INTRODUCTION
Muscle food (meat), derived from skeletal muscles, which constitute the body of livestock, is an example of nutritionally excellent food. Different meat types not only differ in terms of nutritional characteristics [1,2] but also in terms of sensory and quality properties [3-5]. Reportedly, these nutritional characteristics as well as sensory and quality properties are very closely related to the meat constituents, histochemical characteristics, and muscle cell (fiber) characteristics [4,6,7]. Different meat types have considerably different nutritional and physicochemical properties depending on the species, breed, and muscle type. Further, the contribution of muscle fiber characteristics to these differences is relatively high [3,4]. Previous studies have also provided substantial evidence that meat quality changes during aging or refrigeration and quality deterioration accompanying freeze–thaw processes are associated with the composition of the muscle fibers constituting meat [8-10].
Among the various factors responsible for the differences in nutritional and physicochemical properties observed between different meat types, the characteristics of the muscle fibers comprising the meat has been identified as the most fundamental factor in this regard [4]. This correlation is well established [4,7]; thus, muscle fiber characteristics not only offer the possibility to predict the physicochemical characteristics of specific muscle types but also allow the prediction of the quality of aged and frozen-thawed meat [8-11]. However, given that the bodies of livestock comprise several muscle types, it is challenging to identify and compare the muscle fiber characteristics of the different muscle types simultaneously. For this reason, most studies conducted in recent decades have been focused on the muscle fiber characteristics of specific muscles [3,4,7,12].
Therefore, in this study, we aimed to enhance understanding regarding the differences in physicochemical properties between different muscle types (or meat cuts) via comparative review of the results of previous studies. Further, in this study, we suggest several strategies for improving the aging or storage quality of meat based on differences in the relationship between muscle fiber characteristics and meat physicochemical properties. Further, we focused on porcine skeletal muscles, which relatively, has been considerably investigated.
MUSCLE FIBER CHARACTERISTICS
Morphologically, the muscle fibers (myocytes) that make up skeletal muscles, which are also multinucleated cells, have long cylindrical shapes with diameter approximately10–100 µm and length in the order of hundreds to thousands of micrometers [13]. Further, the outer membrane of muscle fibers, composed of hundreds of myofibrils, is surrounded by sarcolemma (endomysium) [14], and their constituent organelles include nuclei, the sarcoplasmic reticulum, which stores Ca and functions in Ca secretion and retrieval, and mitochondria, which are mainly involved in energy metabolism. They also contain motor endplates and transverse canaliculi, which are responsible for transmitting motor stimuli, capillary vessels, which transport energy sources and oxygen, and adipose cells, which are often distributed within the muscle fibers [13,14].
Additionally, myofibrils are composed of two filament types: actin filaments (or thin filaments), which are mainly composed of actin, troponin complex (I, C, and T), and tropomyosin and myosin filaments (or thick filaments), which are mainly composed of myosin [15]. The kinetic energy of the muscle fiber is generated by the contraction-relaxation mechanism (twitch), which occurs through the combined and separate action of the two (actin and myosin) filament types. The contraction-relaxation speed of the muscle fiber, the energy source required during contraction-relaxation, and the activity of myosin ATPase, which hydrolyzes energy (ATP) varies depending on the muscle fiber type [13-15].
Muscle fibers are classified into several types according to their metabolic and contractile properties [12]. The energy source required for the contraction-relaxation mechanism is mainly derived from oxidative or glycolytic energy generation systems. Specifically, muscle fibers that predominantly use energy sources that require oxygen within mitochondria are called oxidative muscle fibers, whereas those that primarily use energy generated via glycolysis are called glycolytic muscle fibers [12,16]. They can also be classified according to their contractile properties as slow-twitch or fast-twitch. Unlike slow twitch muscle fibers, fast twitch muscle fibers can quickly and strongly generate kinetic energy; however, they easily become fatigued [12,16,17].
Additionally, muscle is a complex tissue composed of several muscle fiber types with different metabolic and contractile characteristics. Thus, its physiological and kinetic characteristics are determined by the architecture and characteristics (such as size, composition, and density) of its constituent muscle fibers [3,13-15]. Previous studies have provided substantial evidence that the physicochemical properties of livestock muscles differ between muscles (or meat cuts) depending on the muscle fiber characteristics observed when the muscles are converted into meat following slaughter [4,7]. Specifically, muscle fiber types that rely on oxidative metabolism have relatively large number of mitochondria, hence a higher content of myoglobin, a protein that transports oxygen within the muscle. For this reason, muscles with a relatively high ratio of oxidative muscle fibers appear redder than their counterparts with a lower oxidative muscle fiber proportion. Conversely, in glycolytic metabolism-dependent muscle fiber types, glycogen remains for a certain period (up to 48 h postmortem, depending on the animal species and muscle type) after slaughter and is used in post-mortem metabolism. This process is accompanied by the accumulation of hydrogen ions in the muscles and a decline in pH. Therefore, muscles with a high proportion of glycolytic muscle fibers have a high post-mortem metabolic rate and a low ultimate pH, which result in meat with poor water-holding capacity, color, tenderness, and protein solubility [4,7].
To analyze muscle fiber characteristics, it is first necessary to classify the muscle fibers. A typical strategy in this regard is to use the metabolic and contractile characteristics of each muscle fiber type. One muscle fiber typing method that was published decades ago and remains currently in use is based on differences in myosin ATPase activity [18,19]. The key to successfully classifying muscle fibers based on this method is to biopsy livestock muscle or collect samples within approximately 1 h after slaughter (before myosin ATPase activity disappears) and freeze them to minimize the degradation. This classification method is based on the fact that myosin ATPase activity in each muscle fiber type shows a different level of lability to different pH ranges (acidic and alkaline) [18,19]. Thus, using this method, it takes less than 1 h to stain muscle fibers, and given that the staining procedure is relatively simple, this method is widely used. Furthermore, using this method, muscle fibers are classified into the slow, fast, and intermediate types (type I, IIB, and IIA, respectively) as previously demonstrated [7,18,19] (Fig. 1A).
