INTRODUCTION
Among several factors, different nutritional regimens play a significant role in sow longevity through subsequent parities. In the past decade, the reproductive performance of high-producing sows has increased dramatically due to genetic improvements [1]. Thus, there may be alterations in the nutrient requirements of high-producing sows [2] that may affect hormone levels, immune markers, and colostrum nutrients, consequently affecting the reproduction performance of sows. A small amount of mineral requirements has huge impacts on sow longevity and reproduction performance. For instance, calcium (Ca) and phosphorus levels in the diet were well-known to influence reproduction and, sow longevity [3,4]. The inclusion of 0.015% to 0.03% magnecium (Mg) in sow diets showed positive effects on reproductive parameters and serum mineral contents [5]. The beneficial effect on components of sow longevity has been exhibited by other minerals too. However, Crenshaw [6] pointed out that the positive response of dietary measures was not achieved in minimzing sow mortality because of lameness caused by manipulation of bone mineralization.
A deficiency of minerals were often found in older sows who completed three parities compared to pregnant gilts [7,8]. The amounts of minerals including Ca and Mg in sows decline as the sow get aged. Therefore, The dietary mineral supplementation to sow is important and, the supplemented minerals should be highly soluble and bioavailable to them. In addition, an adequate nutrient supply to the placenta of sows is important for proper fetal development since the placenta has the feto-maternal interface coordinating maternal nutrient supply and fetal metabolic requirements [9,10]. The levels of maternal mineral and vitamin could affect hormonal regulatory pathway which links maternal metabolism and the feto-placental unit [11]. High Ca diets have been found to suppress calcitriol levels and it alters lipid and energy metabolism of umbilical cord and placenta in the sow [12–16]. Consequently, it influences lipid and energy metabolism in the developing fetus.
The common Ca resource, calcium carbonate, is not only derived from limestone or rock, but also from the calcified skeletal remains of the red marine algae, especially species Lithothamnion. The bioavailability of the marine-derived Ca and Mg complex is high [17] and, it has a porous honeycombed vegetative cell structure. The structure of the Ca-Mg complex provides beneficial effects on its chemical usage and absorption [18]. The limestone supplemented into the basal diet as a Ca source can be lowered by replacing it with a lower level of highly soluble and bioavailable Ca source without compromising the performance of the animals. To this end, the Ca-Mg complex was evaluated in the present study.
It was hypothesized that supplementing the basal diets with marine-derived Ca-Mg complex, which has increased bioavailability for gestating gilts in their first parity as well as gestating to lactating sows in the upcoming subsequent parities may enhance the serum contents of Ca, Mg, and vitamin D in sows and their progeny and reduce stress hormones, enrich colostrum composition in sows through four parities which would eventually improve sow longevity and performance. It was also hypothesized that supplementing marine-derived Ca-Mg Complex would affect placental and umbilical gene expression essential to lipid and energy metabolism, which may eventually affect fetal growth and development. Therefore, the objective of this study was to assess the effect of marine-derived Ca-Mg complex on lipid and glucose metabolism-associated gene expression, serum metabolites, colostrum nutrient profile, and stress hormones during the four successive parities of sows.
MATERIALS AND METHODS
The experimental protocol (DK-2-1927) for this study got consent from the Animal Care and Use Committee of Dankook University, Korea.
The marine-derived Ca-Mg complex (27% Ca and 10% Mg) is a commercial product of Celtic Sea Minerals (Currabinny, Carrigaline, Co. Cork, Ireland).
As per the information of the manufacturing company, the mineral complex was harvested from seawater once the accumulated minerals in the Lithothamnion alga frond broke off and fell to the bottom of the sea. In order to produce a commercial marine-derived Ca-Mg complex for the feed industries, the mineralized fronds were sterilized, dried, and milled [19]. This mineral complex does not only contain Ca and Mg but, also includes 72 trace minerals such as strontium, manganese, selenium, copper, and zinc.
