INTRODUCTION
The scale of the pet food industry has rapidly expanded as an increasing number of households worldwide have companion animals. Moreover, the change in the perception of companion animals (humanization trends, e.g., the pet treated as a member of a family) has prompted consumers to take an increasing interest in the quality of the diet of their pet [1]. Dog foods are composed of main nutrients such as proteins, lipids, carbohydrates, and trace nutrients such as minerals and vitamins. The European Pet Food Industry Federation (FEDIAF), National Research Council (NRC), and American Feed Control Officials (AAFCO) provide information on the recommended level of each nutrient for a balanced nutrient supply for companion animals [2–4]. Proteins, in particular, are an essential nutrient, supplying essential amino acids necessary for the growth and metabolism of companion dogs [5]. Hence, the level and digestibility of crude protein (CP) can be an important indicator for evaluations of the dog food quality [6]. CP digestibility data can also offer information on nutrient bioavailability to consumers to enhance the reliability of the dog food quality and provide value from the perspective of animal health and welfare [7].
Ethical issues of animal experimentation have increased worldwide. Efforts to support replacement, reduction, or refinement (3Rs) of animal use have been made in research. Moreover, the Food and Drug Administration in the US has announced that alternate methods can be used in preclinical research to determine the safety and efficacy of drug before human trials. Moreover, it is especially difficult to use dogs for experimentation because many people consider dogs as a companion animal and a member of the family. Regardless, evaluation of the nutritional value of foods (raw materials) for companion animals demands abundant time, financial investment, and animal experiments [8]. In the pet food industry, these limitations entail practical challenges in product development, quality control, and data provision for consumers through adequate assessments of food value in the pet food industry [9]. Therefore, it is necessary to develop an alternate method to research the nutritional utilization in dogs without animal experimentation.
The biological accessibility of nutritive or non-nutritive factors and nutrient digestibility can be analyzed using an in vitro digestion method that can simulate the digestive process of the body, with advantages such as controlled selectivity and high reproducibility [6,10,11]. Therefore, this method is suitable for exploring and proving new hypotheses in nutritional research. Furthermore, it has attracted attention as an alternative to animal experiments in fields related to food and nutrition, which could help avoid the recent ethical debates [12]. In vitro digestion models are also advantageous because they allow the selective simulation of physiological conditions (pH, temperature, enzymes, and microorganisms) in the digestive tract of an animal (oral cavity, stomach, small intestine, and ileum), in addition to being easy to use, low-cost, and independent of special devices [13]. Previous studies on in vitro digestion have included a wide spectrum of research subjects ranging from humans [14–16] to livestock [17–19] and fish [20]. Some sophisticated computerized in vitro digestion models can even simulate physical or physiological aspects, from the rate of food migration to enzyme concentrations and pH changes [21,22]. Notably, different in vitro digestion models have been reported for predicting digestibility in economically important animals, such as livestock [17,23,24], whereas very few studies have been conducted to predict digestibility in companion dogs. Studies on companion dogs using in vitro digestion models have presented regression equations to predict the digestibility of several commercial diets [6,25,26]. However, they have limitations in reflecting the characteristics of protein digestion observed in vivo because of the diversity of protein sources in commercial diets [27]. Hence, using regression equations to predict digestibility, the digestibility of CP can be estimated for a wide range of dog foods; however, precise prediction might not always be possible.
Therefore, this study was performed to evaluate the potential feasibility of an in vitro method for predicting the apparent total tract digestibility (ATTD) of CP in dogs via a static in vitro digestion approach and a regression equation.
MATERIALS AND METHODS
All animal experiments were conducted in compliance with the methods approved by the Institutional Animal Care and Use Committee of the National Institute of Animal Science (NIAS), Republic of Korea (approval number: NIAS-2021-513). All experiments were conducted on dogs owned by the NIAS (National Institute of Animal Science, Rural development Administration, Republic of Korea). All dogs were provided with an independent space (170 × 210 cm/dog) with a constant indoor temperature (22°C–23°C) and lighting (16 h light and 8 h dark cycle). The animals were provided with drinking water ad libitum and fed twice daily. The food intake was also measured daily. Each animal was provided with approximately 3 h of outdoor activity. The health of the dogs was monitored daily and was checked by a veterinarian in NIAS, if needed.
