INTRODUCTION
The gut microbiota plays a vital role in the physiological, metabolic, and nutritional uptake functions of its host [1]. The host usually accommodates and establishes a symbiotic relationship with the different bacterial species populating its digestive tract [2]. Further, the gut microbiota associates itself with the epithelial membrane of enterocytes to form a protective layer against pathogens [3]. Therefore, a clear definition of a “healthy” microbiota is important to help establish approaches for the modulation of its composition, which can result in improved host health and performance. In chickens, the distal part of the intestinal tract, namely the cecum and rectum, is inhabited by a greater number of microbial species than the proximal part, such as the crop, proventriculus, and gizzard [4].
A growing body of evidence suggests that diet is a major factor affecting the microbiome [5,6]. In fact, the non-digestible and unabsorbed portions of chicks’ diets can serve as available fuel for microbial growth in the gut [7]. Insoluble fibers in general are used by microbes to produce short-chain fatty acids (SCFA) via fermentation [8]. These SCFA generated are widely considered to be having health-promoting effects. For instance, studies revealed that after purification, lignin and mannan oligosaccharides could be used as potential antibiotics and growth promoters in broiler chickens [9,10]. Besides, another dietary strategy used to positively modify the intestinal bacterial population resides in the use of phenolic compounds. Polyphenols are thought to be transformed by microbes into derived metabolites which can inhibit pathogens and activate the proliferation of the same beneficial microbes [11].
Plant-derived products have been extensively evaluated as feed additives, due to their potential effects on growth and health in livestock [12–14]. Indeed, researchers have been conducting trials using non-conventional dietary ingredients in poultry farming. As a result, novel products such as pine particles have been tested as feed additives in chickens under thermoneutral and heat stress conditions [15,16]. Besides, it was highlighted that the submission of dietary fiber-rich products to different extraction techniques could enhance the bioavailability of nutrients [17]. For instance, hot water extraction was used to transform spent mushroom substrate into a valuable feed additive in dairy cows [18]. Similarly, solubles from shredded, steam-exploded pine particles (SSPP) can be obtained after submitting pine particles to hot water extraction. The resulting SSPP is primarily composed of phenolic compounds, lignin, and carbohydrates.
In this study, we attempted to thoroughly assess the cecal microbiota and growth performance in broilers supplemented with SSPP. Considering the chemical composition of SSPP, we hypothesized that its dietary inclusion in broiler diets can enhance beneficial bacterial growth, resulting in an overall “healthier” microbiota. For doing so, birds were fed SSPP-supplemented diets from 8 to 28 days old. Thereafter, parameters such as growth performances, organ indexes, plasma biochemicals, and cecal microbiota characteristics (diversity and composition) were evaluated.
MATERIALS AND METHODS
All the experimental procedures for this study were approved by the Institutional Animal Care and Use Committee of Gyeongsang National University (GNU-200916-C0057).
SSPP was prepared via the explosion of pinewood chips (2 cm × 2 cm × 0.5 cm) with steamed water at 200°C for 11.5 min. The first stage resulting particles were used in our previously published studies [15,16]. The current SSPP used in this trial was obtained after mixing particles with water (ratio of 46:100 v/w) following extraction at 80°C for 213 min. The extract was then filtered through a Whatman filter paper, grade 2 (Z177601, Sigma-Aldrich, Seoul, Korea), and stored at 4°C until use. The chemical composition of SSPP obtained was the following: 4.9% phenolic compounds, 9.2% acid-insoluble lignin, and 75.2% carbohydrates (Table 1).
A total of 323 unsexed day-old Ross 308 broiler chicks were obtained from a commercial hatchery. Following standard rearing conditions, chicks were raised in a room containing H-type battery cage assemblies with 3 tiers and 7 cages per tier. The room environmental condition was set at 34 ± 1°C and 50% relative humidity for the first 3 days and then the temperature was decreased gradually to reach 22 ± 1°C on day 28. During the first seven days, the birds were fed ad libitum with a commercial starter diet (Nonghyup Feed, Seoul, Korea) in crumbled form. From day 8, chicks (n = 216) were allocated into three different treatment groups having similar body weights. The three dietary treatments were supplemented with 0% (0% SSPP), 0.1% (0.1% SSPP) and 0.4% (0.4% SSPP) SSPP in basal diets. The composition of the basal diets is shown in Table 2. During the whole trial, feed and water were provided ad libitium, and lightning conditions were 23 h of light and 1 h of dark. Each treatment had 12 cages containing 6 birds. The length, width, and height of the cages were 90 cm × 70 cm × 45 cm respectively. Average daily feed intake (ADFI), average daily gain (ADG), and feed conversion ratio (FCR) were calculated based on weekly body weight and feed intake.
The feeds were purchased from Nonghuyp Feed (Seoul, Korea), and their compositions were provided by the company.
Trace minerals and vitamins provided per kilogram of premix: vitamin A, 12,000,000 IU; vitamin D3, 3,000,000 IU;vitamin E, 40,000 IU; vitamin K3, 2,000 IU; vitamin B1, 2,000 mg; vitamin B2, 5,000 mg; vitamin B6, 3,000 mg; vitamin B12, 20 mg; niacin, 40,000 mg; pantothenic acid, 10,000 mg; folic acid, 1,000 mg; iron, 88,000 mg; copper, 72,600 mg; zinc, 60,000 mg; manganese, 66,000 mg; iodine, 990 mg; selenium, 220 mg; cobalt, 330 mg.
