INTRODUCTION
Oxidative stress can be viewed as an imbalance between prooxidants and antioxidants in the body [1]. Various stress can adversely affect the structure and physiology of cells, causing impairment of transcription, RNA processing, translation, oxidative metabolism, membrane structure, and function [2,3]. Under normal conditions, intracellular levels of reactive oxygen species (ROS) are maintained at low levels by various antioxidant systems [4]. However, if the production of various ROS exceeds the neutralizing capacity of antioxidant systems, oxidative stress is initiated [4]. Therefore, various functional nutrients including amino acids (AA), vitamins, and minerals may be potential methods to alleviate oxidative stress in poultry.
Among various AA, dietary supplementations of threonine (Thr), tryptophan (Trp), and glycine (Gly) have gained great attention due to their effect on alleviating stress responses in the intestinal epithelium of broiler chickens [5–7]. The Thr is an important AA for mucin production [8]. The Trp is a key precursor molecule for synthesizing serotonin, melatonin, kynurenic acid, quinolinic acid, and nicotinic acid in the body [9]. Moreover, Gly works with other AA for several crucial metabolic synthesis of creatine, heme, glutathione (GSH), bile acids, nucleic acids, and uric acid [10].
Various vitamins such as vitamin C (VC), vitamin E (VE), and vitamin A (VA) are known to alleviate stress responses, which play a role as representative antioxidants in broiler chickens [11–13]. The VC has an important metabolic antioxidant role by donating an electron to oxidized molecules [14]. Likewise, Jena et al. [15] demonstrated that dietary supplementation of VE had significantly lower malondialdehyde (MDA) levels, increased activities of superoxide dismutase and catalase enzymes, and ferric-reducing antioxidant power in erythrocytes. Moreover, dietary supplementation of VA has an antioxidant effect by decreasing serum and liver MDA levels in broiler chickens reared under heat stress conditions [16].
Various minerals also play important roles in poultry. Among many minerals, chromium (Cr), selenium (Se), and Zinc (Zn), are known to improve growth performance and health by increasing antioxidant capacity of poultry. The Cr is an integral component of chromodulin, which participates in the insulin signaling process across cell membranes [17]. Insulin metabolism is known to be associated with lipid peroxidation [18,19], and therefore, Cr may also be involved in antioxidant activity in the body. In addition, Se is an integral component of glutathione peroxidase (GSH-Px) required for cellular defenses against ROS which are highly oxidizing agents in the body, especially under stress conditions [20]. Moreover, Volkova et al. [21] reported that Zn can increase the synthesis of metallothionein and copper-zinc superoxide dismutase (CuZnSOD), which acts as a free radical scavenger to lower oxidative stress in animals.
Intestinal epithelial cells (IECs) are responsible for the absorption of nutrients and could function as an efficient barrier to prevent the invasion of pathogens and toxins from the lumen [22]. The IECs originated from an animal are well-established in vitro models to study intestinal epithelial functions [23]. The use of in vitro cell culture models is highly increased because the use of in vivo animal models involves ethical issues. In addition, it is tedious and expensive to prepare and maintain in vivo animal models. However, most studies evaluating functional nutrients and oxidative stress in IECs have been performed with IECs (i.e., Caco-2 cell or IPEC-1) from humans and pigs. Thus, studies evaluating effects of functional nutrients on chickens’ intestinal epithelial cells (cIECs) exposed to oxidative stress conditions are limited.
Therefore, the objective of the present experiment was to compare the protective effects of functional nutrients on cIECs treated with oxidative stress.
MATERIALS AND METHODS
The protocol for the present experiment was approved by the Institutional Animal Care and the Use Committee (IACUC) at Chung-Ang University (IACUC approval No. A2022063).
