INTRODUCTION
Soybean meal is the major protein source in the pig diet. In recent years, because of continuous increases in soybean meal prices and transgenic crop safety problems, alternative protein sources, including bean dregs, have been widely sought to reduce the content of soybean meal in feed [1,2]. In the process of making soy milk and tofu, soybeans are first mechanically crushed and then cooked, and the residue left over from the final filtration is soybean dregs [3]. The dry matter of bean dregs contains 27% protein and 53% carbohydrate, which contains more fiber and less protein than soybean meal [4,5]. Bean dregs contain easily decomposable carbohydrates and amino acids and are rich in carbon and nitrogen [6]. China produces over 80,000 tons of bean dregs annually, and most bean dregs are discarded [7]. However, trypsin inhibitors, the major anti-nutritional factor in soybean dregs, can reduce the digestion and absorption of dietary protein [8]. Reducing the anti-nutritional factors in soybean dregs has become a significant problem in the utilization of soybean dregs.
Feed fermentation is a promising solution to reduce these adverse effects of trypsin inhibitors [6,9]. Because bean dregs contain insoluble fiber and 70% moisture, wheat bran was added to reduce the moisture to 45%, which was suitable as a substrate for solid fermentation [10]. Wheat bran also contains a moderate quantity of starch that can contribute to the fermentation of the mixed product. Fermentation by Bacillus subtilis or Aspergillus oryzae can significantly enhance the relative content of crude protein [11,12]. It can degrade antigenic proteins and trypsin inhibitors in soybean protein [13]. Allergic reactions and immunoreactivity induced by soybean protein can be reduced by microbial fermentation [14]. Furthermore, oligosaccharides extracted from fermented bean dregs significantly reduced the concentration of ammonia nitrogen and pH and elevated short-chain fatty acid levels in imitation gut fermentation [15], which showed that fermented bean dregs could have a prebiotic function.
The profitability of large-scale pig farms depends on sow productivity, including litter size, piglet weight, and sow reproductive performance [16]. Nutrition in the maternal diet plays a vital role in fetal development and offspring growth, making it an essential factor to consider when feeding sows. The fetus gains weight rapidly in late gestation (GD), which is the critical period for fetal growth [17,18]. We chose to intervene nutritionally in sows during late GD.
This study aimed to assess the effect of a fermented mixture of bean dregs and wheat bran (FBW) in compound feed for sows during late GD on production parameters, nutrient digestibility, colostrum composition, fecal microbial flora, and constipation.
MATERIALS AND METHODS
This experiment was approved and conducted under the supervision of the Animal Care and Use Committee of Nanjing Agricultural University (Nanjing, Jiangsu Province, China). All animals were raised and maintained per the Animal Care and Use Guidelines of Nanjing Agricultural University (SYXK (Su) 2011-0036).
The experiment was conducted on a pig breeding farm (Suqian Municipality, Jiangsu, China). A total of 24 sows (PIC, Camborough) at GD 85 with parities of 5.98 ± 0.41 were selected based on body weight (BW) (228.75 ± 5.4 kg), and they were assigned to 4 groups (n = 6). The dietary treatments were 1) Control (CON; diet without FBW), 2) FBW3 (CON+3%FBW), 3) FBW 6 (CON+6%FBW), and 4) FBW 9 (CON+9%FBW). The FBW used in this trial was fermented by bean dregs and wheat bran with B. subtilis, Lactobacillus acidophilus, Saccharomyces cerevisiae, and Enterococcus. faecalis. The weight ratio of bean dregs (wet) to wheat bran was 4:6. The 4:6 ratio was DM-based. After the raw materials were fully mixed, the mixed bacterial fluid was inoculated, and the inoculation proportion was 6%. The proportions of L. acidophilus, S. cerevisiae, B. subtilis, and E. faecalis in the mixed broth were 2:2:1:1. The initial water content of the fermentation was 45%, the fermentation tank was sealed. First, ferment at 35°C for 4 hours, then the fermenter heats up to 37°C and continues to ferment for 8 hours, then the fermenter cools down to 30°C and continues to ferment for 36 hours. The chemical analysis of FBW is presented in Table 1. The basal diet was formulated according to NRC (2012) for gestating and lactating sows. FBW was supplemented when it was wet but calculated as an air-dry condition. The ingredients and compositions of the diets are provided in Table 2.