On day 28, at the end of the trial, 8 birds from each treatment were randomly selected and euthanized using CO2, thereafter, blood and organ were sampled. Blood was drawn from a heart puncture and then collected into heparinized vacuum containers (#367874, BD, Franklin Lakes, NJ, USA). Plasma was obtained by centrifuging blood samples at 2,000×g for 10 min at 4°C, and was stored at –20°C for subsequent analysis. The duodenum, jejunum, ileum, liver, and spleen were sampled and weighed. Absolute and relative weights were calculated as organ indexes. In addition, the length of the duodenum, jejunum, ileum, and ceca were measured before being snap-frozen in liquid N2 and stored at –80°C for further analysis.. Plasma metabolite concentrations were measured according to the manufacturer guide using a VetTest Chemistry Analyzer (IDEXX, Westbrook, ME, USA) with a dry-slide technology [19].
The DNeasyPowerSoil 135 Kit (Qiagen, Hilden, Germany) was used to conduct the total genomic DNA of cecal samples according to the manufacturer’s protocol. The cecal samples were randomly selected from 8 birds per treatment. The DNA samples obtained were quantified using Quant-IT PicoGreen (Invitrogen, Waltham, MA, USA). Thereafter, the 16S metagenomic sequencing library was constructed for metagenomic estimation using the Herculase II Fusion DNA Polymerase Nextera XT Index Kit V2 (Illumina, San Diego, CA, USA). Illumina platform was used for sequencing the library (Macrogen, Seoul, Korea). The fastp program was then used to perform quality profiling, adapter trimming, and read filtering. Sequences within the range of 400 to 500 bp were used and paired-end reads were assembled into one sequence using FLASH (v1.2.11) software. The CD-HIT-EST program was used to determine the number of operational taxonomic units (OTUs) with a 97% sequence identity cutoff. BLAST+ (v2.9.0) program was then used to check taxonomic similarity against the reference database (NCBI 16S Microbial). Identical coverage of less than 85% was identified as not defined. QIIME software (v1.9) was used to evaluate the OTU abundance and taxonomic information of the microbes.
In the current study, the cage was considered as the experimental unit. The experiment was performed in a completely randomized design with diet as a single factor. Downstream data wrangling, analysis, and visualization were performed using different packages of the R software v.4.1.0 (R core Team, 2021). Variables were tested for the homoscedasticity and normality assumption with Levene’s and Shapiro-Wilk’s tests respectively [20]. Data following a normal distribution, namely, growth performances, organ indexes, and plasma biochemical parameters were analyzed via a one-way ANOVA, followed by a Tukey post-hoc test when a significant p-value (p < 0.05) was found.
Alpha and beta diversity metrics were calculated for the estimation of microbial community diversity. Indices such as OTUs, Chao1, Shannon, inverse Simpson, and Good’s coverage were calculated to estimate alpha diversity. Non-parametric Kruskal-Wallis test followed by a pairwise Wilcoxon rank sum test (adjusted via the Benjamini-Hochberg method) was performed to assess alpha diversity indices and relative abundance between treatment groups. Concerning beta diversity analysis, Non-Metric Dimensional Scaling (NMDS) based on Bray Curtis distance matrix was used for visualizations [21]. Furthermore, the homogeneity of multivariate dispersion among treatment groups was tested by conducting a permutational multivariate analysis of dispersion (PERMDISP) [22]. Thereafter, analysis of similarities (ANOSIM) was used to evaluate the effects of SSPP supplementation on microbiota composition variability between samples based on beta diversity distance matrices [23].
Linear Discriminant Analysis (LDA) Effect Size (LEfSe) was applied to determine the OTUs most likely to explain differences (p < 0.05; | LDA effect size | > 2.0) between control and SSPP-supplemented diets. In addition, to uncover internal interaction within microbial communities, a correlation network analysis was executed [24].
One way-ANOVA, Kruskal-Wallis, and pairwise Wilcoxon rank sum tests were conducted using base R functions. NDMS, PERMDISP, and ANOSIM were conducted with envfit, betadisper, and anosim functions of the “vegan” package. Finally, the “igraph”, and “circlize” packages were used to draw the microbial community network and chord diagram while the LEfSe algorithm was launched from the “mothur” software [25] via the R command line.
RESULTS
Table 3 presents the effects of increasing the concentration of dietary SSPP supplementation on growth performance parameters. Supplemental SSPP did not significantly affect ADG, ADFI, and FCR, regardless of inclusion level. In addition, there was no impact of SSPP supplementation on absolute and relative organ weights (Table 4). Similarly, absolute and relative organ lengths were not significantly modified by the inclusion of different SSPP levels in the diet of broilers (Table 5). As depicted in Table 6, plasma metabolites did not present any changes in their concentration regardless of the inclusion of SSPP in the diet or not.
The alpha diversity results including OTUs, Chao1, Shannon, inverse Simpson, and Good’s coverage are presented in Fig. 1. Compared to the control group (0% SSPP) a decrease (p < 0.05) in species richness was observed with low supplementation levels (0.1% SSPP). On the other side, the birds belonging to the 0.4% SSPP group presented an overall increase (p < 0.01) in species richness when compared to their 0.1% SSPP counterpart, as indicated by observed OTUs and Chao1 indices. Furthermore, the similar pattern observed in both indices was indicating that the sequencing depth obtained was sufficient. In contrast, none of the other indices (Shannon, inverse Simpson, and Good’s coverage) were showing statistical differences neither when SSPP was supplemented at 0.1% or 0.4%.