In the present study, the cIECs were isolated and cultivated according to the method described by Kaiser et al. [22] with slight modifications [24]. Eggs from specific pathogen free (SPF) birds (VALO Biomedia GmbH, Osterholz-Scharmbeck, Germany) were purchased and incubated using an egg hatching incubator. Primary cells isolated from SPF bird’s embryo at 16 d of embryogenesis. The intestine was excised and placed in Hank’s Balanced Salt Solution (HBSS, Gibco, Pasiley, UK) supplemented with Calcium and Magnesium. The intestine was washed with HBSS to remove blood and impurities. It was cut into small fragments (~1–2 cm) at room temperature and placed into a 50-mL tube containing HBSS medium. Intestinal tissue fragments were cut again into small pieces with a sterile scalpel blade and placed into a 50-mL tube with 25 mL of digestion medium: Dulbecco Modified Eagle Medium (DMEM; Hyclone, Logan, UT), 2.5% fetal bovine serum (FBS; Corning Cellgro, Manassas, VA, USA), 2,500 μg/mL gentamicin (Sigma-Aldrich, St. Louis, MO, USA), 100 μg/mL penicillin/streptomycin (Gibco, Grand Island, NY, USA), 1 U/mL dispase II (Sigma-Aldrich), and 75 U/mL collagenases (Sigma-Aldrich). Intestinal fragments were digested in a digestion medium at 37°C for 2 h. Digested intestinal fragments were then filtered through cell strainers (width 40 μm and 100 μm; Corning, Falcon, Vineland, NJ, USA) to isolate crypts from larger intestinal cells. After the crypt was isolated, the acquired material was centrifuged at 100×g for 3 min. The supernatant was removed, and the residual cell pellet was replaced in washing medium (DMEM, 2% D-sorbitol, 2.5% FBS, 100 μg/mL penicillin/streptomycin and 2,500 μg/mL gentamicin) and centrifuged at 400×g and 10 min to isolate cIECs. These cIECs were mixed with 10 mL of growth medium (DMEM, 2.5% FBS, 10 μg/mL insulin, 100 μg/mL penicillin/streptomycin, 2,500 μg/mL gentamicin and 1,400 μg/mL hydrocortisone). After, cIECs were seeded in cell culture plates (SPL, Pocheon, Korea) coated with fibronectin (Sigma-Aldrich) and incubated at 37°C with 5% CO2. Cells were routinely cultivated in the culture medium (DMEM, 2.5% FBS, 10 μg/mL insulin, and 100 μg/mL penicillin/streptomycin).
Each dose of functional supplements was mixed with culture medium (DMEM, 2.5% FBS, 10 μg/mL insulin and 100 μg/mL penicillin/streptomycin). Before the formal experiments, preliminary tests were done to determine optimal concentrations of supplements on cIECs by measuring cell viability. Each supplemental level of functional nutrients was reported by previous studies. Cells were then exposed to 0 mM supplement (control), 20 mM Thr (L-Thr; Sigma-Aldrich) [25], 0.4 mM Trp (L-Trp; Sigma-Aldrich) [26], 1 mM Gly (Sigma-Aldrich) [5], 10 μM VC (L-ascorbic acid; Sigma-Aldrich) [27], 40 μM VE (α-tocopherol acetate; Sigma-Aldrich) [28], 5 μM VA (retinyl acetate-water Soluble; Sigma-Aldrich) [29], 34 μM Cr (potassium dichromate; Sigma-Aldrich) [30], 0.42 μM Se (seleno-L-methionine; Sigma-Aldrich) [23], and 50 μM Zn (zinc sulfate heptahydrate; Sigma-Aldrich) [31] for 24 h with 6 replicates for each treatment. After 24 h, cells were further incubated with the fresh culture medium (positive control, PC) or 1 mM H2O2 (negative control, NC; added from stock solutions prepared in cell culture medium) with different supplements (each treatment).
Cells were seeded into 96-well plates at a density of 5 × 104 cells/well and incubated at 37°C for 24 h in a humified atmosphere containing 5% CO2. The experimental procedure followed the method of Chung et al. [32]. Briefly, cells were incubated for 24 h and treated with or without 1 mM H2O2 for 6 h. After exposure to functional supplements and H2O2, cell viability was determined using a Quanti-MAX WST-8 assay (Biomax, Seoul, Republic of Korea) following the manufacturer’s protocol. After incubation, cells were replaced with 100 μL of fresh culture medium. After adding 10 μL of WST-8 assay solution into each well, cells were then incubated for 4 h. Subsequently, the absorbance of cells in each well was measured at 450 nm using a microplate reader (Spectramax 190, Molecular Device, CA, USA).