The animal trials were carried out from June 2019 to March 2021 at Dankook University swine farm, Cheonan, Korea. A total of 72 crossed-bred gilts ([Yorkshire × Landrace] × Duroc), average body weight of 181 kg were used. From the first pregnancy the gilts were randomly allocated to three groups. There were 24 gilts per treatment diet. The treatment diets were 0.3LC (basal diet – MgO − 0.3% limestone + 0.4% marine-derived Ca-Mg complex), and 0.7LC (basal diet - MgO - 0.7% limestone + 0.4% marine-derived Ca-Mg complex. Due to the limitations of the facility and workforce, 72 gilts were unable to start the trial at the same time. Thus, the gilts were subdivided into three rounds (round 1, 2, and 3) with 8 gilts per treatment per round. Each group in the rounds was continuously provided with the treatment diets up to the subsequent four parities. As recommended by National Research Council [20], sows were given basal diets to meet or exceed the nutrient requirements during gestation and lactation (Tables 1 and 2). The treatment diets were provided to sows until the end of lactation during each successive parity, which was 135 days. From the first day of gestation, the individual body weight and backfat thickness of all sows were regularly recorded to check their condition during the trial. All sows were kept in fully slatted individual farrowing crates (2.10 × 1.80 m) for 107 days of gestation. Sows freely access to water from a drinker. Sows were not offered feed on the day of parturition. The temperature inside the farrowing house was kept at around 20°C. After the farrowing, a lactation diet was gradually increased for four days, and then sows were given ad libitum intake until the weaning day of their piglets, the 21st day.
CON, Basal diet; 0.3LC, Basal diet - MgO - 0.3% limestone + 0.40% marine-derived Ca-Mg complex; 0.7LC, basal diet - MgO - 0.7% limestone + 0.40% marine-derived Ca-Mg complex.
Provided per kg of complete diet: 16,800 IU vitamin A; 2,400 IU vitamin D3; 108 mg vitamin E; 7.2 mg vitamin K; 18 mg riboflavin; 80.4 mg niacin; 2.64 mg thiamine; 45.6 mg D-pantothenic; 0.06 mg cobalamine; 12 mg Cu (as CuSO4); 60 mg Zn (as ZnSO4); 24 mg Mn (as MnSO4); 0.6 mg I (as Ca(IO3)2; 0.36 mg Se (as Na2SeO3).
CON, Basal diet; 0.3LC, Basal diet - MgO - 0.3% limestone + 0.40% marine-derived Ca-Mg complex ; 0.7LC, basal diet - MgO - 0.7% limestone + 0.40% marine-derived Ca-Mg complex.
Provided per kg of complete diet: 16,800 IU vitamin A; 2,400 IU vitamin D3; 108 mg vitamin E; 7.2 mg vitamin K; 18 mg riboflavin; 80.4 mg niacin; 2.64 mg thiamine; 45.6 mg D-pantothenic; 0.06 mg cobalamine; 12 mg Cu (as CuSO4); 60 mg Zn (as ZnSO4); 24 mg Mn (as MnSO4); 0.6 mg I (as Ca(IO3)2); 0.36 mg Se (as Na2SeO3).
Feed samples were dried in an oven (Daihan Scientific, Seoul, Korea), maintaining the temperature of 70°C for 72 hours and finely ground to pass through a 1-mm screen. The feed samples were duplicated for dry matter (DM;method 930.15), crude protein (N×6.25; method 988.05), crude fat (method 954.02), Ca (method 984.01), P (method 965.17), Mg (method 968.08), and amino acids (method 982.30E) analysis, which is followed by the procedure from the Association of Official Analytical Chemists International [21].
For the gene expression study, the tissue of the placenta and umbilical cord from five randomly selected animals per treatment were collected immediately after farrowing, and RNA was isolated from these tissues and stored at −80°C until analysis. The RNA extraction was carried out by Trizol methods using TRIzol reagent (Invitrogen, Carlsbad, CA, USA) and a Taco™Prep homogenizer (GeneReach Biotechnology, Taichung, Taiwan). Immediately after RNA extraction, quality and the quantity of total RNA were determined spectrophotometrically using an ND-1000 spectrophotometer (Nanodrop Technologies, Wilmington, DE, USA). Then, We synthesised cDNA using the ReverTra Ace qPCR RT Master Mix (Toyobo, Osaka, Japan) and a thermocycler (Bio-Rad Laboratories, Berkeley CA, USA) at the Center for Biomedical Engineering Core Facility (Dankook University, Cheonan, Korea).