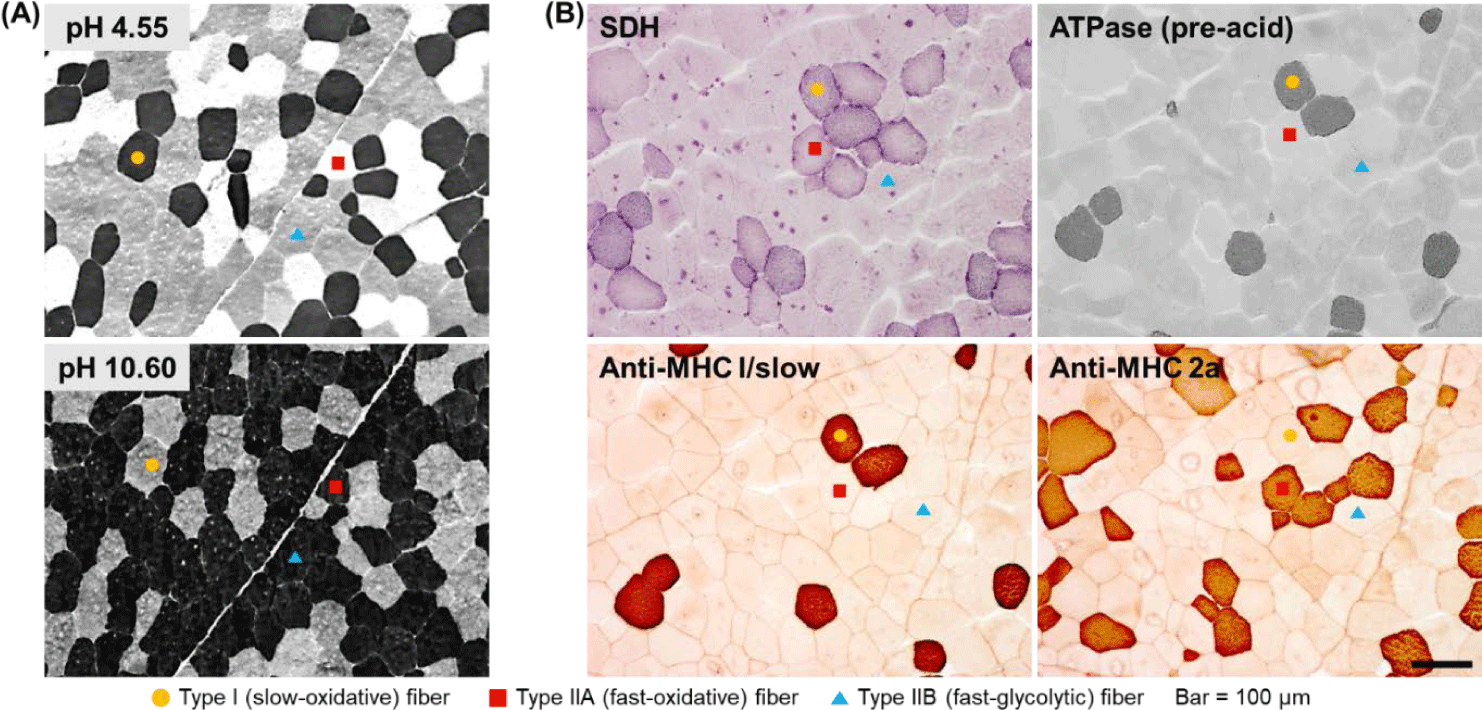
Although the use of difference in myosin ATPase pH lability is simple and convenient, it has the disadvantage that it does not offer the possibility to classify fast-type muscle fibers in greater detail. In other words, several muscle fibers classified as fast type using this method still show different metabolic characteristics (oxidative vs. glycolytic), but cannot be distinguished further. Therefore, another strategy to distinguish muscle fibers is to make use of differences in the activities of enzymes involved in oxidative metabolism, of which succinic dehydrogenase (SDH) is a representative example [20]. There is further evidence to support the use of differences in both myosin ATPase and SDH activities as a more accurate and detailed strategy for classifying muscle fibers [12,21]. Thus, rather than classifying muscle fibers as fast or slow or as oxidative or glycolytic, it is possible to more accurately express the characteristics of each muscle fiber type by classifying them as slow-twitch/oxidative (SO), fast-twitch/oxidative (FO), and fast-twitch/glycolytic (FG) as previously reported [12,20] and shown in Fig. 1B.
Another strategy for classifying muscle fiber types is to analyze the distribution of myosin isoforms, specifically, myosin heavy chain and light chain isoforms (Fig. 1B). Thus, muscle fiber types can be divided into four or more types based on the distribution of myosin heavy chain isoforms or the combination of the distributions of myosin heavy-chain and light-chain isoforms [17,21]. Further, immunohistological methods using several commercially available antibodies offer the possibility to classify muscle fiber types into as many as seven or eight types [21]. In a previous study on pig skeletal muscles, muscle fibers were classified into six types and the effects of the physicochemical properties of the constituent muscle fibers on the characteristics of pork loin meat were reported [22]. In situ hybridization (ISH) is another strategy for typing muscle fibers [12]. Basically, ISH is primarily used to determine the location of specific nucleic acid sequences in chromosomes or tissues. Further, it involves the use of mRNA obtained from oligonucleotides and RNA probes followed by analysis using microscopy [23]. Notably, types I, IIA, IIX, and IIB porcine skeletal muscles have been previously distinguished using this method [12,24].
Taken together, muscle fibers are classified into several types depending on their contractile and metabolic characteristics, and given that each muscle type has distinct characteristics, the physicochemical properties of different meat cuts vary depending on the characteristics of their constituent muscle fiber types (composition, size, etc.). Further, there are several methods for typing muscle fibers, with each method having its own characteristics and depending on the purpose of a given study, different methods or multiple methods can be used in parallel.