Tight junction permeability in cIECs was measured using fluorescein isothiocyanate (FITC)-dextran as demonstrated by Park et al. [33] and Hoff et al. [34]. Briefly, cells were seeded in the apical chamber of a 24-well transwell filter with 0.4 μm pores (SPL) at 4 × 105/well and incubated at 37°C for 24 h in a humified atmosphere containing 5% CO2. For cell differentiation, the culture medium was replaced with a differentiation medium containing 50 μg/mL dexamethasone (Sigma-Aldrich) every 2 d. On the 9th day of the seeding process, cells were incubated with supplements for 24 h and treated with or without 1 mM H2O2 for 6 h. After cells were treated, FITC-dextran (Sigma-Aldrich) dissolved in cell differentiation medium was added to the apical chamber at a final concentration of 2.2 mg/mL and well plates were incubated for 1 h. Five aliquots of 200 μL were taken from each well of a 24-well plate and added into a black 96-well plate with a clear bottom (SPL). The amount of fluorescence in the black 96-well plate was measured by a fluorescence reader (Spectramax, Gemini EM, Molecular Devices, Sunnyvale, CA, USA), at excitation and emission wavelength of 490 and 535 nm, respectively.
The trans-epithelial electrical resistance measurements were conducted as described by Du et al. [35] and Vergauwen et al. [27]. Briefly, differentiated cIECs in 0.4 μm pores apical chamber as described above were treated with functional supplements and H2O2. Then 24-well plates were placed in a hot plate (Daihan scientific, Wonju, Republic of Korea) at 37°C. The TEER was measured at 0 h, 24 h, and 48 h using an EVOM 3 epithelial voltohmmeter (World Precision Instruments, Sarasota, FL, USA) following the manufacturer’s protocol. The TEER was calculated as Ω·cm2 by multiplying by the surface area of the apical chamber (0.33 cm2).
Statistical analysis was performed as a completely randomized design by using the PROC MIXED procedure of SAS (SAS Institute, Cary, NC, USA). Each replicate was considered an experimental unit. Outlier data were checked by the UNIVARIATE procedure of SAS [36]. The LSMEANS procedure was used to calculate treatment means. In addition, if the difference was significant, the PDIFF option of SAS was conducted to separate the means. The significance for statistical tests was considered at p < 0.05.
RESULTS
The effects of various functional feed nutrients on the viability of cells treated with oxidative stress of H2O2 are shown in Fig. 1. The cell viability was less (p < 0.05) in NC treatments with oxidative stress and no supplements than in PC treatments without oxidative stress and supplements. All treatments of functional nutrients in cells treated with H2O2 increased (p < 0.05) cell viability as compared to NC treatments. The supplementations of Thr, Trp, Gly VC, and VE in cells treated with H2O2 showed greater (p < 0.05) cell viability than the supplementation of other nutrients including VA, Cr, Se, and Zn.