The treatment-dependent gene expression levels for FABP4, CD36, SCD, FAS, SLC2A2 (primers shown in Table 3) were quantified by RT-qPCR and compared between CON and 0.3LC or 0.7LC. As a housekeeping gene, a primer pair of glycerol aldehyde-3-phosphate dehydrogenase (GAPDH) of Sus scrofa (ENSSSCG00000000694). A positive RT-qPCR reaction was detected by CFX96 Real-Time PCR System (Bio-Rad Laboratories). The relative gene expression levels were determined by 2−ddCT method. For each cDNA sample, RT-qPCR validation was performed in triplicates.
Blood samples (5 mL) were collected via jugular venipuncture from 5 sows per treatment group (i.e., three groups, n =15) and two piglets per representative sow (n =30) during each successive parity, 12 hours after parturition, into vacuum tubes without additives. The serum was centrifuged for 15 min at 3,000×g at 4°C and stored at −20°C until serum Ca, Mg, vitamin D, estrogen, cortisol, growth hormone, and prolactin concentrations. Ca and Mg in the serum were analyzed using the calorimetry method with the help of Cobas C702 (Roche Diagnostics, Mannheim, Germany). Serum vitamin D was measured using Elecsys vitamin D total chemiluminescence binding assay (Roche Diagnostics) with a Cobas 8000 e602 analyzer (Roche Diagnostics). The analysis of serum estrogen was done using an RIA kit (MP Biochemical) and detected with the help of 1479 WIZARD Gamma counter (Perkin Elmer, Massachusett, Waltham, USA). Prolactin was measured using a chemiluminescence Binding Assay (Roche Diagnostics) with a Cobas E801 analyzer (Roche Diagnostics). The growth hormone in the blood was analyzed using a growth hormone CLIA kit and detected with the help of IMMULITE ® 2000XPi (Siemens, Washington, DC, USA).
About 30 mL of colostrum were collected manually from the same mammary glands (first, third, and fifth teat on both sides) of five representative sows in each group (n = 15 per treatment) within five hours postpartum into a sterilized 50 ml falcon tube and mixed thoroughly at each parity. The collected colostrum samples were kept in an icebox, transported to the laboratory, and stored at −20°C until further analyses. Colostrum concentrations of IgG, IgA, and IgM were assayed using specific ELISA kits (Abnova, Taipei, Taiwan) and run according to the manufacturer’s instructions. The determination of fat was done using the Rose-Gottlieb method [22]. Protein, Ca, and Mg were performed according to the methods outlined by the Association of Official Agricultural Chemists International [21]; protein, using the Kjeldahl method (N × 6.32); Ca, and Mg, by the spectrophotometric method.
Saliva was collected before and after 5 hours of farrowing from 5 sows per treatment group (i.e., three groups, n =15 per treatment) during each parity. The sows were allowed to chew on the rope for 30 minutes, and the saliva from the cotton rope was squeezed into falcon tubes and stored at −20°C until analysis. The cortisol, epinephrine, and norepinephrine concentrations in saliva were determined by ELISA kit (catalog no. KA1885, KA 1882, and KA1891 respectively, Abnova, Taipei, Taiwan) as per manufacturer’s instructions.
For gene expression, data were analyzed using the MIXED procedure of SAS ( v.9.3, SAS Institue, Cary, NC, USA) for each parity separately. The data for other indices were analyzed as a 4×3 factorial arrangement. Two-way analysis of variance (ANOVA) was used to assess the parity effect (four successive parities), and three dietary treatments (with/without supplemental marine-derived Ca-Mg complex) during gestation to lactation, as well as possible interactions between these variables. The means of the data were presented with the SEM and a probability (p) level lower than 0.05 was considered a significant difference among the groups, p < 0.01 or < 0.001 indicated a highly significant difference.