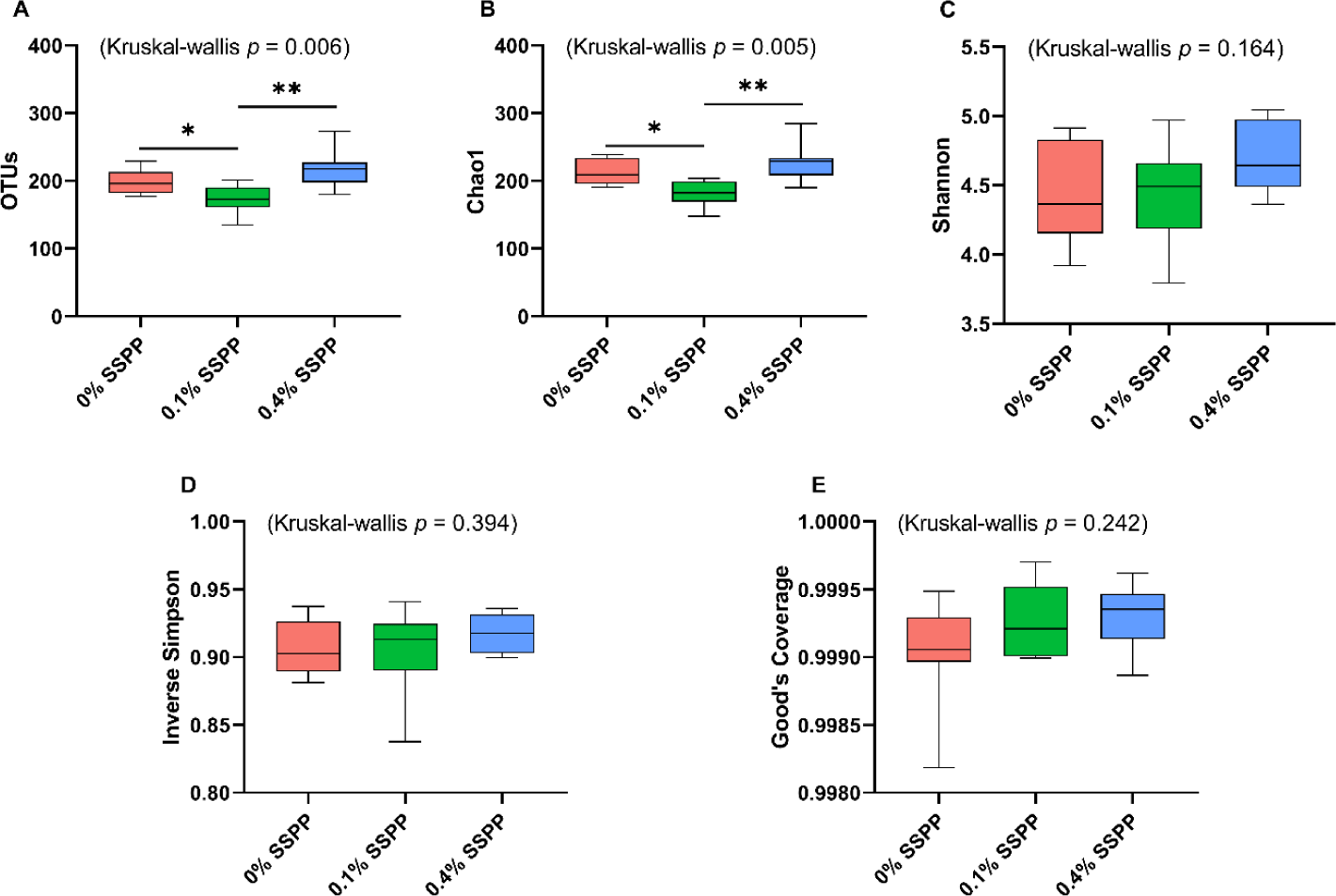
Beta diversity results are depicted in Fig. 2. In this trial, PERMDISP revealed that individual community variation was greater (p < 0.05) in the control group (0% SSPP), while lower variances were found in SSPP-supplemented groups (0.1% SSPP and 0.4% SSPP). In addition, the NMDS plot using the Bray-Curtis distance matrix showed SSPP supplementation-related clustering, with birds fed SSPP clustering closer to each other (Fig. 2B). Similarly, microbial community structures were significantly different (r = 0.31, p < 0.01) when all birds fed SSPP (0.1% SSPP and 0.4% SSPP) were compared to the control group based on ANOSIM.
