FSH.
The combination effect of P4 and FSH on immature oocytes recovered through oviduct flushing after ovulation demonstrated sufficient capacity for meiotic resumption, leading to a higher maturation rate to the MII stage than that reported in other reports. This difference may be ascribed to the method of oocyte recovery. Previous reports on canine IVM mainly employed ovariohysterectomy to recover immature oocytes. However, in this study, we performed the recovery of immature oocytes through oviduct flushing 72 h after the assumed ovulation. Oocytes retrieved through ovariohysterectomy were selected based on morphological classification for good quality [37], whereas oocytes retrieved from the oviduct had been exposed to in vivo conditions for a longer duration, at least 72 h, which may have conferred them with higher meiotic competence than that of those recovered by ovariohysterectomy.
The stage of the estrous cycle may have an effect on the maturation rate of oocytes. In this study, all the recovered oocytes were focused on the follicular stage, specifically after ovulation. Interestingly, even at the same follicular stage, immature oocytes recovered from the oviduct after ovulation demonstrated higher meiotic competence than that demonstrated by immature oocytes retrieved directly from the follicles. Previous research has explored the effect of canine IVM across various estrus cycles [22,36,38,39]. Kim et al. reported that the stage of the estrous cycle for oocyte recovery is an important factor for successful meiotic competence of canine oocytes, with high glutathione (GSH) contents observed in in vitro matured oocytes collected from the follicular stage and in vivo matured oocytes [22,39]. Oh et al. reported similar results, showing a higher proportion of oocytes reaching MI and MII stages, regardless of the stage of the canine serum during the follicular stage [38]. In this study, we exclusively focused on oocytes recovered during the follicular stage, precisely after ovulation. The findings suggest that even at the same follicular stage, immature oocytes obtained from the oviduct after ovulation possess a higher meiotic competence than those retrieved directly from the follicles.
Subsequently, we conducted SCNT to investigate the viability of in vitro matured oocytes for cloning purposes. Interestingly, the difference between in vivo and in vitro oocyte maturation did not affect the fusion rate in SCNT. This result coincides with other reports in mammals [40-42]. Regarding the in vivo development of SCNT embryos between the in vivo and in vitro groups, the pregnancy rate did not show significant differences, but the number of offspring was significantly higher in the in vitro group than that in the in vivo group. These results suggest that in vitro groups have the same feasibility for the full-term development of cloned embryos as in vivo groups. Additionally, we successfully produced healthy cloned dogs in both groups. Notably, the cloned dogs in the in vitro group represent the first successful production of cloned puppies using in vitro matured oocytes.
In conclusion, this study marks the first report of an IVM system for canine immature oocytes recovered in vivo, leading to the successful production of cloned puppies using in vitro matured oocytes. To date, there have been no reports on the production of live puppies using in vitro matured oocytes. These results indicate that immature oocytes recovered in vivo hold promise as oocyte donors for SCNT and exhibit comparable full-term developmental potential to in vivo matured oocytes. However, it is important to note that the present study solely focused on immature oocytes after ovulation. Further research should consider investigating other estrous stages, which could potentially enhance canine cloning efficiency.