RESULTS
The effect of marine-derived Ca-Mg complex on relative mRNA expression of SLC2A2, FAS, SCD, CD36, and FABP4 genes in the placenta and umbilical cord during parity 1 to 4 is presented in Fig. 1. During parity 1, the relative mRNA expression of these genes in the placenta and umbilical cord was not affected by supplementing the sow diets with Ca-Mg complex. During parity 3 and 4, the relative expression of the SCD gene was significantly downregulated (p < 0.01) in the umbilical cord of piglets born to 0.3LC and 0.7LC sows compared with the CON counterpart. From the second parity, the expression of the SLC2A2 (p < 0.003) gene was upregulated in both the placenta of 0.7LC sow and the umbilical cord of piglets born from 0.7LC sows compared with CON and 0.3LC groups, and the expression of FABP4 (p < 0.043) gene was upregulated in the placenta of 0.3LC sows compared with CON and 0.7LC sows.
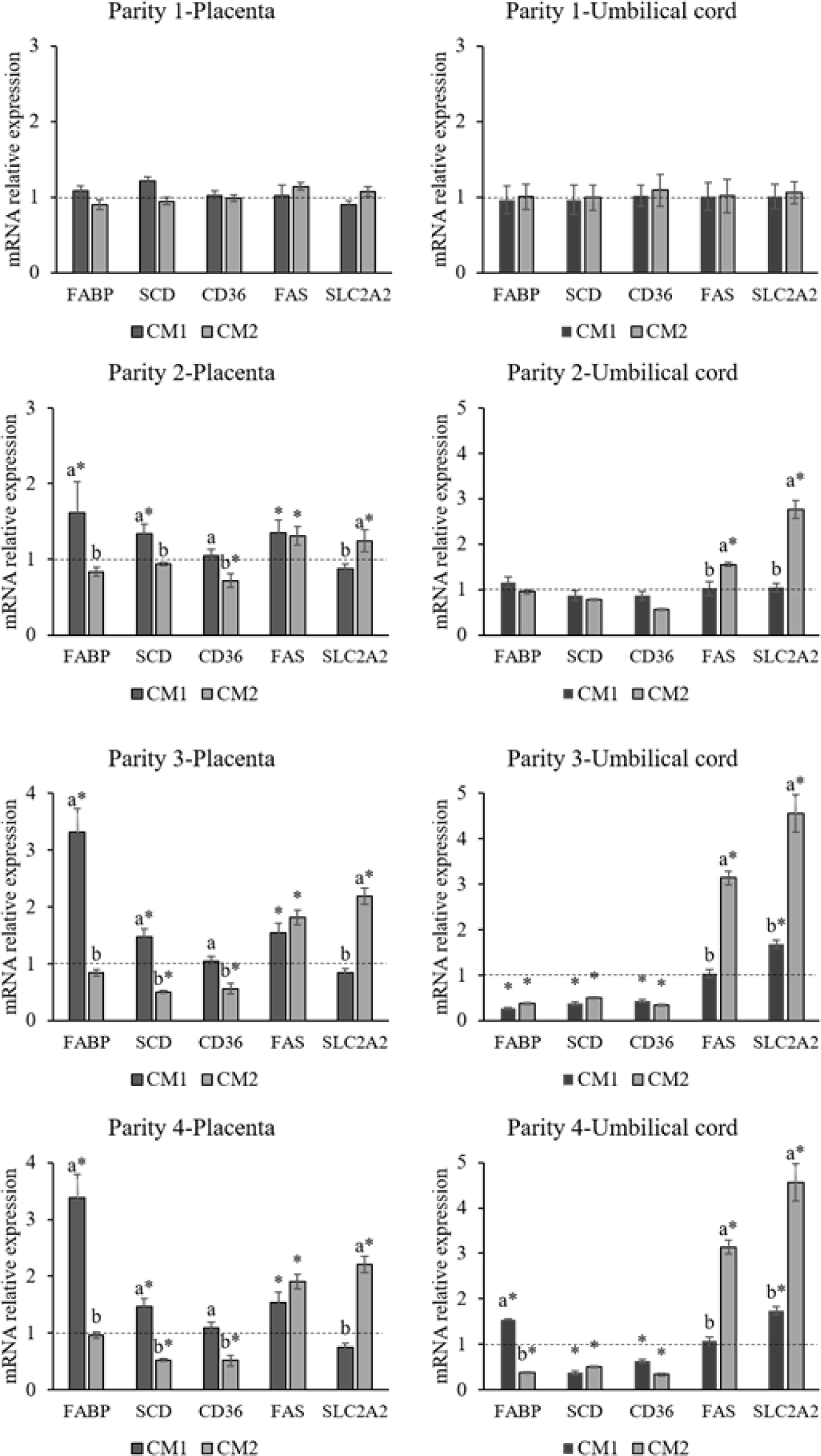
The impact of dietary supplementation of marine-derived Ca-Mg complex on the hormones, Ca, Mg, and vitamin D concentrations in the serum of sows and their piglets during four successive parities is shown in Table 4. The inclusion of Ca-Mg complex in the sows’ diet increased (p < 0.05) serum Ca and Mg concentrations in sows and their piglets regardless of parities. However, the serum vitamin D, prolactin, and estrogen concentrations in sows and serum growth hormones in piglets were not affected by the inclusion of the Ca-Mg complex in sows’ diet. The serum vitamin D concentration tends to be higher (p < 0.1) during the first parity, whereas serum prolactin and estrogen concentrations were higher (p < 0.05) during the fourth and third parity, respectively. The lower levels of serum Ca in sows were observed at the fourth parity compared with the first parity. The lowest growth hormone concentration was (p < 0.05) in the piglets born to sows during the fourth parity. The growth hormone concentration was gradually reduced by continuous parities.
CON, Basal diet; 0.3LC, Basal diet - MgO - 0.3% limestone + 0.40% marine-derived Ca-Mg complex; 0.7LC, basal diet - MgO - 0.7% limestone + 0.40% marine-derived Ca-Mg complex.