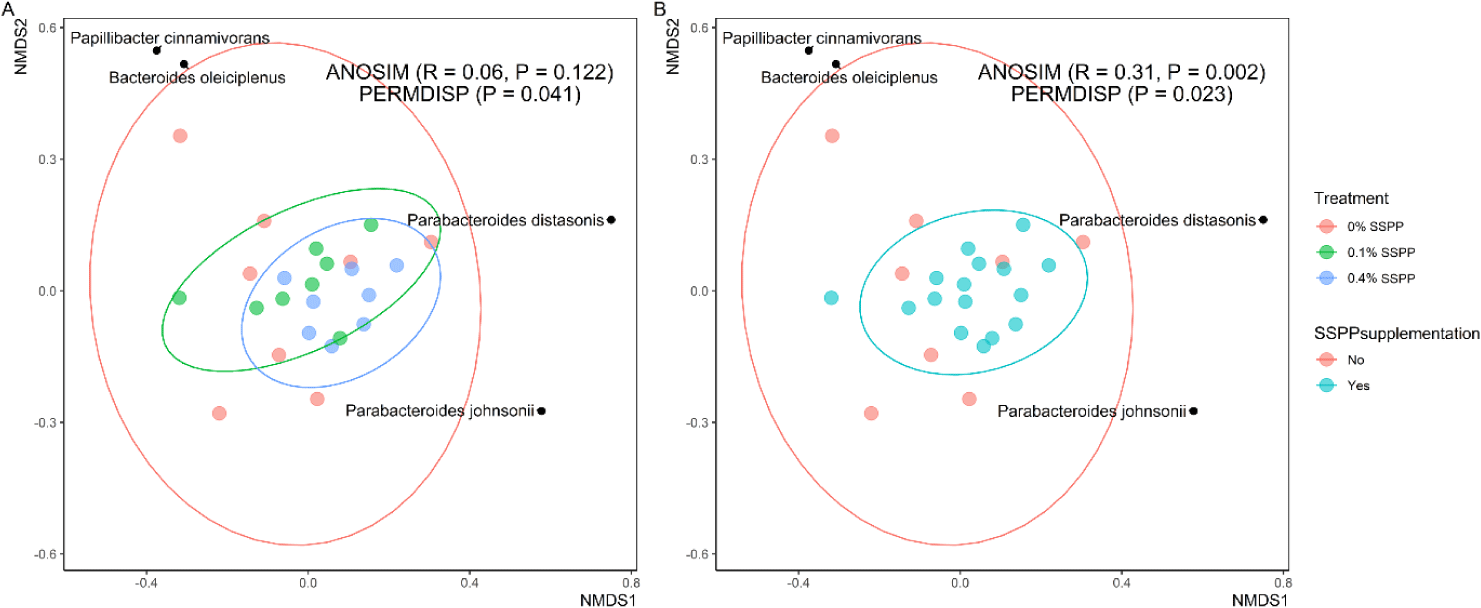
As illustrated in Fig. 3 the relative abundance of OTUs from birds’ cecal microbiota was analyzed at different taxonomic levels. Firmicutes (56.6%) and Bacteroidetes (40.2%) together were representative of the majority of the microbiome from birds’ cecal samples in the current trial (Fig. 3A). SSPP supplementation also led to an increase in Bacteroidetes relative abundance (from 35.5% to 42.7%) while concomitantly decreasing Firmicutes proportions (from 60.3% to 52.6%). Although minimal, there was a reduction in Proteobacteria relative abundance (from 3.8% to 1.2%) with the supplementation of SSPP. The dominant classes across treatment groups were Clostridia (45.4%), Bacteroidia (40.2%), and Bacilli (8.3%) (Fig. 3B). The birds supplemented with SSPP had a higher abundance of Bacteroidia and a lower abundance of Bacilli while the Clostridia abundance did not seem to be affected treatment-wise. More precisely, the relative abundances of Bacteroidia increased from 34.5% for 0% SSPP to 42.4% and 42.7% for 0.1% SSPP and 0.4% SSPP, respectively. On the other side, Bacilli proportions dropped from 11.7% to 7.4% and 5.9% for 0% SSPP, 0.1% SSPP and 0.4% SSPP, respectively. Order level microbiota analysis revealed that Eubacteriales (45.4%), Bacteroidales (40.2%), and Lactobacilliales (6.51%) which were unchanged, increased (from 35.5% to 42.7%), and decreased (from 8.5% to 5.1%) by SSPP supplementation, respectively, and accounted for the largest proportion of the microbial composition (Fig. 3C).
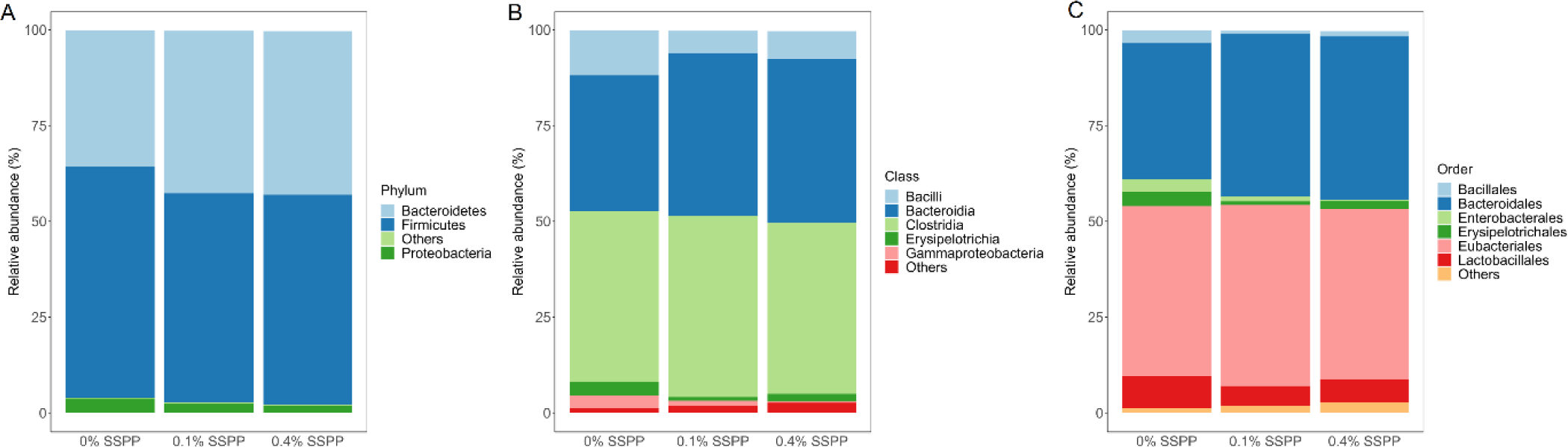
At lower taxonomic levels (family and genus), statistically, significant modifications were detected, with 0.4% SSPP group generally increasing abundance (Fig. 4). Indeed, Vallitaleaceae, Defluviitaleaceae, and Clostridiaceae had their proportion significantly increased (p < 0.05) by 0.4% SSPP when compared to either the 0.1% SSPP or 0% SSPP groups. In addition, the abundance of Clostridiaceae was also significantly higher at 0.1% SSPP in contrast to 0% SSPP. Similarly, the Vallitalea, Hespellia, Defluviitalea, Butyricicoccus and Anaerofilum genera were significantly more abundant in the 0.4% SSPP. On the other side, 0.1% SSPP led to a reduction of Defluviitalea when set against the 0% SSPP diet.