COMPARISON OF MUSCLE FIBER CHARACTERISTICS BETWEEN DIFFERENT TYPES OF PORCINE SKELETAL MUSCLES
Muscle fiber characteristics generally include muscle fiber size (cross-sectional area, diameter, and perimeter), relative area or numerical composition, and density (number of muscle fibers distributed per unit area). Over the past 30 years, studies have been conducted to determine the muscle fiber characteristics of several animal species (cattle, pig, chicken, goat, duck, etc.), breeds (Yorkshire, Berkshire, Landrace, Duroc, Tamworth, cross breeds, etc.), sex, and muscle type. Table 1 summarizes representative results in this regard for porcine skeletal muscles [3,6,9,12,25-34].
Breed | Sex | Age (day) | Weight (kg) | Muscles | Typing method | Muscle fiber characteristics1) | References | ||||
---|---|---|---|---|---|---|---|---|---|---|---|
Type | CSA | Area % | Number % | Density | |||||||
Yorkshire | Female | 170 | (L) 100 | M. longissimus thoracis et lumborum | ISH | I | - | - | 10.9 | - | [12] |
I/IIA | - | - | 0.1 | - | |||||||
IIA | - | - | 6.7 | - | |||||||
IIA/IIX | - | - | 0.3 | - | |||||||
IIB | - | - | 62.3 | - | |||||||
IIX | - | - | 17.5 | - | |||||||
IIX/IIB | - | - | 2.2 | - | |||||||
mATPase | I | - | - | 11.0 | - | ||||||
IIA | - | - | 7.0 | - | |||||||
IIB | - | - | 82.0 | - | |||||||
M. rhomiboide | ISH | I | - | - | 68.0 | - | |||||
I/IIA | - | - | - | - | |||||||
IIA | - | - | 12.0 | - | |||||||
IIA/IIX | - | - | - | - | |||||||
IIB | - | - | - | - | |||||||
IIX | - | - | 20.0 | - | |||||||
IIX/IIB | - | - | - | - | |||||||
mATPase | I | - | - | 68.0 | - | ||||||
IIA | - | - | 12.0 | - | |||||||
IIB | - | - | 20.0 | - | |||||||
LYD | Male Female | 185 | (L) 105 | M. longissimus thoracis | mATPase | I | 4,226 | 12.5 | 12.7 | 29.7 | [25] |
IIA | 4,204 | 9.4 | 9.9 | 22.9 | |||||||
IIB | 4,624 | 78.1 | 77.4 | 183.4 | |||||||
Yorkshire | Male | - | (L) 101 | M. longissimus thoracis et lumborum | mATPase | I | 2,376 | 6.8 | 9.6 | - | [26] |
IIA | 1,751 | 3.3 | 6.2 | - | |||||||
IIBr | 2,483 | 7.5 | 10.0 | - | |||||||
IIBw | 3,666 | 82.4 | 74.3 | - | |||||||
SDH | I | 2,415 | 6.5 | 9.5 | - | ||||||
IIA | 1,868 | 3.5 | 6.6 | - | |||||||
IIBr | 2,616 | 7.5 | 10.0 | - | |||||||
IIBw | 3,940 | 82.5 | 73.9 | - | |||||||
Berkshire | Male Female | 172.7 | (C) 77.75 | M. longissimus thoracis et lumborum | mATPase | I | - | 7.5 | 10.7 | - | [27] |
IIA | - | 5.8 | 9.0 | - | |||||||
IIB | - | 85.0 | 80.3 | - | |||||||
Landrace | (C) 77.75 | I | - | 6.2 | 9.1 | - | |||||
IIA | - | 5.6 | 9.4 | - | |||||||
IIB | - | 88.2 | 81.5 | - | |||||||
LYD | (C) 81.85 | I | - | 5.4 | 8.1 | - | |||||
IIA | - | 7.1 | 12.4 | - | |||||||
IIB | - | 87.5 | 79.4 | - | |||||||
Yorkshire | (C) 75.4 | I | - | 6.8 | 8.9 | - | |||||
IIA | - | 6.4 | 10.6 | - | |||||||
IIB | - | 86.8 | 80.5 | - | |||||||
LYD | Male | - | (C) 78.5 | M. longissimus thoracis | IHC | I | 2,743 | 6.2 | 12.9 | - | [9] |
IIA | 3,052 | 3.4 | 6.1 | - | |||||||
IIA/IIX | 4,765 | 0.4 | 0.6 | - | |||||||
IIB | 6,114 | 68.1 | 63.1 | - | |||||||
IIX | 6,869 | 17.6 | 14.6 | - | |||||||
IIX/IIB | 8,251 | 4.4 | 3.1 | - | |||||||
M. psoas major | I | 2,375 | 18.5 | 23.5 | - | ||||||
IIA | 2,166 | 20.4 | 28.1 | - | |||||||
IIA/IIX | - | - | - | - | |||||||
IIB | 4,191 | 32.4 | 23.2 | - | |||||||
IIX | 3,409 | 28.8 | 25.2 | - | |||||||
IIX/IIB | - | - | - | - | |||||||
M. semimembranosus | I | 3,055 | 6.3 | 12.0 | - | ||||||
IIA | 3,011 | 4.7 | 8.6 | - | |||||||
IIA/IIX | 6,631 | 2.9 | 2.6 | - | |||||||
IIB | 6,997 | 79.3 | 65.4 | - | |||||||