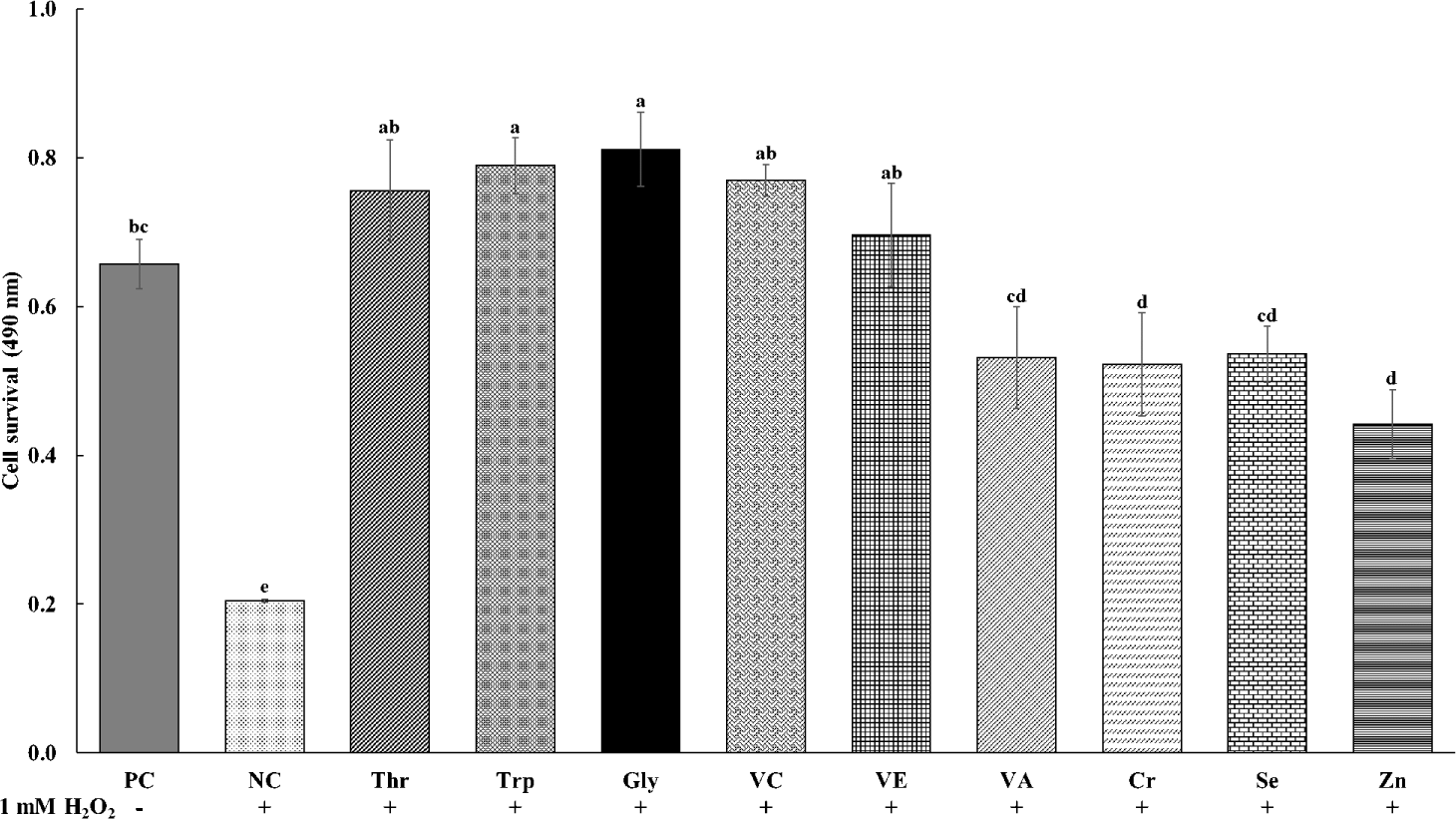
The effects of various functional nutrients on cellular permeability of tight junctions in cIECs treated with oxidative stress of H2O2 are presented in Fig. 2. The cell permeability was the greatest (p < 0.05) in NC treatments with oxidative stress and no supplements among all treatments. All treatments of functional nutrients decreased the cellular permeability in cells treated with H2O2. The supplementations of Trp, Gly, VC, and Se in cells treated with H2O2 showed the least (p < 0.05) cellular permeability. In addition, the supplementation of Thr, VE, VA, Cr, and Zn in cells treated with H2O2 decreased (p < 0.05) cellular permeability and showed similar values with PC treatments without oxidative stress and supplements.