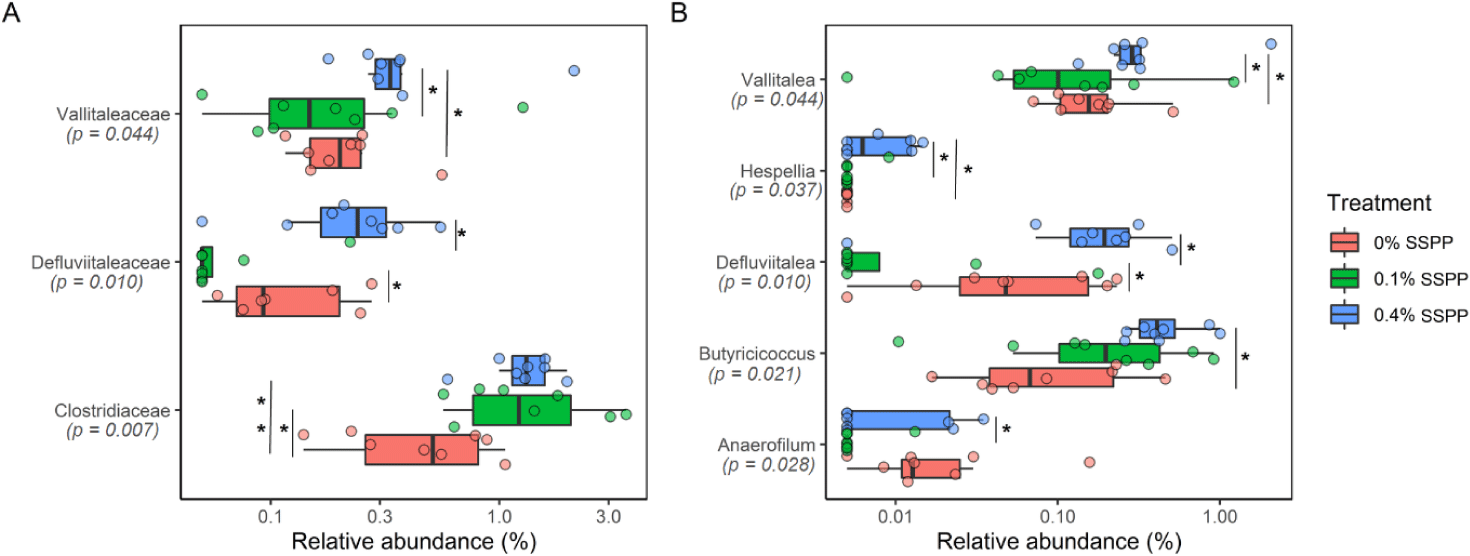
The identification of species potentially representing biomarkers of SSPP supplementation was conducted via LEfSe (Fig. 5). Ruminococcus albus, Christensella timonensis, Acetivibrio alkalicellulosi, Butyricicoccus pulliceacorum, and Anaerotignum propionicum were enriched (p < 0.05; | LDA effect size | > 2.0) in the cecum of birds fed SSPP supplemented diets Besides, LEfSe identified only Anaerofilum agile as a biomarker of the basal diet.