IIX | 3,634 | 7.2 | 11.4 | - | |||||||
IIX/IIB | 4,520 | 2.3 | 2.9 | - | |||||||
M. semitendinosus | I | 4,866 | 23.9 | 26.7 | - | ||||||
IIA | 4,468 | 14.1 | 17.0 | - | |||||||
IIA/IIX | 4,992 | 1.6 | 1.7 | - | |||||||
IIB | 6,282 | 29.7 | 25.7 | - | |||||||
IIX | 5,407 | 24.8 | 24.7 | - | |||||||
IIX/IIB | 7,479 | 6.0 | 4.3 | - | |||||||
Berkshire | - | - | (L) 110 | M. longissimus thoracis et lumborum | mATPase | I | 3,302 | 6.5 | - | 20.0 | [28] |
IIA | 2,801 | 9.4 | - | 34.0 | |||||||
IIB | 4,398 | 84.1 | - | 194.0 | |||||||
Duroc | I | 3,025 | 6.1 | - | 20.0 | ||||||
IIA | 3,014 | 9.1 | - | 30.0 | |||||||
IIB | 5,133 | 84.9 | - | 170.0 | |||||||
Landrace | I | 2,933 | 6.5 | - | 22.0 | ||||||
IIA | 2,781 | 7.6 | - | 27.0 | |||||||
IIB | 4,987 | 86.0 | - | 177.0 | |||||||
Yorkshire | I | 3,031 | 6.9 | - | 23.0 | ||||||
IIA | 2,698 | 8.3 | - | 31.0 | |||||||
IIB | 5,050 | 84.8 | - | 173.0 | |||||||
KNP and Landrace | Male Female | 203 | (C) 89.2 | M. longissimus thoracis et lumborum | mATPase | I | 3,877 | 9.1 | 12.1 | - | [6] |
IIA | 3,989 | 8.3 | 11.3 | - | |||||||
IIB | 4,843 | 80.8 | 77.2 | - | |||||||
Berkshire | Male | - | - | M. longissimus thoracis et lumborum | IHC SDH ISH | I | - | 9.8 | 10.19 | - | [24] |
IIA | - | 4.0 | 3.61 | - | |||||||
IIB | - | 54.8 | 55.70 | - | |||||||
IIX | - | 29.8 | 29.96 | - | |||||||
M. psoas major | I | - | 11.9 | 12.31 | - | ||||||
IIA | - | 10.6 | 10.20 | - | |||||||
IIB | - | 41.7 | 42.58 | - | |||||||
IIX | - | 41.6 | 41.69 | - | |||||||
Duroc | M. longissimus thoracis et lumborum | I | - | 14.5 | 11.40 | - | |||||
IIA | - | 3.4 | 4.03 | - | |||||||
IIB | - | 52.8 | 52.36 | - | |||||||
IIX | - | 29.6 | 34.71 | - | |||||||
M. psoas major | I | - | 14.7 | 8.54 | - | ||||||
IIA | - | 15.0 | 8.67 | - | |||||||
IIB | - | 39.5 | 50.62 | - | |||||||
IIX | - | 39.8 | 33.01 | - | |||||||
Tamworth | Male | - | - | M. longissimus thoracis et lumborum | IHC SDH ISH | I | - | 12.3 | 14.69 | - | [24] |
IIA | - | 5.9 | 3.16 | - | |||||||
IIB | - | 49.2 | 51.85 | - | |||||||
IIX | - | 32.3 | 29.64 | - | |||||||
M. psoas major | I | - | 12.1 | 14.62 | - | ||||||
IIA | - | 7.6 | 14.76 | - | |||||||
IIB | - | 38.2 | 40.13 | - | |||||||
IIX | - | 41.5 | 39.36 | - | |||||||
Yorkshire | M. longissimus thoracis et lumborum | I | - | 12.1 | 12.87 | - | |||||
IIA | - | 3.7 | 6.13 | - | |||||||
IIB | - | 51.3 | 49.68 | - | |||||||
IIX | - | 35.6 | 31.44 | - | |||||||
M. psoas major | I | - | 9.2 | 12.74 | - | ||||||
IIA | - | 8.4 | 7.81 | - | |||||||
IIB | - | 49.5 | 38.65 | - | |||||||
IIX | - | 33.9 | 40.71 | - | |||||||
KNP and Landrace | Male Female | 190 | (C) 79.85 | M. longissimus thoracis et lumborum | IHC | I | 4,797 | 8.6 | - | 21.6 | [29] |
IIA | 4,242 | 7.6 | - | 16.8 | |||||||
IIA/IIX | 4,494 | 3.6 | - | 5.3 | |||||||
IIB | 5,601 | 58.8 | - | 99.2 | |||||||
IIX | 6,823 | 19.5 | - | 33.5 | |||||||
IIX/IIB | 7,566 | 7.4 | - | 8.2 | |||||||
Domestic pig | Male Female | 165 | (C) 81 | M. gluteus superficialis | mATPase | I | 2,880 | 6.8 | - | - | [30] |
IIA | 3,420 | 4.0 | - | - | |||||||
IIB | 5,940 | 89.3 | - | - | |||||||
M. infra spinam | I | 4,780 | 53.0 | - | - | ||||||
IIA | 5,390 | 19.7 | - | - | |||||||
IIB | 5,780 | 27.3 | - | - | |||||||
M. longissimus thoracis et lumborum | I | 2,950 | 6.5 | - | - | ||||||
IIA | 2,750 | 3.2 | - | - | |||||||
IIB | 5,790 | 90.3 | - | - | |||||||
M. masseter | I | 2,700 | 22.5 | - | - | ||||||
IIA | 2,850 | 70.8 | - | - | |||||||
IIB | 2,450 | 6.7 | - | - | |||||||
M. semimembranosus | I | 3,050 | 6.6 | - | - | ||||||