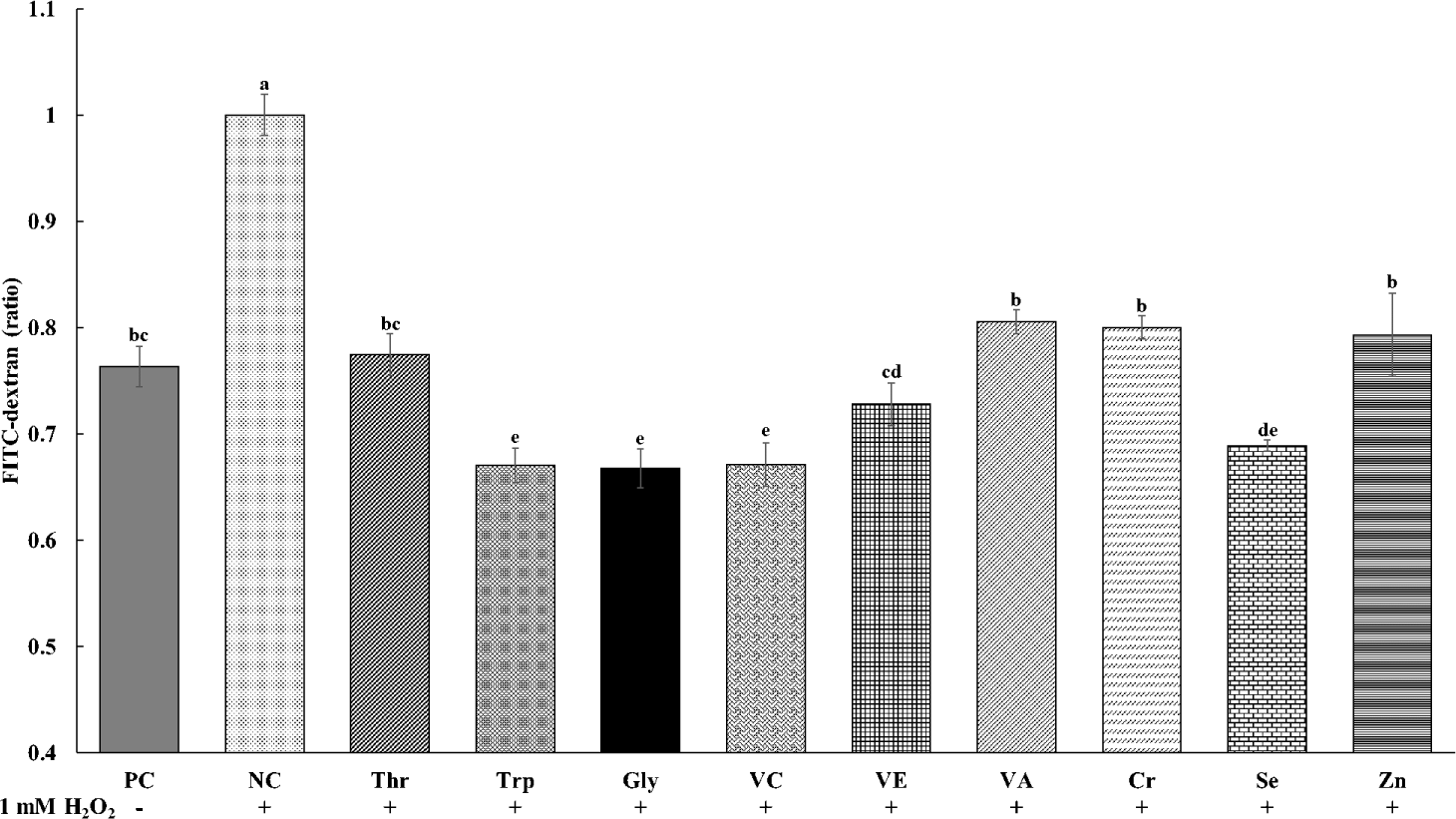
The effects of various functional nutrients on the TEER values for cIECs are shown in Fig. 3. At all measurement times, the TEER values were the least (p < 0.05) consistently in NC treatments with oxidative stress and no supplements among all treatments. All treatments of functional nutrients increased (p < 0.05) TEER values in cells treated with H2O2. At 24 h, the supplementations of Trp and Gly in cells treated with H2O2 showed greater (p < 0.05) TEER values than PC treatments without oxidative stress and supplements. Furthermore, other supplementations of Thr, VC, VE, VA, Cr, Se, and Zn in cells treated with H2O2 showed no difference with PC treatments. At 48 h, the supplementations of Thr, Trp, and Gly in cells treated with H2O2 showed the greatest (p < 0.05) TEER values among all treatments, and the supplementations of VC and VE in cells treated with H2O2 showed greater (p < 0.05) TEER values than the supplementations of VA, Cr, Se, and Zn in cells treated with H2O2.