Premix provided the following per kg of complete diet: vitamin A, 50 KIU; vitamin D3, 15 KIU; vitamin E, 200 IU; vitamin K3, 8 mg; vitamin B1, 10 mg; riboflavin, 12 mg; vitamin B6, 12 mg; niacin, 200 mg; folic acid, 6 mg; pantothenic acid, 100 mg; sodium chloride, 3.0%–6.0%; choline chloride, 2,000 mg; iron, 1,200 mg; copper, 200 mg; manganese, 120 mg; zinc, 800 mg; iodine, 1.4 mg; selenium, 1.0 mg; Ca, 4.0%–8.0%; total P, 1.5%; Lysine, 0.16%.
The sows were fed different diets from GD 85 to 19 of lactation (LD) when the piglets were weaned. The average day for the gestating period was 114 days. The sows were provided with 3 kg of feed every day from GD 85 to GD 111, and they were fed twice daily at 06:30 and 15:30. Then, the feed allowance was reduced by 0.5 kg/d until the farrowing day. Sows were fasted on the farrowing day and were fed 2 kg/d beginning at LD 2. The ration was gradually increased by 1 kg/d until d 6 postpartum, and then the sows were allowed ad libitum experimental diets and water. During LD, sows were fed daily at 06:30, 10:00, 15:30, and 20:00. The sows were housed individually in GD crates (2.2 m length × 0.65 m width) and were transferred to individual farrowing crates (2.2 m length × 1.5 m width) at GD 110.
The numbers of total piglets born, alive and stillborn were recorded on the farrowing day. Body length was measured from the occipital bone to the root of the tail. BW and length were used for the calculation of body mass index (BMI): [BW (kg) / length (m)2] [19]. Cross-fostering was maintained within the diet treatment to adjust the small size to approximately 12.20 ± 0.21 piglets per sow within 3 d after birth. Cross-fostering occurred within the treatments as GD management of the sows was standard. Death rates were recorded after cross-fostering had been completed, and those dead piglets were not replaced. No feed was offered to the piglets, and sow troughs were sufficiently high to prevent the piglet from eating sow feed. However, piglets were allowed ad libitum water via nipple drinkers at weaning, and the number of weaned piglets was recorded. Piglets were weighed within 24 h of birth (LD 0) and weighed on LD 3, 7, 14, and upon weaning. After weaning, estrus was assessed in sows for 21 d, and the weaning-to-estrus interval (WEI) of sows was recorded. Unconsumed feed was weighed daily, and the average daily feed intake (ADFI) was evaluated.
Blood samples (5 mL) were collected from all sows via jugular venipuncture 2 h after feeding on the morning of LD 1 and on the weaning day. Blood samples were collected from 12 randomly selected piglets per group on LD 19 via jugular venipuncture. Blood samples were centrifuged for 10 min at 1,100×g force and 4°C, and serum was collected and stored at –20°C for further analysis. Colostrum samples (30 mL) were hand stripped from median mammary glands on both sides from all sows within 3 h of farrowing and were analyzed fresh. Milk yield was calculated as litter gain × 4.2 [20].
Every day during LD, we ranked the feces of each sow by visual qualitative evaluation. Feces were scored according to [21]: 0 = absence of feces, 1 = dry and pellet-shaped, 2 = between dry and normal, 3 = normal and soft, but firm and well-formed, 4 = between normal and wet, still formed but not firm and 5 = very wet feces, unformed and liquid. Depending on the number of consecutive days with no fecal production, we classified the grade of constipation as mild (no feces for 2 consecutive days), severe (no feces for 3 or 4 consecutive days), and extremely severe (no feces for more than 5 consecutive days).
At LD 19, fresh fecal samples were collected in sterile 2-mL centrifuge tubes without any treatment, and these samples were stored at −80°C until they were used for 16S rDNA gene sequencing analysis. Fecal samples were collected in plastic bags and fixed on site by mixing with 10% hydrochloric acid (10 mL hydrochloric per 100-g fresh feces) on the last 3 days of LD. The total weight of feces was not less than 200 g per sow. Diet samples were collected in plastic bags by quartation at the same time. Fecal and diet samples were stored at −20°C before the apparent nutrient digestibility assessment.