The effect of the marine-derived Ca-Mg complex on the nutrient contents of colostrum in sows during four successive parities is presented in Table 5. The inclusion of the Ca-Mg complex led to higher (p < 0.05) Ca and Mg concentrations, and trends in higher protein and IgM concentrations were also observed regardless of parities. The fat and IgA concentrations in colostrum were higher (p < 0.05) during the third and fourth parity, respectively. A trend in higher Ca concentration was observed during the third parity. However, Mg, protein, IgG, and IgM concentrations in colostrum were not affected by parity.
CON, Basal diet; 0.3LC, Basal diet - MgO - 0.3% limestone + 0.40% marine-derived Ca-Mg complex; 0.7LC, basal diet - MgO - 0.7% limestone + 0.40% marine-derived Ca-Mg complex.
The effect of supplementation of marine-derived Ca-Mg complex to sows’ diets on salivary stress hormone levels before and after farrowing during four successive parity is shown in Table 6. The inclusion of marine-derived Ca-Mg complex in sows’ diets significantly reduced (p < 0.05) cortisol, epinephrine and norepinephrine concentrations compared with CON regardless of parity in sows after farrowing, however before farrowing, except for the reduction in norepinephrine, cortisol and epinephrine concentrations were not significantly affected by the inclusion of Ca-Mg complex in the diets. Before farrowing, the epinephrine concentrations were higher (p < 0.05) during the first and second parities than the third and fourth parities. The level of norepinephrine was significantly lower in the fourth parity compared to other parities. After farrowing, the levels of epinephrine and norepinephrine during the second parity showed the highest levels and, the cortisol levels of the second and third parities was higher (p < 0.05) than the other two parities. There were no interactive effects between parity and dietary treatments on salivary stress hormones, serum parameters, and the nutrient contents of colostrum.
CON, Basal diet; 0.3LC, Basal diet - MgO - 0.3% limestone + 0.40% marine-derived Ca-Mg complex; 0.7LC, basal diet - MgO - 0.7% limestone + 0.40% marine-derived Ca-Mg complex.