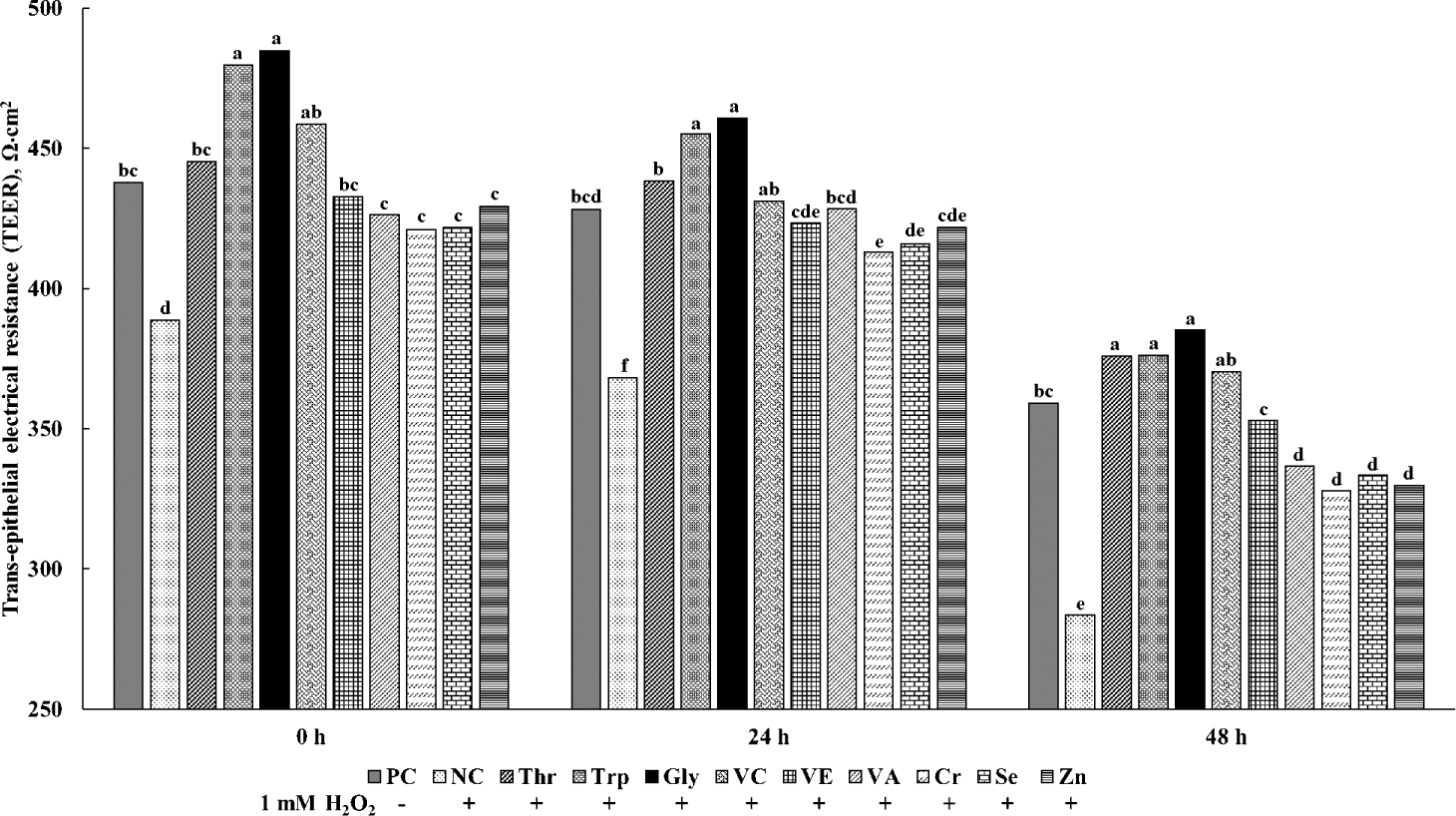
DISCUSSION
In the present study, H2O2 was used as a potent trigger of oxidative stress to disrupt the intestinal epithelial barrier of cIECs [37]. Oxidative stress can be recognized as an imbalance between prooxidants and antioxidants in the body [38]. Under stress conditions, antioxidant systems based on several antioxidant agents and mechanisms can protect cells in the body from the harmful effects of oxidative stress [39,40]. Oxidative stress can damage cell membrane structure and disrupt tight junction complex in the intestine [41]. For this reason, increases of macromolecules, endotoxins, and pathogens due to impaired tight junctions are also related to decreased absorption of nutrition and increased inflammation in the intestine [42]. Exposure to H2O2 resulted in numerous dead or disrupted cells [43]. In addition, exposure to 1 mM H2O2 for 1 h resulted in acute cytotoxicity to IPEC-J2 cells [27]. Similar observation was found in the current experiment.