IIA | 3,480 | 3.6 | - | - | |||||||
IIB | 6,210 | 89.8 | - | - | |||||||
Wild pig | M. gluteus superficialis | I | 4,070 | 17.9 | - | - | |||||
IIA | 3,430 | 16.4 | - | - | |||||||
IIB | 4,760 | 65.8 | - | - | |||||||
M. infra spinam | I | 4,450 | 55.3 | - | - | ||||||
IIA | 4,230 | 33.5 | - | - | |||||||
IIB | 4,740 | 11.3 | - | - | |||||||
M. longissimus thoracis et lumborum | I | 3,400 | 13.0 | - | - | ||||||
IIA | 2,900 | 17.3 | - | - | |||||||
IIB | 3,740 | 69.7 | - | - | |||||||
M. masseter | I | 3,240 | 68.0 | - | - | ||||||
IIA | 2,700 | 28.7 | - | - | |||||||
IIB | 2,410 | 3.3 | - | - | |||||||
M. semimembranosus | I | 3,890 | 16.6 | - | - | ||||||
IIA | 3,230 | 16.1 | - | - | |||||||
IIB | 3,760 | 67.3 | - | - | |||||||
Polish Large White | Female | 176 | (C) 78.8 | M. longissimus lumborum | IHC | I | 2,516 | 4.9 | 8.2 | 18.0 | [31] |
IIA | 2,485 | 9.5 | 15.0 | 33.0 | |||||||
IIB | 4,658 | 85.6 | 76.9 | 167.0 | |||||||
Puławska | Female | 198 | (C) 76.34 | I | 2,752 | 8.9 | 11.6 | 27.0 | |||
IIA | 2,547 | 11.7 | 16.3 | 38.0 | |||||||
IIB | 3,926 | 79.4 | 72.1 | 169.0 | |||||||
YLD | - | - | (C) 79.2 | M. longissimus thoracis et lumborum | mATPase | I | - | 5.7 | 8.4 | - | [32] |
IIA | - | 7.7 | 13.4 | - | |||||||
IIB | - | 86.6 | 78.2 | - | |||||||
LYD | Male | - | (C) 79.2 | M. biceps brachii | IHC | I | 2,669 | 16.7 | - | 56.5 | [3] |
IIA | 1,965 | 20.5 | - | 105.0 | |||||||
IIA/IIX | 1,802 | 3.6 | - | 17.7 | |||||||
IIB | 2,656 | 10.8 | - | 36.9 | |||||||
IIX | 2,625 | 32.4 | - | 121.9 | |||||||
IIX/IIB | 2,267 | 16.0 | - | 65.8 | |||||||
M. biceps femoris | I | 3,040 | 28.1 | - | 92.0 | ||||||
IIA | 2,192 | 14.1 | - | 65.9 | |||||||
IIA/IIX | 1,286 | 1.0 | - | 7.6 | |||||||
IIB | 2,905 | 32.1 | - | 114.0 | |||||||
IIX | 2,898 | 17.6 | - | 65.9 | |||||||
IIX/IIB | 2,019 | 7.1 | - | 29.1 | |||||||
M. diaphragm | I | 2,062 | 43.2 | - | 210.7 | ||||||
IIA | 1,654 | 33.0 | - | 200.7 | |||||||
IIA/IIX | - | - | - | - | |||||||
IIB | 2,609 | 5.7 | - | 23.2 | |||||||
IIX | 1,960 | 10.1 | - | 51.8 | |||||||
IIX/IIB | 1,859 | 8.1 | - | 43.5 | |||||||
M. gracilis | I | 4,095 | 15.9 | - | 38.9 | ||||||
IIA | 3,937 | 13.1 | - | 32.9 | |||||||
IIA/IIX | 4,462 | 1.0 | - | 2.2 | |||||||
IIB | 3,897 | 9.7 | - | 26.1 | |||||||
IIX | 4,043 | 50.6 | - | 128.7 | |||||||
IIX/IIB | 2,968 | 9.7 | - | 33.1 | |||||||
M. infrahyoid | I | 4,404 | 49.4 | - | 111.8 | ||||||
IIA | 3,981 | 33.2 | - | 83.5 | |||||||
IIA/IIX | - | - | - | - | |||||||
IIB | - | - | - | - | |||||||
IIX | 4,042 | 9.4 | - | 23.6 | |||||||
IIX/IIB | 4,284 | 8.0 | - | 19.5 | |||||||
M. longissimus thoracis et lumborum | I | 1,937 | 4.2 | - | 21.9 | ||||||
IIA | 1,107 | 11.2 | - | 102.5 | |||||||
IIA/IIX | - | - | - | - | |||||||
IIB | 1,719 | 42.2 | - | 242.8 | |||||||
IIX | 1,802 | 26.4 | - | 152.0 | |||||||
IIX/IIB | 2,010 | 15.9 | - | 79.0 | |||||||
M. psoas major | I | 4,050 | 21.5 | - | 49.8 | ||||||
IIA | 3,834 | 26.5 | - | 71.3 | |||||||
IIA/IIX | 3,399 | 2.0 | - | 5.0 | |||||||
IIB | 4,171 | 10.7 | - | 24.2 | |||||||
IIX | 3,886 | 14.5 | - | 42.3 | |||||||
IIX/IIB | 4,578 | 24.4 | - | 57.7 | |||||||
LYD | Male | - | (C) 79.2 | M. rectus abdominis | IHC | I | 3,803 | 49.4 | - | 135.2 | [3] |
IIA | 2,838 | 19.8 | - | 74.3 | |||||||
IIA/IIX | 3,312 | 2.3 | - | 6.9 | |||||||
IIB | 2,932 | 3.1 | - | 9.4 | |||||||
IIX | 3,761 | 18.1 | - | 43.9 | |||||||
IIX/IIB | 3,104 | 7.4 | - | 23.3 | |||||||
M. rectus femoris | I | 2,302 | 14.8 | - | 63.6 | ||||||
IIA | 1,791 | 21.0 | - | 113.2 | |||||||
IIA/IIX | 1,971 | 0.9 | - | 4.7 | |||||||