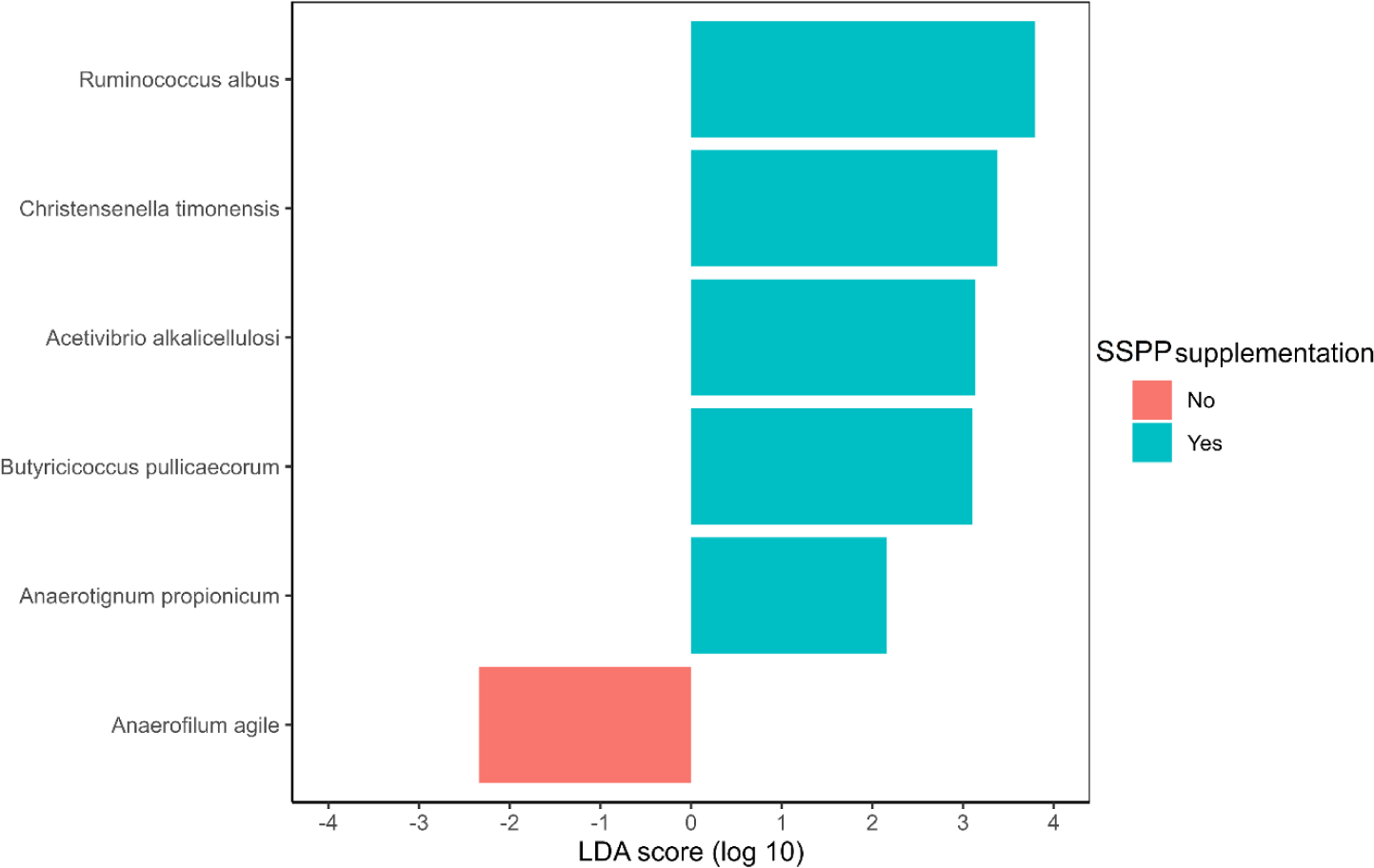
Correlation network analysis was used to detect interactions between cecal microbial species of birds (Fig. 6). Simultaneous visualization from the network graph, and chord diagram is primordial to enhance the reader’s understanding of the microbial interactions. After prevalence filtering and selecting strong and significant correlations (p < 0.05, | r |= 0.65) the correlation network obtained had 43 nodes (species) and 35 edges (interactions). There was a striking predominance of Clostridia in the network, while Bacilli and Erysipelotrichia were represented in minority. Besides, Tissirella and Bacterodia had only single individuals in the network. Bacilli appeared to be mostly connected between themselves while Erysipelotrichia were linked to Clostridia. Two interesting subnetworks could be spotted (Fig. 6A) with the first one composed of Bacilli and Clostridia while the second one regrouped Clostridia and Erysipelotrichia. Clostridia were generally positively correlated with each other. Only a few negative correlations were detected in the network and appeared to be evenly distributed among intra-classes (3) and inter-classes (4) connections.