DISCUSSION
Placental metabolism and transport play an influential role in fetal nutrition and metabolism since they determine the availability of nutrients to the fetus [23,24]. The compromised placental function inevitably has both short- and long-term consequences for the developing conceptus. Abnormal lipid transport during pregnancy can cause adverse impacts on the fetus. Previous studies reported that different Ca levels in the diet can affect glycolipid metabolism [25]. The aim of current study is to evaluate the partial replacement of limestone in the basal diet with marine-derived Ca-Mg complex for four successive parities. The expression levels of certain genes (FAS, CD36, FABP4, SCD) related to lipid metabolism and glucose transporter (SLC2A2) in the placenta and umbilical cord were tested. Among these genes, the mRNA expression of stearoyl-CoA desaturase (SCD) genes was downregulated in the umbilical cord of piglets born to 0.3LC and 0.7LC sows compared with CON in parity 3 and 4. There is a strong link between obesity, insulin resistance, and the SCD1 gene [26]. SCD1 catalyzes the synthesis of monounsaturated fatty acids (FAs) from saturated FAs. It has been reported that the activity of maternal and fetal SCD1 are associated with infant adiposity and negative correlations with carbohydrates and fat intakes in sows [27]. Although the specific roles of SCD1 in the umbilical cord have not been reported, the deficiency of SCD1 decreases lipogenesis and elevates lipolysis [26,27], which results in an increase of energy content. Thus, the downregulation of the SCD1 gene in the umbilical cord with the supplementation of Ca-Mg complex to sow diets potentially elevates energy transportation to the piglet, which in turn, benefits to the development of a fetus during the sow’spregnancy. Subsequently, it could increase the birth weights of the piglets. In a human study, Wang et al. [28] demonstrated that FABP4 might have an essential role in embryonic implantation and the maintenance of pregnancy, and its deregulation may result in pregnancy loss. The upregulation of FABP4 genes in the placenta from 0.3LC sow groups in the present study during the fourth parity indicated that sows were able to establish and maintain pregnancy even during the later parity stage. Additionally, FABP4 is involved in the regulation of glucose metabolism. Glucose is particularly important among the nutrients supplied by maternal circulation because it is the principal energy substrate for the developing fetus. The main regulatory factor in glucose transfer across the placenta is the activity of specific glucose transporters, the members of the GLUT family such as SLC2A. Although the SLC2A2 is important for the control of plasma membranes for glucose trnaport [29]. For gestating swine, glucose and insulin management are important to minimize embryo and fatal losses. Constant levels of glucose and insulin in sows could inhibit the hunger caused by limited feeding and, consequently, increase the physiological condition and activity of sows. The upregulation of SLC2A2 in the umbilical cord in piglets from the 0.7LC group during the fourth parity may indicate that progenies from Ca-Mg complex treated sows have reduced a risk of suppression in glucose uptake and glucose-stimulated insulin secretion.
Due to increased requirements of the developing fetal skeleton for mineralization in late pregnancy, the need for Ca, Mg, and vitamin D increases for all mammals. Therefore, with the progress in gestation, the maternal needs for Ca, Mg, and vitamin D increase more specifically in the highly prolific sows. Earlier studies on the effects of dietary Mg levels on serum Mg levels in swine are inconsistent. For instance, in a previous study by Zang et al. [5], it was reported that the serum Mg and Ca levels in farrowing sows were linearly increased, in addition to a linear increase in serum Mg levels, growth hormone levels in nursing progeny from sows or gilts receiving the supplementation of 0.015% and 0.03% Mg in their diets. In contrast, supplemental magnesium exerted an adverse effect on serum magnesium levels in growing and finishing pigs [30]. However, Nuoranne et al. [31] indicated that the serum Mg level is not a reliable index of body magnesium status. In the present study, the serum Ca and Mg concentrations in sows and their piglets were higher in gilt/sow groups receiving marine-derived Ca-Mg complex supplemented diets than those receiving CON diets. However, the serum vitamin D, in gilts/sows was not affected by Ca-Mg complex supplementation to the gestation and lactation diets in the present study. The disparity in the findings among different studies may be due to the concentration and bioavailability, as well as the single or combined supplementation of Mg or Ca to the animals.
The hormone prolactin is known to induce the mammary gland to produce milk [32]. In the present study, serum prolactin and estrogen levels in sows and the growth hormone level in the progeny born to sows receiving Ca-Mg complex-supplemented diets were not affected, indicating the supplementation of these minerals had no adverse effects on these hormone levels.