The experimental diets were designed to meet the nutritional requirements suggested by AAFCO [28] for adult dogs (Table 1). The CP level in diets was formulated to 20% (22.01%, Analyzed CP value as dry-based), 30% (31.35%, Analyzed CP value as dry-based), and 40% (41.34%, Analyzed CP value as dry-based) [28], and the diet was prepared according to the procedure reported by Seo et al. [29]. All ingredients used in the experimental diets were purchased as commercial powdered products without any palatants. As Table 1 shows, all ingredients were mixed, steamed, and formulated. After preparation, the experimental diet was stored at −20°C until use. The food was thawed and warmed to room temperature immediately prior to feeding. An extruded dry type of commercial dog food was purchased from a local brand in the Republic of Korea (chicken meat and rice flour as the base) containing 28.02% experimental CP (analyzed as the dry-based value) and was used to validate the linear regression equation for predicting the ATTD of CP. The chemical composition of the commercial dog diet is listed in Table 1.
All the experimental diets used chicken powder as the protein source, and each experimental diet was formulated to contain 20%, 30%, and 40% CP.
The commercial food was an extruded dry type (chicken and rice base) for adult dogs, and information on its chemical composition was the same as that provided by the manufacturer.
Provided per kilogram of experimental diets: vitamin A, 5,250 IU; vitamin D3, 375 IU; vitamin E, 37.5 mg; vitamin K, 0.078 mg; vitamin B1 (thiamine), 4.2 mg; vitamin B2 (riboflavin), 3.9 mg; vitamin B6 (pyridoxine), 3 mg; vitamin B12, 0.021 mg; D-calcium pantothenate, 9 mg; niacin, 45 mg; folic acid, 0.6 mg; biotin, 0.054 mg; taurine, 1,500 mg; FeSO4·H2O, 66 mg; MnSO4·H2O, 5.7 mg; ZnSO4·H2O, 75 mg; CuSO4·H2O, 11.25 mg; Na2SeO3, 0.27 mg; Ca(IO3)2, 1.35 mg.
The CP ATTD of the experimental diet was evaluated using eight healthy beagle dogs (5 years old; neutered 4 males, spayed 4 females). Food supply was set to meet the maintenance energy requirements estimated using the equation (metabolizable energy [ME], 132 kcal kg body weight [BW]0.75 per day) suggested by the Association of American Feed Control Officials [28]. Experimental diets were provided to dogs for two weeks including three days to adjust it and four days to collect feces.
The total collection method was used to analyze the in vivo CP ATTD. Fecal samples were collected for four days and weighed daily during the study period. Fecal samples were stored at −20°C until analyzed. To analyze the levels of water (Association of Official Analytical Chemists [AOAC] method 934.01) and CP (AOAC method 984.13) in fecal and food samples, the methods established by AOAC [30] were followed. The CP ATTD was calculated using equation 1 [31]:
The in vitro digestion method comprised two digestive steps, in reference to the static in vitro digestion method suggested by Seo et al. [13]. Digestion progresses sequentially from the gastric digestion phase to the small intestinal phase. The experimental diet was dried in a dry oven (65°C) and ground to a constant weight (<1 mm particle size). For the gastric digestion phase, a 10 g sample (<1 mm), 250 mL (25 mL/g feed) of 0.1 M phosphate buffer (pH, 6.0), and 100 mL (10 mL/g feed) of 0.2 M HCl were placed in an Erlenmeyer glass flask (volume, 1,000 mL). The pH of the mixture was adjusted to 2.0 using 0.1 M HCl. Next, 1 mL of pepsin-HCl solution (100 mg/mL of 0.075 N HCl solution and pepsin from the porcine gastric mucosa, ≥ 250 units/mg, #P7000, Sigma-Aldrich, St. Louis, MO, USA) and 1 mL of chloramphenicol solution (2.5 mg/mL EtOH, #C-0378, Sigma-Aldrich) were added, and the mixture was incubated in a shaking incubator (39°C, 150 rpm) for 6 h. For the small intestinal phase, 100 mL (10 mL/g feed) of 0.2 M phosphate buffer (pH, 6.8) and 50 mL (5 mL/g feed) of 0.6 M NaOH were added to the digestive fluid after the gastric digestion phase. The pH of the mixture was adjusted to 7.5 using 0.1 M NaOH. Next, 12.5 g of bile salts (1.25 g/g feed, #13805, Sigma-Aldrich) and 2.50 g of pancreatin (0.25 g/g feed, #P7545, 8 × USP specifications, Sigma-Aldrich) were added, and the mixture was incubated in a shaking incubator (39°C, 180 rpm) for 18 h. At the end of the entire digestive process, the undigested fraction was collected using a bottle top vacuum filter (pore size, 0.22 µm; polyethersulfone membranes, TPP Techno Plastic Products AG, Zurich, Switzerland) and dried in a dry oven (65°C) for subsequent analysis.