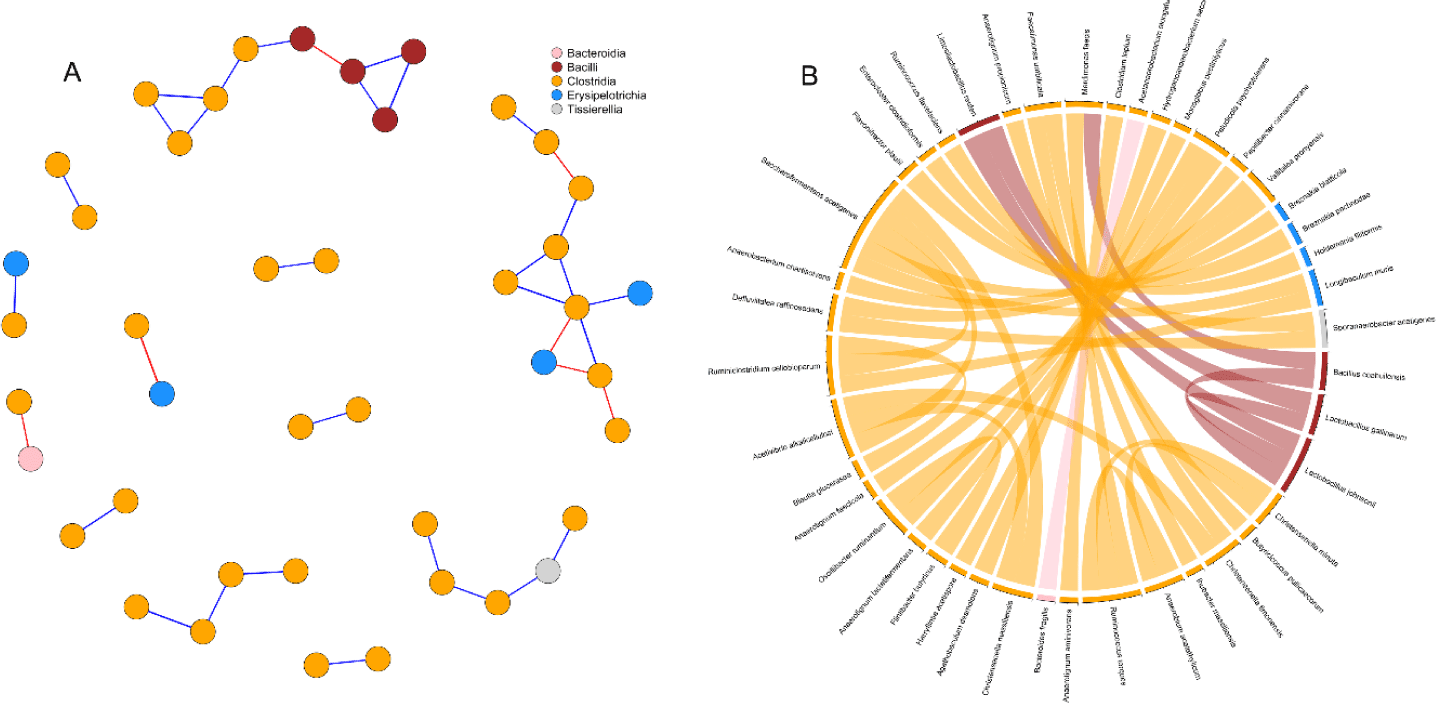
DISCUSSION
The utilization of phytogenic feed additives in animal nutrition has been the subject of recent research due to their potential role in the growth and health of livestock [26]. Among these compounds, byproducts from the wood industry are gaining popularity [27,28]. Their relatively low price and availability make them suitable for their inclusion in broilers’ diets [29]. The current study provides a detailed assessment of the growth, health, and cecal microbiota in broilers after SSPP supplementation.
There were no significant differences in ADFI, final body weight, and ADG between control and SSPP-fed birds. These results were in agreement with our previous study that evaluated the effects of other particles (from 1% to 2% of inclusion) on broilers’ growth [16]. Similarly, a 0.2% chestnut wood extract supplementation did not significantly increase growth performance in broilers [28]. On the other side, a considerable improvement was seen in ADG, feed intake, and FCR when chicks were supplemented (from 25 to 100 g/kg of feed) with dietary charcoal obtained from the oak tree [30]. Therefore, it is easily understandable that in vivo trials focusing on the supplementation of byproducts from the wood industry have inconsistent effects on poultry performances. The disparity observed in the results within studies can be explained by their setups and designs. Even though dietary wood-derived products are well known for their relatively high amount of fiber, carbohydrates, and phenolic compounds [31,32], factors such as source, extraction method, supplementation duration, and inclusion level have a greater influence on growth-related outcomes [33].
Although relatively simple to assess the organ index is a highly informative parameter that gives insights into the development status of the organ [34]. In the current study, we evaluated the relative weight and length of intestinal segments. None of the different organ indexes evaluated appeared to be affected by the inclusion of SSPP in broilers’ diets. It is already acknowledged that improvement in intestinal development leads to enhanced nutrient absorption, which is reflected by higher growth [35]. Since chicks’ body weight did not significantly differ among treatments, the observed results were therefore expected.
While our findings did not support any effects of SSPP supplementation on phenotypic parameters (growth and organ development), there was a clear indication of diet-induced microbial shifts. Other researchers also highlighted that feed additives and diet were usually associated with significant modifications in microbiome composition and diversity [36–38]. In the current trial, both the diversity and composition of the cecal microbiota were influenced by the inclusion of SSPP in the diet. SSPP supplementation significantly impacted not only richness indices (OTUs and Chao1) but also beta diversity. For example, a lower individual variation was observed in the SSPP-supplemented birds in comparison to the control birds, suggesting that regardless of the inclusion level, SSPP had the same effects on broilers’ microbial community structure. Besides, it is critical to mention that the overall variations observed between controls and birds supplemented with SSPP were mostly caused by changes in relative abundance rather than taxonomic composition. Similar findings were also reported in our previously performed trials [15,16].
In chickens, the cecal microbiota considerably increases in complexity and composition in comparison to the proximal part of the gastrointestinal tract [39]. Firmicutes and Bacteroidetes are the two dominant phyla usually followed by Proteobacteria and Actinobacteria [40]. Bacteroidetes are gram-negative bacteria providing their host energy in the form of acetate and propionate through the fermentation of originally indigestible polysaccharides [41]. On the other side, Firmicutes are gram-positive bacteria and the main producer of butyrate [42]. In the current study, SSPP-supplementation was shown to induce an augmentation in Bacteroidetes abundance concomitant with a reduction in Proteobacteria proportions. Like most plant-based bioproducts, SSPP contains considerable amounts of lignin, phenolic compounds, and carbohydrates. It was suggested that polyphenols ingestion can lead to modifications in Firmicutes to Bacteroidetes ratios by mainly supporting the proliferation of Bacteroidetes [11]. Similarly, Bacteroidetes proportions were found to increase in cecal and cloacal samples from chickens fed polyphenol-rich mulberry byproducts [43]. Healthy broiler individuals tended to have a similar proportion of Firmicutes and Bacteroidetes while a higher Firmicutes to Bacteroidetes ratio was correlated with obesity and body fat accumulation [44]. Furthermore, an increase in Proteobacteria abundance may lead to compromised growth and inflammatory reactions [45,46]. Although SSPP supplementation is likely to be associated with overall health improvement in broiler chicks, the relatively short duration of inclusion (21 days) in the current trial might have limited the effectiveness of SSPP as we only saw a slight improvement in birds’ body weight. Therefore, further studies evaluating the long-term supplementation of SSPP will be of great interest.