IIB | 2,921 | 32.7 | - | 112.3 | |||||||
IIX | 2,518 | 11.2 | - | 44.8 | |||||||
IIX/IIB | 2,902 | 18.6 | - | 65.7 | |||||||
M. semimembranosus | I | 2,757 | 15.3 | - | 57.6 | ||||||
IIA | 1,817 | 5.8 | - | 34.3 | |||||||
IIA/IIX | 1,857 | 2.7 | - | 15.1 | |||||||
IIB | 3,167 | 51.0 | - | 157.7 | |||||||
IIX | 2,268 | 22.9 | - | 102.3 | |||||||
IIX/IIB | 2,765 | 2.3 | - | 8.9 | |||||||
M. semitendinosus | I | 3,551 | 3.0 | - | 8.3 | ||||||
IIA | 2,096 | 18.5 | - | 59.7 | |||||||
IIA/IIX | - | - | - | - | |||||||
IIB | 2,736 | 32.0 | - | 116.5 | |||||||
IIX | 3,259 | 26.8 | - | 82.9 | |||||||
IIX/IIB | 3,246 | 19.7 | - | 59.2 | |||||||
M. subcapularis | I | 3,150 | 23.9 | - | 73.8 | ||||||
IIA | 2,850 | 18.6 | - | 66.0 | |||||||
IIA/IIX | 2,847 | 1.3 | - | 4.8 | |||||||
IIB | 3,569 | 16.7 | - | 46.7 | |||||||
IIX | 3,519 | 27.7 | - | 77.4 | |||||||
IIX/IIB | 3,523 | 11.7 | - | 34.8 | |||||||
M. superficialis digital flexor | I | 3,329 | 29.3 | - | 80.8 | ||||||
IIA | 2,492 | 23.1 | - | 93.6 | |||||||
IIA/IIX | 2,489 | 2.0 | - | 8.0 | |||||||
IIB | 2,355 | 18.3 | - | 72.0 | |||||||
IIX | 1,705 | 13.3 | - | 58.7 | |||||||
IIX/IIB | 2,190 | 14.0 | - | 60.2 | |||||||
M. vastus | I | 1,995 | 10.3 | - | 52.9 | ||||||
IIA | 1,577 | 17.5 | - | 113.2 | |||||||
IIA/IIX | 1,795 | 2.9 | - | 17.6 | |||||||
IIB | 2,747 | 25.4 | - | 101.3 | |||||||
IIX | 2,835 | 21.3 | - | 86.4 | |||||||
IIX/IIB | 2,750 | 22.6 | - | 81.0 | |||||||
LYD | - | - | (C) 82.5 | M. longissimus thoracis | IHC | I | 2,977 | 6.4 | 10.3 | - | [33] |
IIA | 2,709 | 5.5 | 9.6 | - | |||||||
IIB | 5,490 | 75.1 | 66.0 | - | |||||||
IIX | 4,439 | 12.9 | 13.7 | - | |||||||
LYD | - | - | (L) 132.9 | M. longissimus thoracis | IHC | I | 3,129 | 6.3 | 10.3 | - | [34] |
IIA | 2,853 | 5.3 | 9.4 | - | |||||||
IIB | 5,682 | 75.3 | 66.4 | - | |||||||
IIX | 4,895 | 13.0 | 13.9 | - |
Muscle fiber size is primarily evaluated via cross-sectional area measurements. A comparison of the muscle fiber sizes of 19 different porcine skeletal muscles is shown in Fig. 2. Among the skeletal muscles, M. diaphragm (DI) and M. vastus (VA) showed relatively small sizes regardless of the muscle fiber type, whereas M. gluteus superficialis (GS), M. gracilis (GR), M. infra spinam (IS), M. semitendinosus (ST), and M. longissimus thoracis (LT) showed larger sizes. Notably, M. longissimus lumborum (LL), LT, and M. longissimus thoracis et lumborum (LTL) were identified under the same muscle type. Further, the LTL is a very long muscle attached from the thoracic to the lumbar vertebrae. Thus, when sampling the LTL muscle, the thoracic (LT) region (ribeye cut) and the lumbar (LL) region (strip loin cut) are classified separately [35-37]. As shown in Fig. 2, LT is composed of larger muscle fibers than LL. This variation within a single muscle has also been previously reported [38]. A comparison of muscle fiber types showed a larger size for FG (types IIX, IIB, IIX/IIB, and IIBw) than for SO (type I) and FO (types IIA, IIA/IIX, and IIBr) muscle fiber types in most muscles. Generally, it has been reported that muscle fibers classified as Types I and IIA are smaller than those classified as Type IIX or IIB. However, IS, GR, and M. infrahyoid (IN) tend to have muscle fiber types with similar sizes and show muscle fiber size characteristics that are different from those of other muscles. This is because different muscle fiber sizes, depending on the muscle type, have different physiological characteristics, such as the ability to control movement at the location where each muscle is attached [3,39].