For the CP level and digestibility, the CP content (AOAC method 984.13) was measured in the undigested fraction from a static in vitro simulation of digestion and experimental diet, following the methods established by AOAC [30]. For these calculations, equation 2 was used [13].
The linear regression equation for predicting the ATTD of CP in adult dogs was determined based on the co-relation between the average in vitro and in vivo CP digestibility data for the experimental diet (CP, 20%–40%). Briefly, to evaluate the regression equation, a commercial diet of the extruded dry type (chicken meat and rice flour as the base) containing 28.02% CP (analyzed value as dry-based; Table 1) was used.
First, a commercial diet was assessed the in vitro digestion process and the CP level in undigested fraction was measured using the same methods as those used for the experimental diet. In vitro digestibility was calculated according to Equation 2. Second, CP ATTD was analyzed by feeding the commercial diet to nine healthy beagle dogs (5 years old, neutered 1 male and spayed 8 females). Total feces were collected and CP level was measured. CP ATTD was calculated according to Equation 1. Third, the in vitro CP digestibility data for the commercial diet were applied to the regression equation to predict the ATTD of CP. In final, the predicted in vivo CP digestibility was compared with the ATTD of CP for the commercial diet tested using nine healthy beagle dogs. The index of similarity between the predicted digestibility and ATTD of CP was calculated according to equation 3 [32]. The index of similarity value closer to 100 means more similar between the two CP digestibility value.
SPSS (ver. 17.0, 2008; SPSS Statistics, Chicago, IL, USA) software was used to perform all statistical analyses, and the results were expressed as the mean ± standard error of the mean. One-way analysis of variance was used to analyze the changes in food intake and digestibility according to the variation in CP levels in the dog food. Tukey’s multiple comparison test was used to test the significance of differences between groups. The Student’s t-test was used to analyze the differences between in vitro and in vivo CP digestibility. The level of significance was set at p < 0.05. To analyze the correlation between CP levels and in vitro or in vivo CP digestibility, Pearson’s bivariate correlation analysis was performed.
RESULTS
To assess in vivo CP digestibility, ATTD was evaluated in beagle dogs fed nutritionally controlled experimental diets. During the study period, the mean daily food intake, metabolic energy intake (MEI), and MEI per metabolic body weight (mBW) did not differ significantly among the experimental groups (p > 0.05; Table 2). In contrast, the mean daily CP intake was different among the groups fed an experimental diet with 20%, 30%, and 40% CP, with values of 46.48 ± 1.20, 62.50 ± 2.46, and 78.65 ± 5.43 g/day, respectively (Table 2). The mean daily CP intake increased according to the CP level in the experimental diet (p < 0.001).
The results of ATTD of CP was 89.26 ± 0.60% when fed 20% CP diet, indicative of the lowest digestibility when compared to that of the experimental diet with 30% CP (92.19 ± 0.68%) or 40% CP (94.02 ± 0.40%) (p < 0.05; Fig. 1A).