Serum total protein (TP) and blood urea nitrogen (BUN) metabolite assays were conducted with commercial kits purchased from Nanjing Jiancheng Biotechnology, Nanjing, China. The absorbance was measured using a microplate reader, and the amount of serum BUN was converted. Colostrum composition analysis was detected by the principle of the Fourier transform infrared technique using MilkoScan TM FT2 (Combifoss FT, FOSS Electric, Hillerød, Denmark). Fecal samples were thawed and over-dried at 65°C for 72 h. Dried feces and experimental diets were ground and passed through a 1 mm screen before chemical analysis. Hydrochloric acid insoluble ash (AIA) was used as an indigestible marker to assess the apparent digestibility of the dietary components. The contents of AIA, dry matter (DM), CP, crude fiber (CF), ether extract (EE), calcium (Ca), and total phosphorus (P) were determined according to official methods of analysis [22]. The apparent total tract digestibility (ATTD) was determined using the following formula [23]:
where AIAD indicates the AIA concentration in the diet; AIAF indicates the AIA concentration in the feces; DCF indicates the dietary component concentration in the feces, and DCD indicates the dietary component concentration in the diet.
Microbial genomic DNA was isolated from fecal samples using a QIAamp-DNA stool mini kit (Qiagen, Hilden, Germany) according to the manufacturer’s instructions. Serial dilutions of positive plasmids were saved in our laboratory. They were used to create standard curves using quantitative real-time PCR (Vazyme Biotech, Nanjing, China) with species and genus-specific primers (Table 3), permitting estimations of absolute quantification based on individual gene copies. The reactions were performed in a total volume of 20 μL, containing 2-μL of template DNA, 0.4-μL of forward and reverse primers, 10-μL of SYBR Green PCR Master Mix (Vazyme Biotech), 0.4-μL of Rox-2, and 6.8-μL of nuclease-free water. The thermal cycling conditions involved an initial denaturation step at 95°C for 30 s, followed by forty cycles of 95°C for 10 s and the appropriate annealing temperature (Table 3) for 30 s. Then, a melting curve was produced to confirm the specificity of amplification. The data were generated as gene copy numbers per gram of wet feces.
Target organisms | Primers | Sequence (5'-3') | Product size(bp) | Annealing temperature (°C) | Reference |
---|---|---|---|---|---|
Total bacteria | Total-F | GTGSTGCAYGGYYGTCGTCA | 123 | 60 | Suzuki et al. [45] |
Total-R | ACGTCRTCCMCNCCTTCCTC | ||||
Clostridium cluster IV | C.leptum-F | GCACAAGCAGTGGAGT | 240 | 60 | Matsuki et al. [46] |
C.leptum-R | CTTCCTCCGTTTTGTCAA | ||||
Clostridium clusterXIVa | Clo14-F | CGGTACCTGACTAAGAAGC | 189 | 60 | Matsuki et al. [46] |
Clo14-R | AGTTTYATTCTTGCGAACG | ||||
Lactobacillus | Lac-F | AGCAGTAGGGAATCTTCCA | 341 | 64 | Khafipour et al. [47] |
Lac-R | ATTCCACCGCTACACATG | ||||
Bacteroidesspp. | Bac303-F | GAAGGTCCCCCACATTG | 126 | 60 | Bartosch et al. [48] |
Bfr-Fmrev-R | CGCKACTTGGCTGGTTCAG | ||||
Escherichia coli | E.coli-F | CATGCCGCGTGTATGAAGAA | 95 | 60.8 | Huijsdens et al. [49] |
E.coli-R | CGGGTAACGTCAATGAGCAAA |
Data were analyzed using the SPSS 20.0 package (SPSS, Chicago, IL, USA), and a one-way ANOVA was performed on the four treatment groups. One-way ANOVA also analyzed linear and quadratic polynomial trends. When the effect was significant, means were compared with each other using the Tukey multiple range test. Before analysis, the data were tested for normality and homoscedasticity. A sow and litter were treated as an experimental unit, and dietary treatment was the only fixed experimental effect. Data are presented as the means and SEM. Differences were considered significant when p < 0.05.