The results from our experiment also revealed that all functional supplements increased the cell viability of cIECs treated with H2O2 compared with NC treatments, consistent with previous studies reporting that various functional supplements could improve cell viability of cells treated with H2O2 by each mechanism. Baird et al. [25] reported that Thr enhanced protective heat shock protein (HSP) 70 expression and cell size stabilization by cellular osmosensing pathway for protecting cells in IPEC-1 from oxidative stress caused by heat stress. Wang et al. [26] reported that 0.4 and 0.8 mmol/L Trp stimulated cell growth because of the fact that Trp activates rapamycin complex 1 (mTORC1) known to regulate cell growth, protein synthesis, and proteolysis in various types of cells. Wang et al. [5] reported that increasing supplementation of Gly increased the growth of IPEC-1 because Gly is known as an essential precursor for the nucleotides that stimulated protein synthesis and inhibited proteolysis in IPEC-1. The VC is well-known as an antioxidant by donating an electron to oxidized molecules [14]. Vergauwen et al. [27] reported that the supplementation of VC increased cell viability and decreased injury in IPEC-J2 under oxidative stress. The VE and VA are also known as antioxidants that prevent lipid peroxidation in the cell membrane by preventing its chain propagation via the removal of free radicals [11,44]. In addition, Cr is an integral component of chromodulin known to participate in the insulin actions that are associated with lipid peroxidation [19,45]. Likewise, Se is an integral component of GSH-Px that is required for cellular defenses against ROS [20]. Volkova et al. [21] reported that Zn increases the synthesis of CuZnSOD, which acts as a free radical scavenger to lower oxidative stress in animals. As described above, nine supplements used in the current study are recognized as representative functional nutrients that act as antioxidants to protect cells from stress or precursors of major molecules in the body. Therefore, our current result agreed with previous studies indicating that functional nutrients increased cell viability under oxidative stress because of increase in cell growth, protein synthesis, and antioxidant capacity.
Tight junctions of intestinal epithelium are mainly composed of transmembrane protein complexes such as occludin (OCLN), claudin (CLDN), and zonula occluding-1 (ZO-1) as cytosolic proteins [46]. Furthermore, the mucin 2 (MUC2) gene expressed by goblet cells is associated with mucin production in the mucous layer of the intestinal epithelium, which also plays a role in intestinal barrier functions [47]. The improvement of tight junction proteins is expected to enhance intestinal mucosal barrier function by sealing the paracellular space between neighboring epithelial cells, therefore protecting the intestine against the passage of toxzins or microorganisms into the body [5]. In addition, tight junction proteins are related to restriction of movement of lipids and membrane-bound proteins between apical and basolateral membranes, thereby contributing to the cell polarity for appropriate intestinal mucosal function [48].
Oxidative stress can damage cell membrane structure and disrupt tight junction complexes in the intestine [41]. Increasing intestinal permeability under stress conditions disrupted intestinal epithelial integrity [49]. In addition, the disruption mechanism of IECs under various stress conditions is associated with tight junctions and adherens junctions [50]. Disruption of tight junctions enables free passage of macromolecules, endotoxins, or pathogens. It is also related to decreased absorption of nutrition and increased inflammation in the intestine [42,51]. The intestinal cells under stress conditions show decreased TEER, which is commonly used to measure intestinal permeability with increasing TEER values indicating decreased intestinal permeability [52].