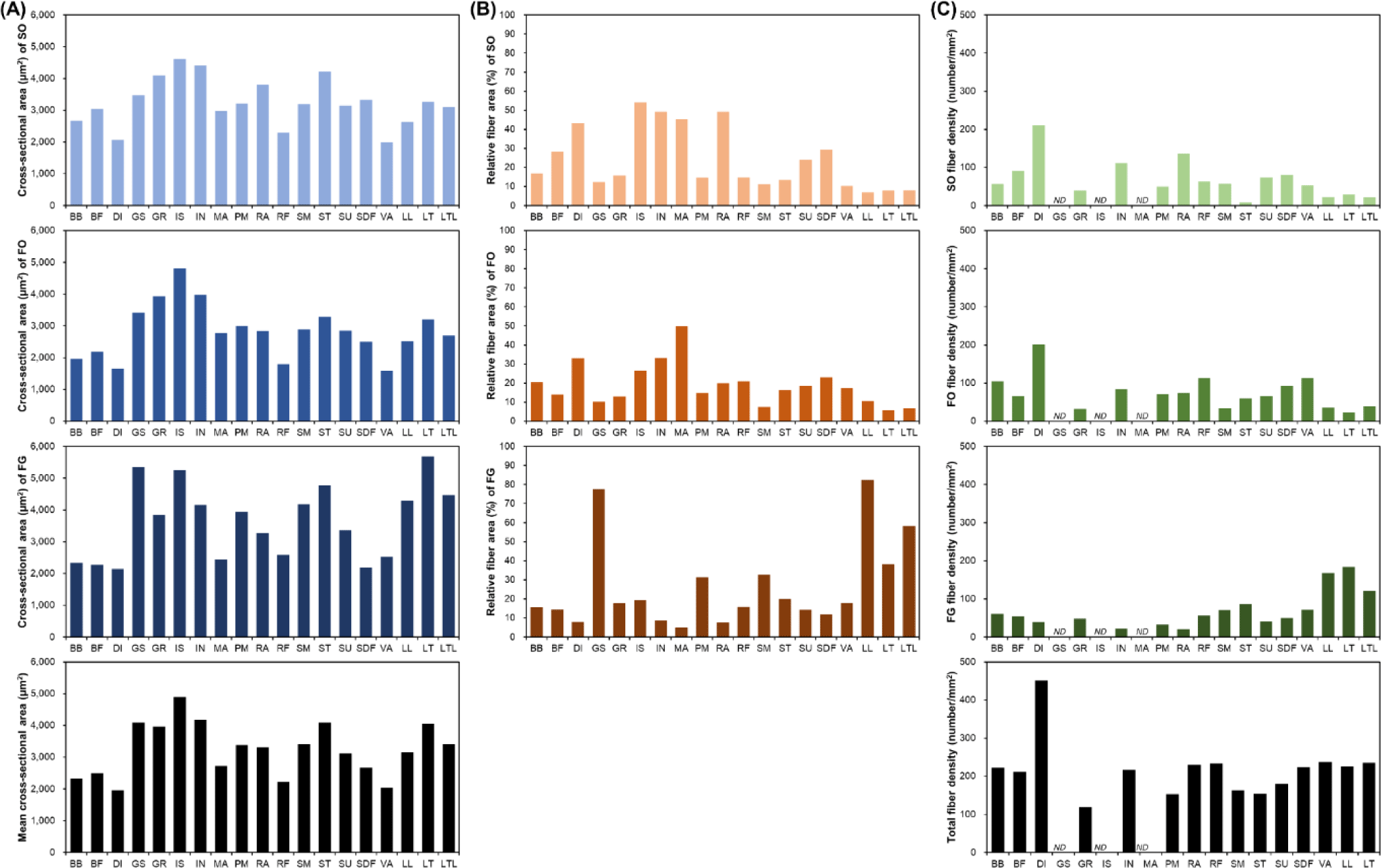
Generally, muscle fiber composition is evaluated base on area composition or number composition. However, when muscle fiber density, which represents the number of muscle fibers distributed per unit area, is also evaluated, the use of area composition is generally preferred [3,28,29]. In addition, given that area composition and numerical composition generally show similar trends, most often, the area composition alone is evaluated [3,28-30]. As shown in Fig. 2B, depending on the muscle type, muscle fiber area composition tended to be vastly different. Specifically, the proportion of SO muscle fibers in DI, IN, IS, M. masseter (MA), and M. rectus abdominis (RA) is approximately 30%–55% and that of FO muscle fibers in DI, IN, and M. semimembranosus (SM) is greater than 30% [3,30]. Further, the proportion of FG muscle fibers in these muscles tended to be less than 10% FG. Conversely, GS and LTL (including LT and LL) were identified as muscles with a low proportion of oxidative metabolism-dependent muscle fibers (SO and FO) and a high proportion of glycolytic metabolism-dependent muscle fibers (FG) [3]. They all tended to possess SO or FO compositions of approximately 10% or below. In particular, the proportion of FG in GS and LL muscles was determined to be approximately 80%. GR, M. rectus femoris (RF), ST, M. subcapularis (SU), and M. superficialis digital flexor (SDF) muscles tended to possess similar ratios of muscle fiber types.
DI showed a noticeable trend in muscle fiber density (Fig. 2C). The size of its constituent muscle fibers tended to be the smallest regardless of the muscle fiber type (Fig. 2A), and this served as the basis for predicting that it has the largest number of muscle fibers per unit area. Further, as shown in Fig. 2C, DI had the highest muscle fiber density [3]. We also observed that in DI, the densities of SO and FO tended to be much higher than that of FG. Furthermore, IN, RA, SU, and SDF showed muscle fiber density trends similar to that observed for DI. Conversely, the muscles in which the density of FG muscle fibers tended to be higher than those of SO or FO muscle fibers included LL, LT, LTL, SM, and ST muscles [3,25,28,29,31]. Although these muscles showed different densities, they all have relatively high FG densities. Meanwhile, M. biceps brachii (BB) and RF muscles tended to have similar densities for each muscle fiber type.
As mentioned above, muscle fibers can be classified according to their contractile and metabolic characteristics, which are representative muscle fiber characteristics [12]. To enhance understanding regarding differences in muscle fiber characteristics between muscles, the distribution of muscle fiber types for each muscle according to these two characteristics is summarized in Fig. 3. From this figure, it is evident that GS, LL, and LTL muscles tended to have more FG muscle fibers than other muscles. Conversely, MA, DI, IN, IS, and RA were identified as muscles with a higher proportion of SO muscle fibers. We noted that SU, SDF, and BF had similar proportions of slow-twitch and fast-twitch muscle fibers but contained relatively high proportions of oxidative metabolism-dependent muscle fibers than glycolytic metabolism-dependent muscle fibers. Another characteristic muscle is the LTL muscle. When it was categorized into the LT and LL muscles, similar ratios of slow-twitch and oxidative muscle fibers are observed; however, their fast-twitch to glycolytic muscle fiber ratio differed (LL > LT). Therefore, as previously reported, this trend implies that in addition to differences in muscle fiber properties between muscles, variations in muscle fiber properties within the same muscle type also exist [38].