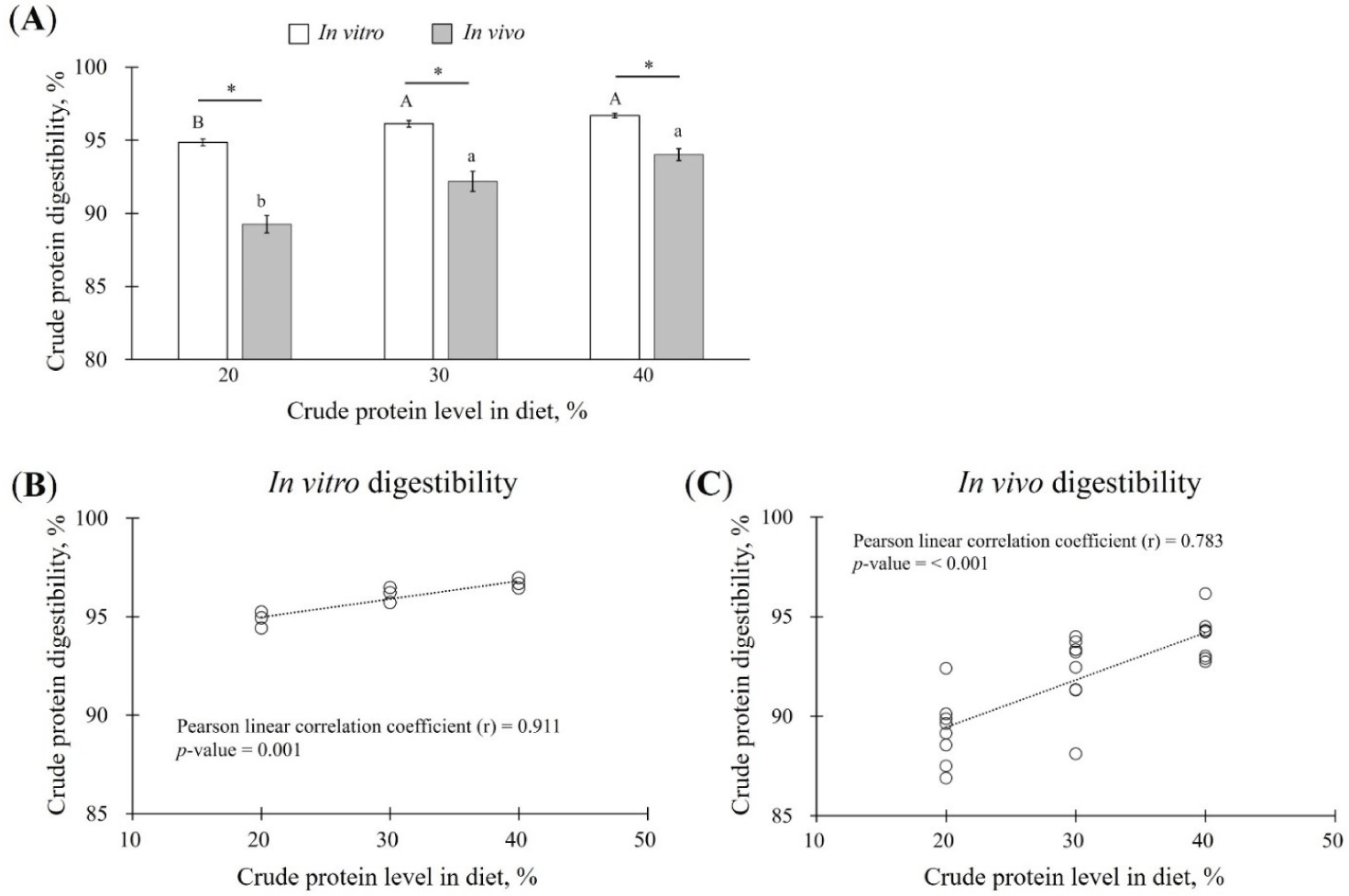
Next, CP digestibility assessed using the static in vitro digestion method was 94.86 ± 0.24%, 96.13 ± 0.23%, and 96.69 ± 0.15% for the experimental diets with 20%, 30%, and 40% CP diets, respectively. In vitro CP digestibility thus tend to increase in a concentration-dependent manner in diets containing 20%, 30%, or 40% CP (p < 0.05; Fig. 1A). In addition, CP digestibility was higher in the in vitro digestion method than that in ATTD for all experimental diets (20%–40% CP) (p < 0.05; Fig. 1A).