RESULTS
The effects of FBW on the apparent digestibility of sows are shown in Table 4. The apparent digestibility of DM was significantly increased by FBW supplementation by treatment (p < 0.01), linear (p < 0.01), and quadratic analysis (p < 0.05). Among them, the apparent digestibility of P was significantly higher with increasing concentrations of FBW by treatment (p < 0.01), linear (p < 0.01), and quadratic analysis (p < 0.01). There was no significant effect on the apparent digestibility of CF, EE, and Ca.
As shown in Table 5, during the first week of LD, the ADFI of sows in the FBW 6 and FBW 9 groups was significantly higher than that of the CON group (p < 0.01). During the third week of LD, the ADFI was significantly increased by linear analysis (p < 0.05). The diets supplemented with FBW significantly increased the ADFI during the LD period by treatment (p < 0.05), linear (p < 0.05), and quadratic analysis (p < 0.05). Maternal FBW supplementation did not affect litter size or the weaning survival rate during the LD period. Piglets at birth, after cross-foster, and at LD 7 showed no treatment effects on litter weight. However, the litter weight of the CON group sows was significantly lighter than that of the other 3 groups at LD 14 by treatment (p < 0.05), linear (p < 0.05), and quadratic analysis (p < 0.05). The litter weight of the CON group sows was significantly lighter than that of the other 3 groups at LD 19 by treatment (p < 0.01), linear (p < 0.01), and quadratic analysis (p < 0.05). The mean piglet weight and piglet average daily gain were not affected by maternal FBW supplementation throughout the LD period. The milk yield of the treatment groups was significantly increased (p < 0.05). The BMI of the treatment groups at birth was found by quadratic analysis to be significantly increased compared with that of the CON group by quadratic analysis (p < 0.05).