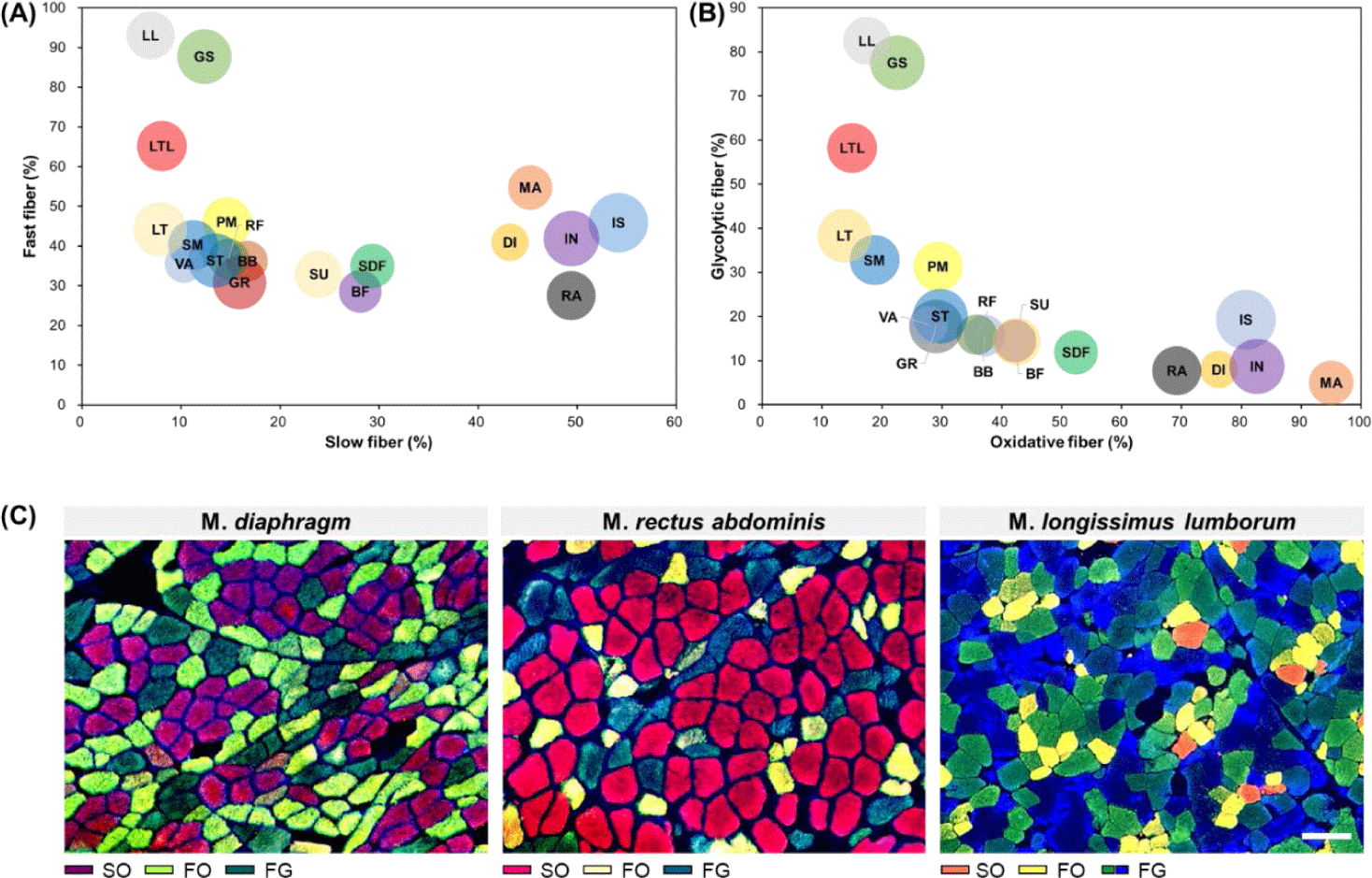
POST-HARVEST STRATEGIES FOR CONTROLLING MEAT QUALITY
Muscle fiber characteristics are reference traits that determine meat quality. They also provide basic information that can be applied to choose optimal storage or processing methods [4,40,41]. For example, when refrigerating or freezing pork, taking into consideration the histological and physicochemical characteristics of each meat part can improve meat quality or prevent quality deterioration [9,10]. In general, considerable effort is being made to identify excellent technologies for packaging and storing (aging) meat [35,40-42], and a deeper understanding of the muscle fiber characteristics of each muscle type or meat cut will make this process easier. Previous studies conducted from this perspective have shown that FG muscle fibers are more vulnerable to freezing than SO muscle fibers [9,10]. In addition, muscles with a higher SO ratio show less significant changes with age during storage. Thus, freezing should be avoided for muscles with a high proportion of FG muscle fibers, and muscles with a high proportion of SO muscle fibers do not need to be ripened for long durations [8]. In other words, regardless of the meat species, SM and ST muscles, which have a relatively higher proportion of FG muscle fibers than other muscle fiber types, show significant muscle tissue destruction and deterioration of meat quality due to freezing, so they should not be frozen unless long-term storage is necessary. On the other hand, PM (tenderloin), DI, SU, SDF, and RA muscles, where the ratio of SO muscle fibers is higher than that of FG muscle fibers, have relatively little change in meat quality even when frozen, so these muscles can be frozen if necessary.
CONCLUSION
Muscle foods (meat) are foods derived from the skeletal muscles of livestock, and determining the characteristics of their constituent muscle fibers is important for understanding differences in physicochemical properties between muscles or meat cuts. In this review, the differences between 19 major porcine skeletal muscles in terms of muscle fiber size, composition, and density were compared. We believe that our findings can be used as basic data to improve the quality of pork or to develop technologies for preventing meat quality deterioration during refrigeration, freeze–thawing, and packaging.