CP digestibility under both in vitro and in vivo conditions tended to increase with increasing CP concentrations in the experimental diets (Fig. 1A). To determine the correlation between the CP concentration in the experimental diet and in vitro or in vivo digestibility, Pearson’s correlation analysis was performed. The correlation coefficient between in vitro CP digestibility and CP levels in the experimental diet was 0.911 (p = 0.001; Fig. 1B). The coefficient between in vivo CP digestibility and the CP level in the experimental diet was 0.738 (p < 0.001; Fig. 1C).
The in vivo and in vitro CP digestibility data of 20%, 30%, and 40% CP contained diets were subjected to a linear regression analysis to predict the ATTD of CP in adult dogs. The linear relationship among the three different CP concentrations is shown in Fig. 2, and the regression equation was calculated using equation 3.
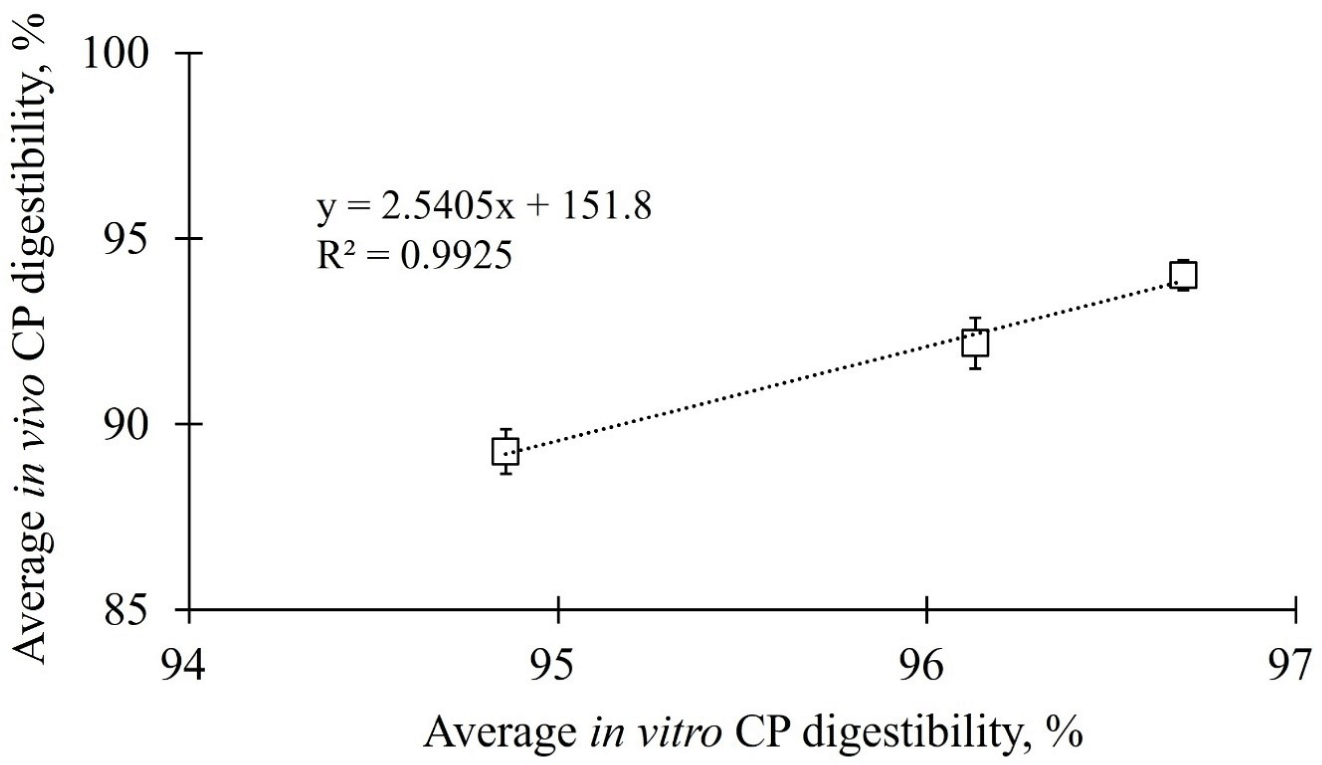
The regression equation developed to predict CP digestibility was validated using a commercial diet, which was an extruded dry type (chicken meat and rice flour as the base) containing 28.02% CP (analyzed value as dry-based). The CP digestibility of the commercial diet was 92.82 ± 0.09%, as calculated using the static in vitro digestion method. The CP digestibility results were applied to the regression equation (Eq. 3) and the in vitro CP digestibility was calculated to be 84.00 ± 0.23% (Table 3). Meanwhile, to obtain the in vivo CP digestibility of the same commercial diet used for in vitro digestibility, a feeding test was performed in dogs and the in vivo CP digestibility was calculated to be 83.87 ± 0.65% (Table 3). The index of similarity between the predicted CP digestibility using the regression equation after in vitro digestion and the measured ATTD of CP in dogs was shown to be 100.16 ± 0.65% (Table 3).