The results from the current study showed that functional nutrients decreased the FITC-permeability and increased TEER values in cIECs under oxidative stress conditions. Permeability assay by FITC-dextran and TEER measurement were applied to measure the functional integrity of the epithelial monolayer [53,54]. This study clearly showed the positive effects of functional nutrients on membrane integrity as assessed by FITC-dextran flux and TEER. As expected, functional nutrients used in this study increased epithelial monolayer integrity in the IECs under oxidative stress. Azzam et al. [55] reported that increasing Thr levels can improve MUC2 gene expression of the ileum and jejunum mucosa in laying hens under heat stress conditions. The MUC2 gene is the marker of goblet cells as a direct target of transcription factors involved in intestinal development and cell differentiation [56]. Wang et al. [26] reported that supplementation of Trp upregulated the abundance of OCLN, CLDN, and ZO-1 in IECs. The Gly can indirectly alter mucin turnover, either by lowering the catabolism of Thr into Gly or serving as a substrate for the synthesis of mucin, given that Gly is placed in the central structure of mucin [57]. Vergauwen et al. [27] reported that VC significantly increased TEER value and decreased FITC-dextran permeability in IPEC-J2 cell monolayer under oxidative stress conditions. Liu et al. [43] showed that dietary supplementation of VE and Se increased TEER values and decreased FITC-dextran permeability in the intestine of pigs exposed to heat stress. Likewise, dietary supplementation of VE and Se improved the epithelial conductance in rats under heat stress conditions [58]. Xiao et al. [29] described that VA increased TEER values and expression levels of OCLN, CLDN, and ZO-1 in Caco-2 cells treated with LPS. Huang et al. [59] reported that dietary supplementations of Cr increased gut morphology such as villus height and villus height:crypt depth ratio in broiler chickens under heat stress conditions. Pardo and Seiquer [60] reported that supplementation of Zn in Caco-2 cells under heat stress conditions decreased intestinal permeability and increased TEER values. Therefore, our results of intestinal permeability agreed with previous studies indicating that functional nutrients used in this study increased intestinal integrity under stress conditions.
In the current study, Thr, Trp, Gly, and VC were more effective than other nutrients in increasing cell viability and decreasing cell permeability. However, there is a lack of data regarding comparison of cellular effects in cIECs treated with various nutrients under oxidative stress conditions. In stress conditions, AA requirement of chickens is reported to be increased [6,61,62]. Therefore, adequate intake of Thr and its efficient utilization by the intestinal cells are required to maintain mucosa integrity [8]. In addition, Thr in enterocytes can be metabolized via the catabolic pathway such as Gly, acetyl CoA, and pyruvate metabolism [63,64]. In addition, Baird et al. [25] showed that Thr prevents cellular apoptosis and enhances cellular HSP expression in an in vitro model of cells exposed to heat stress. The Trp play a role in protein synthesis as other AA, but it is also a key precursor molecule for synthesizing serotonin, melatonin, kynurenic acid, quinolinic acid, and nicotinic acid in the body [9]. Likewise, Gly contributes to various metabolic functions and works with other AA for several crucial metabolic syntheses of creatine, heme, GSH, bile acids, nucleic acids, and uric acid [10]. The VC has an important metabolic role because of its reducing properties by donating an electron to oxidized molecules [14]. Although other nutrients also have various antioxidant effects in cells treated with H2O2, Thr, Trp, Gly, and VC had more positive effect on improving the antioxidant capacity of cells in the current experiment. To the best of our knowledge, this is the first study to explore the effects of nine functional nutrients on tight junction barrier functions of cIECs. However, the clear reason why Thr, Trp, Gly, and VC were more effective than other nutrients is not available. Further studies are required to identify different cellular mechanisms regarding cell functions and integrity among functional nutrients.
In conclusion, all functional nutrients used in the current study improve cell viability and decrease intestinal permeability. Especially, Thr, Trp, Gly, and VC are more effective in improving cell viability and decreasing intestinal permeability of cIECs. The current experiment can provide the potential approach to screen various functional nutrients in vitro before conducting in vivo studies.