Colostrum is a complex biological fluid containing a number of nutrients and protective factors that play a pivotal role in the early gastrointestinal development of suckling piglets [33]. The colostrum intake by the suckling piglets positively influences the growth, health, and survival of piglets because it provides passive immunity derived from maternally transmitted immunoglobulin [34–36]. In the present study, the colostrum obtained from sows fed Ca-Mg complex had higher concentrations of Ca, Mg, protein, and IgM suggesting the efficacy of supplemental minerals in enhancing the minerals, protein, and immunoglobulin content of colostrum which will eventually have a positive effect in their piglets’ health and performance. The pigs receiving dietary Mg aspartate have been reported to have reduced stress hormones such as cortisol and catecholamine, and a reduction in plasma epinephrine was observed in finishing pigs receiving Mg five days prior to slaughter [37]. The findings of the present study also showed a significant reduction in salivary stress hormones (cortisol, epinephrine, and norepinephrine) after farrowing regardless of parity suggesting the effectiveness of Ca-Mg complex supplementation in calming the animals. Thus, the reduction in stress hormones after farrowing in 0.3LC and 0.7LC sows may indicate a positive effect on sow recovery which may impact subsequent farrowing positively.
The serum Mg level was significantly lower, and serum Ca and vitamin D levels tended to be low in the fourth parity sow. The levels of serum Ca and Mg were lower in the litters born to fourth parity sows compared with other parities suggesting that with the increase in parity, the mineral and vitamin levels get lower. Mahan and Taylor-Pickard [8] suggested that with the advancement in parity, the minerals stored in the body get depleted. The serum prolactin level in sows was found to be higher in fourth parity sows. In agreement with the present findings, Famer et al. [38], and Quesnel et al. [39] also demonstrated that prolactin concentration was lower in primiparous than multiparous sows at 24 h post-partum, which could be due to the increased need for the induction of higher milk production in multiparous sows due to more litter number than primiparous sows. The higher estrogen levels in the control group of the third and fourth parities in the present study may be due to the maturation of the reproductive system.
Previous studies reported variations in colostrum components, including fat, immunoglobulin, fat content, and growth factors between multiparous and primiparous sows [40,41]. For instance, colostrum fat was highest in primiparous sows, which declines as parity advances [39,42]. However, in the present study, the fat content of colostrum was the highest for the third parity sows, and the IgA level was the highest in the fourth parity sows among the other three parities. In contrast, no parity effect was observed on colostrum fat content [43] and IgA levels [42]. The protein levels in colostrum were not affected by the parity, which corroborated with Segura et al. [42]. The discrepancies in these findings are influenced by animal breed [41].
Sows in farms are regularly exposed to stressors throughout their production period, and the level of stress could be influenced by sows’ parities [44,45]. In the present study, the stress-related hormones analyzed from sows’ saliva were higher in first and second parity sows than in third and fourth parity sows. The reduction in stress hormones in sows during the third and fourth parity may be due to the ability to cope with stressors as they gain experience with them.
CONCLUSION
The inclusion of marine-derived Ca-Mg complex in the sow diet led to the downregulation of SCD gene during the third and fourth parities indicating that lipid metabolism was unaffected in the sows reducing the risk of obsessing newborns. Whereas the upregulation of FABP4 gene in the placenta of 0.3LC sows and SLC2A2 gene in the umbilical cord of piglets born to 0.7LC sows indicate that sows could establish and maintain the pregnancy even during the advanced parity stage, and their progenies had a reduced risk of suppression in glucose uptake and glucose-stimulated insulin secretion. The partial replacement of limestone in the basal diet of sows with marine-derived Ca-Mg complex significantly increased the serum Ca and Mg, colostrum Ca, Mg, protein, IgM levels, and reduced stress hormones after farrowing compared to sows fed control diet regardless of parity. However, depletion of Ca and Mg was observed in the fourth parity sows fed the control diet, and a reduction in stress hormones was observed in the third and fourth parity sows suggesting the need to continue supplementing the diet with bioavailable Ca and Mg from gilt to advanced parity to improve sow longevity and reproductive parameters and replace limestone in the sow’s diet.