As shown in Table 6, maternal FBW supplementation did not affect the CP, lactose, fat, solid, or solid-not-fat contents. A significant decrease was observed in urea concentration (p < 0.05). As shown in Table 7, the concentration of BUN in sows and piglets’ serum at weaning was found to be significantly decreased (p < 0.01), and the concentration of TP in piglet serum at weaning was significantly increased in FBW groups compared with that of the CON group (p < 0.01). The concentration of BUN in weaning sows and piglet serum was significantly different among the treatments (p < 0.01).
As shown in Table 8, compared with the CON group, sows treated with FBW had lower fecal Escherichia coli counts by treatment (p < 0.01), linear (p < 0.05) and quadratic analysis (p < 0.05). The fecal count of Clostridium cluster XIVa in the FBW 3 group was significantly decreased compared to that in the CON group (p < 0.05). As shown in Fig. 1A, during the 3 weeks of LD, the fecal scores of the FBW 6% and FBW 9% groups were significantly higher (p < 0.05) than the fecal scores of the CON group every week, and the fecal scores of the FBW 3% group were significantly higher (p < 0.05) than the fecal scores of the CON group only in the second week. The grade of constipation for the sows is shown in Fig. 1B.
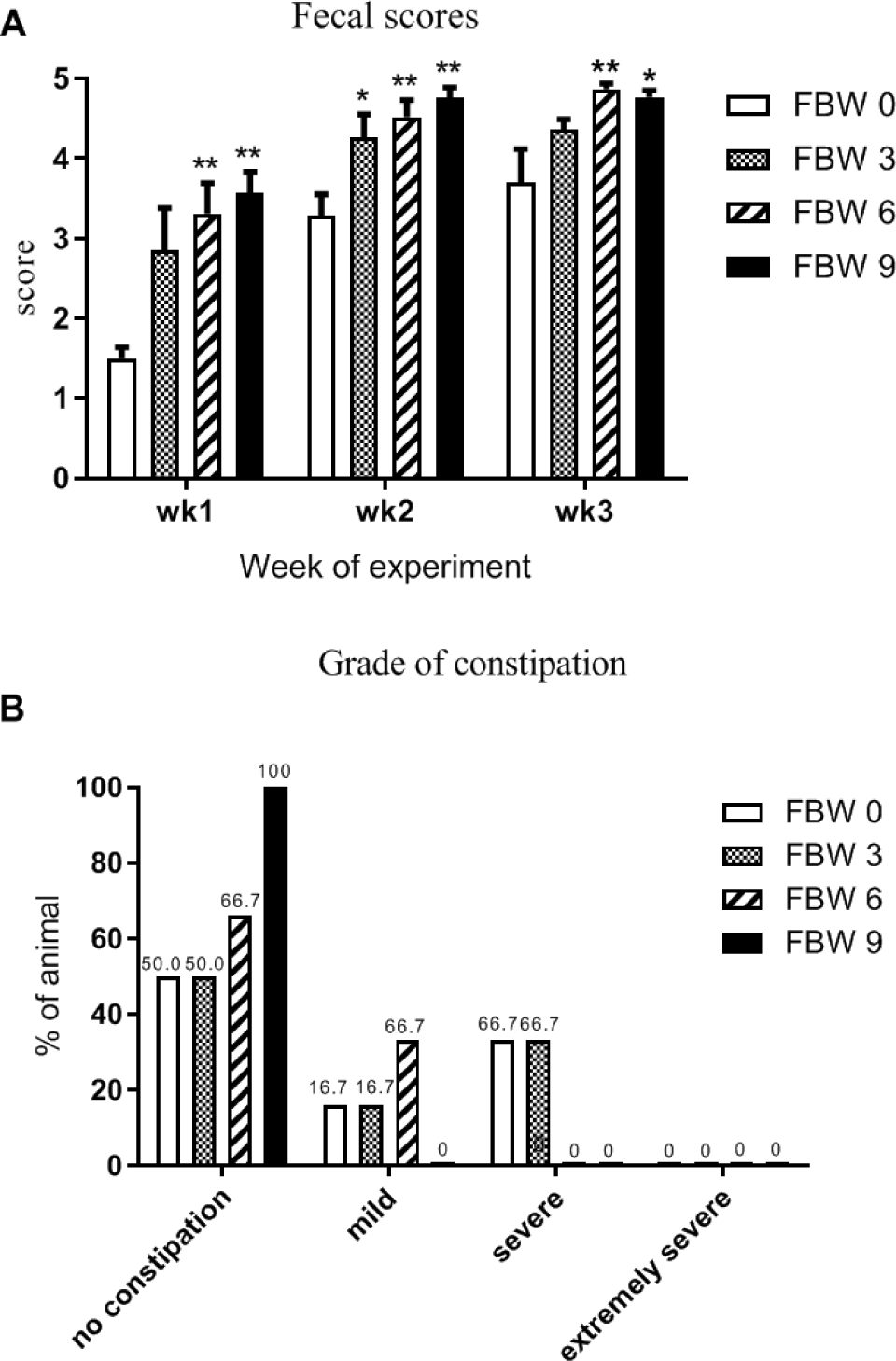
DISCUSSION
In the current study, DM and P retention were higher in the FBW treatments than in the CON treatments. Studies assessing the effects of FBW in sows are limited. The function and morphology of the small intestine are often used to measure indicators of digestion and nutrient absorption. Studies have shown the utilization of supplemented fermented diets to modulate the intestinal microbial community structure and activity and enhance the integrity and function of the intestinal epithelial barrier [24-27]. In addition, a study indicated that fermented soya bean extracts reduce the adhesion of enterotoxigenic E. coli to intestinal epithelial cells in pigs and prevent diarrheal diseases [28]. Although the small intestine morphology was not investigated in this study, the positive effect of fermented forage on intestinal function may lead to the enhancement of nutrient digestion and absorption ability. Since a large amount of P is often produced in fermented feed [29,30], it is not surprising that a high preservation rate of P was observed in the results.