In agreement with other studies [47,48], the abundance of bacteria belonging to families Clostridiaceae, Defluviitaleaceae, and Vallitaleaceae was significantly affected by feed supplementation. Clostridiaceae are among the phylotypes responsible for the transformation of glucose, lactate, and succinate into butyrate [49]. Furthermore, starch cleavage enzyme production is one of the main characteristics of bacteria in this family [50]. Clostridiaceae relative abundance was not only significantly higher after SSPP supplementation, but the level of significance increased with higher SSPP doses. This finding suggests that SSPP inclusion in broilers’ diets resulted in the proliferation of bacteria with amylolytic properties, indicating an overall higher metabolic activity of hydrolyzable components. The relative abundance of the genera Defluviitaleaceae, and Vallitaleaceae was also modified by SSPP supplementation, and the 0.4% SSPP group consistently showed the highest value. Interestingly, both genera were similarly affected, perhaps because they are close phylogenetic relatives with an estimated sequence similarity of about 89% [51]. In fact, in our datasets, each of these genera represented a single species which were Defluviitalea raffinosedens and Vallitalea pronyensis for Defluviitalea and Vallitalea, respectively. D. raffinosedens is a saccharolytic bacterium that acts synergistically with its cellulolytic counterpart to enhance cellulolysis by preventing feedback inhibition and improving the degradation process [52]. V. pronyensis is a fermentative anaerobic bacterium found in hydrothermal chimneys rich in alkaline compounds [53]. Interestingly, both species are thermophilic and thus can withstand temperatures ranging from 41°C to 122°C. As chicken core body temperature can reach 45°C under heat stress [54], the current results suggest that SSPP supplementation can be an appropriate approach against heat stress. Here, as described in another study [55], the relative abundance of the genus Butyricicoccus was significantly increased by dietary supplements. Furthermore, LEfSe identified B. pulliceacorum as a biomarker of SSPP supplementation (| LDA effect size | > 3). This particular species has recently been used as a probiotic in chickens [55] and humans [56] due to its resistance to bile, colonization efficiency, and butyrate production. From an intestinal health perspective, this type of butyrate-producing bacteria stimulates enterocyte growth and reduces the invasion of pathogenic bacteria such as Salmonella [57].
LEfSe also showed that R. albus, C. timonensis, A. alkalicellulosi, and A. propionicum were microbial biomarkers of dietary SSPP inclusion. In LEfSe, the effect size can be translated as the magnitude difference of the abundance [58]. Therefore, species with higher LDA scores in absolute values, rank higher for biomarker relevance. R. albus was the top-ranked biomarker (| LDA effect size | > 3.5) of SSPP supplementation. This particular specie was found to produce significant amounts of albusin-B, a bacteriocin that is lethal to several other gram-positive bacteria [59]. Since bacteria belonging to the phyla Firmicutes are gram-positive, it might therefore explain the reduction in their proportion seen after including SSPP in the diet. Information related to C. timonensis, A. alkalicellulosi, and A. propionicum remains limited and scarce, especially concerning chicken. Indeed, only studies related to the discovery and taxonomy of these microbial species are available [60,61]. Thus, more specialized experiments are needed to elucidate their potential roles in the chicken microbiome.
Interactions between bacteria and their environment contribute greatly to the balance in microbial systems [62]. In the current study, we used microbial network analysis to gain additional insights into the structure of the microbial communities. In a microbial network, cooperative interactions or similar biological functions between species are positively correlated whereas competition between species is negatively correlated [63]. From our current results, the prevalence of positive correlations observed between species belonging to the class Clostridia, was suggesting mutualistic relationships between such microbes. Similarly, the co-culture of bacteria belonging to the Clostridia class has been shown to improve the yield of cellulose fermentation [64,65]. On the other side, negative correlations in the network were found between Erysipelotrichia, Bacteroidia and Clostridia. A previous study [66] suggested that some bacteria of the classes Clostridia and Erysipelotrichia consume sugar acids and sugar alcohols to produce butyrate. Therefore, the anticorrelation between these species revealed in the network may indicate competition for nutrient availability. Consistent with our previous study [67], we detected a negative correlation between the only Bacteroidetes (Bacteroides fragilis) and a Firmicute (Acetanaerobacterium elongatum) present in our network. While there is a lack of studies reporting interactions between these two species, the negative correlation can be largely explained by considering their phylum. In fact, a significant number of trials have highlighted the negative correlation between these two phyla [15,16,68].
In conclusion, SSPP supplementation strongly influenced the cecal microbiota of broilers without affecting growth performances. When birds were fed with SSPP, there was an overall shift in microbial community structure toward a “healthier” profile. However, the results also suggest that trials focusing on supplementing SSPP for a longer period and evaluating its effect in heat-stressed birds can lead to interesting findings.