The commercial diet used was a chicken- and rice-based adult dog diet (CP, 28.02%; analyzed as a dry-based value).
Predicted in vivo CP digestibility value was calculated by applying the in vitro CP digestibility value to the equation for predicting in vivo CP digestibility.
DISCUSSION
Data on nutrient bioavailability in the food are essential to provide well-balanced pet foods [6,7]. Proteins, in particular, comprise essential amino acids necessary for growth, homeostasis, and consistent immunity in companion animals. Amino acid content and types vary across different protein-based raw ingredients, and the in vivo amino acid availability or requirement can vary according to the type of ingested protein source [33]. Hence, it is necessary to evaluate the nutritional value of the food based on the protein source present in the dog food. Evaluations of ATTD have been widely used as a general method to obtain the value of in vivo CP digestibility [34]; however, this method requires time and is costly, with the most serious drawback being the social concern regarding animal experimentation. Consequently, despite consumer demand, the dog food industry is struggling to provide accurate and efficient data on the in vivo nutrient utilization of dog foods. Therefore, this study suggested the combination of a static in vitro simulation of digestion and a linear regression equation to improve the evaluation method for in vivo nutrient utilization, especially for proteins. As a result, the estimated in vivo digestibility of CP was highly similar to the in vitro digestibility of CP from ATTD in dogs.
To investigate the correlation between the estimated in vitro digestibility and measured in vivo digestibility, the digestibility of experimental diets containing varying levels of CP was assessed under in vitro and in vivo conditions. Experimental diets with 20%, 30%, and 40% CP concentrations were prepared using chicken meat powder, and the variation in the contents of other nutrients was minimized by applying the nutritional requirements of AAFCO. In vitro digestibility was evaluated by a static in vitro simulation of digestion and in vivo digestibility was calculated using ATTD in dogs. The results of this study revealed that, for all experimental diets, CP digestibility was higher under in vitro conditions than under in vivo conditions. In vivo digestion is influenced by various parameters (e.g., protein types, bacteria, and endogenous proteins) and complex digestive mechanisms inside the body. For example, in vivo digestion is a dynamic process characterized by pH changes and the continuous production and release of digestive enzymes that occur with the flow of food through different compartments of the digestive organs (oral cavity, stomach, small intestine, and ileum) [12]. In contrast, in vitro digestion was performed under strictly controlled conditions of pH, temperature, enzyme content, and digestion time [6,25,26,35,36,37]. Therefore, the different degrees of digestibility under these two conditions can be explained. Despite clear limitations in simulating an actual digestive process using a static in vitro digestion model [38], this study demonstrated a correlation between in vitro and in vivo digestibility.