Growth performance is an important indicator of the quality of feed fermentation. We observed a significant increase in the ADFI in FBW-treated sows during LD. Several studies have reported that fermented feed could enhance the growth performance of the herd. Sows’ ADFI and litter weight increased when LD diets were supplemented with fermented corn and soybean meal mixed feed [31]. However, Wang et al. observed that when 5% fermented soybean meal was added to the basal diet from GD 85 to weaning, the weaning litter weight and mean BW were not affected [32]. Various potential factors might result in these discrepancies. One factor is the ingredients in the supplementation. Wang et al. used soybean meal as fermented feed, whereas the products used in our study were mixed, including bean dregs and wheat bran. Another factor was the difference in probiotics. We used L. acidophilus, S. cerevisiae, B. subtilis, and E. faecalis to produce fermented feed in the present study. In contrast, Wang et al. used B. subtilis, Hansenula anomala and Lactobacillus casei [32]. Different probiotic combinations can produce different proteases that can influence the absorption and digestion of nutrients. This may improve the overall protease activity of the fermented feed, promote the growth of fermentation microorganisms, improve the content of organic acids, reduce pH, inhibit the growth of harmful microorganisms and improve the quality of FBW [33,34]. Furthermore, the number and weight of piglets after cross-sending may also affect the piglet weaning weight. In addition, we observed that the 9% FBW-treated sow ADFI was lower between LD8-LD14 and LD15-LD19 than the 6% FBW-treated sow ADFI according to quadratic analysis. This may be because too much insoluble fiber can shorten the residence time of the chyme in the intestine, and because too much soluble fiber can adhere to the surface of the chyme to form a nutrient barrier, which is unfavorable for the digestion of nutrients, in turn, affecting the appetite of sows [35].
The quality of colostrum and the growth of newborn piglets largely depend on the nutrient intake and utilization of sows. In the current study, the decreased urea content in colostrum reflects the increased nitrogen utilization in sows. The growth performance and health conditions of newborn piglets mainly depend on the quality and quantity of colostrum and milk from sows. Therefore, the elevated litter weight may have resulted from elevated milk yield. Nutrient utilization, especially energy and protein absorption, during LD affects the milk yield of sows [36]. These results showed that the sows supplemented with FBW absorbed more energy and protein than sows in the CON treatment by increasing the sows’ ADFI and apparent digestibility; then, the sows produced more milk for the piglets.
BUN, a waste byproduct of protein breakdown, is an indicator used to assess amino acid balance and protein metabolism status. A good balance of amino acids in the diet could reduce the content of BUN [37]. In the present study, supplementation with FBW reduced the serum BUN concentration in sows and piglets on the weaning day, demonstrating that supplementation with FBW could improve the efficiency of protein utilization in sows. The significant change in the content of urea in colostrum also confirms this. This may be due to the high content of acid-soluble protein in the FBW, which animals more easily absorb. This finding was consistent with the TP status in piglets on the weaning day, in which supplementation with FBW significantly increased the concentration of serum TP in piglets on the weaning day. However, this study showed that the treatment sows had a significantly lower TP content than CON sows on the weaning day. We speculate that FBW-treated sows consumed more energy and protein to produce milk. The serum TP and BUN concentrations in sows at birth were not significantly affected by FBW supplementation. The duration of supplementation may determine its influence.
The fiber content in the diets greatly affected the intestinal activity of sows after farrowing. In the present study, the results showed that supplementation with FBW helped the intestine to avoid extended constipation. This may be due to the fiber from wheat bran and bean dregs in the treatment group. In the three weeks of LD, the sows in the treatment group always had higher fecal scores than the CON group. The high fecal score values indicate that the intestine was more active during LD. The study reported that a high-fiber diet could decrease extended constipation during the perinatal period by promoting intestinal activity [38]. The fecal scores of sows were lowest at birth and rose gradually until the end of this experiment. The sows in the treatment group recovered good intestinal activity sooner than those in the CON group.
FBW significantly reduced fecal E. coli counts in all treatment groups. E. coli is a necessary factor for healthy intestinal microflora of sows and contains many pathotypes that lead to various diseases. The decrease in E. coli could reduce the disease risk of sows. Meanwhile, elevated levels of E. coli and Clostridium were found in the intestine of constipated patients [39,40]. A reduction of Clostridium enterica improves constipation symptoms [41-43]. The high content of fiber and live bacteria in FBW may be responsible for changing the flora in sow feces. The decrease in E. coli and Clostridium may have contributed to stimulating intestinal activity [44], which promotes better physical condition and production performance of lactating sows.
CONCLUSION
This study demonstrated that dietary supplementation with FBW during late GD and LD increased ADFI and protein utilization and attenuated constipation in sows, which increased milk yield and piglet growth performance. FBW also improved fecal scores and decreased the content of pathogenic bacteria in feces. These findings suggest that supplementation with FBW helped improve sow production performance, and 6% FBW is recommended as a suitable dose for the best product performance in sows and piglets.