The results of this study also demonstrate a trend of increasing CP digestibility under the two digestion conditions (in vitro and in vivo), in line with the increase in CP concentration in the experimental diet. Pearson’s correlation analysis showed that, under both in vitro (r = 0.911, p = 0.001) and in vivo (r = 0.783, p < 0.001) conditions, CP digestibility exhibited a significant linear correlation with CP levels in the experimental diet. This implies a definite correlation between the CP levels in dog food and CP digestibility. A previous study by Yamka et al. [39] reported similar findings in vivo. They examined the digestibility of foods (low-ash poultry meal base) containing varying concentrations of CP (10%–25%) in healthy adult dogs and showed that an increase in the CP level in the food increases CP digestibility [39]. Thus, the trend of increasing CP digestibility with the increase in CP concentration was observed. Moreover, the findings of this study support a strong correlation of digestibility between in vitro digestion using a static in vitro simulation method and in vivo digestion using ATTD.
For reasons related to their advantageous speed and cost, prediction models for in vivo digestibility using regression analysis are being widely investigated for economically important animals (cattle, poultry, pigs, and rabbits), companion animals, and humans [6,40–42]. Prediction models for in vivo digestibility using regression analysis have not only identified nutrients in foods, including carbohydrates [24], CP [43], and phosphorus [44], but have also verified the potential feasibility of prediction models using regression analysis for energy [23] and the postprandial glycemic index [45]. Nevertheless, there have been few studies on prediction models for in vivo digestibility using regression analysis in dogs. Biagi et al. [6] assessed 16 commercial diets (extruded dry type) and found that the coefficient of determination of the linear regression equation was low for the estimation of CP ATTD in adult dogs (r2 = 0.510) [6]. Kawauchi et al. [38] proposed a CP digestibility prediction model based on 16 dog foods, including meat, bone meal (MBM), and poultry by-product meal (PM). Interestingly, they proposed an independent equation for each of the two protein-based raw materials; however, the reliability of both equations was low (MBM: r2 = 0.126; PM: r2 = 0.216) [38]. In this study, the regression equation was generated based on the correlation results of both static in vitro simulation and in vivo ATTD and showed a highly reliable coefficient value for the CP prediction equation for dogs (r2 = 0.9925). Moreover, the predicted CP digestibility using the regression analysis with the data from the static in vitro digestion of CP had a significantly high similarity (100.16%) with that of in vivo CP digestibility in dogs. This suggests that the regression equation proposed in this study has potential utility in predicting ATTD of CP in adult dogs. To test the reproducibility of the regression equation, a commercial dog food (extruded dry type) from a local brand in the Republic of Korea was used in this study. As the experimental diet was produced in lab, the digestibility of the commercial dog food was assessed using two methods: a static in vitro simulation method and ATTD in dogs. The in vitro digestibility was predicted using a regression equation and the in vivo digestibility was calculated as ATTD in dogs. The predicted CP digestibility of the commercial dog food using the regression equation was not significantly different from the in vivo ATTD measured in dogs and showed 100.16 ± 0.65% similarity. Therefore, the regression equation is suitable not only for experimental diets produced in the lab but also for commercially produced extruded dry type diets to predict the in vivo digestibility.
Animal feeding tests in the nutritional research field is a common and essential process, although it requires prohibitive cost, a safe animal facility, animal experts, and technical support. A static in vitro simulation of digestion is cost effective and requires minimum experimental skill and lab space, unlike animal experiments. The combination of a static in vitro simulation of digestion method and the regression analysis allows researchers to obtain information on the digestibility of CP without the necessity of animal experimentation. Thus, the data in this study are expected to prove the nutritional value of proteins for the development and production of dog food and in fields related to nutrition, where in vivo digestibility must be predicted according to varying protein content.
Evaluation of in vivo utilization of nutrients, especially proteins, is crucial not only for companion dog health management but also for the use of an optimized level of high-cost protein-based raw materials, contributing to more reasonable purchases by consumers. However, the pet food industry claims that it is difficult to evaluate the in vivo utilization of nutrients when they develop or produce pet food products because of the requirement of animal experiments. Therefore, the non-animal method to predict in vivo digestibility using an in vitro digestion technique and regression analysis is expected to satisfy both the animal feed industry and consumers. In conclusion, this approach may be a simple and reproducible potential method for assessing the CP digestibility of dog diet, but a further research considering the characteristics of the various protein sources that dog diet may contain is absolutely necessary.