INTRODUCTION
Postweaning multisystemic wasting syndrome (PMWS) is now referred to as a porcine circovirus type 2 (PCV2) systemic disease (PCV2-SD) because PCV2 infection is evidently associated with PMWS [1,2]. PMWS was first found in the middle of 1990’s. It was one of the most disastrous diseases in pig industry worldwide before PCV2 vaccine was first commercialized in 2006 [1-3]. After the introduction of a vaccine against PCV2a type, pig producers gradually recovered from economic losses caused by PMWS [4,5]. However, several evidence indicates that vaccination against PCV2a type cannot completely remove circulating PCV2 after an infection [6,7]. Besides, PMWS still occurs occasionally in vaccinated piglets [8,9]. Several speculations have been raised to explain why PCV2a vaccine is not effective to eradicate PCV2 infection. First, PCV2a vaccine might not cover all genetic variations of PCV2. At least eight different PCV2 genotypes (PCV2a – PCV2h) have been identified based on their capsid structures [10]. Indeed, the field studies in US and Korea have reported the failure of PCV2a vaccine during outbreaks of PCV2b mutant infections [8,9]. Second, vaccination against PCV2 itself can be a driven force to induce antigenic variation of PCV2 capsid [11,12]. Therefore, developing multivalent vaccines against PCV2 is currently ongoing [13]. Overall, PCV2 infection is still considered as one of the most critical problems in pig industry worldwide [14].
Clinical symptoms after PCV2 infection can vary from regional inflammation targeting reproductive, respiratory, or digestive tracks to systemic inflammation including PMWS [15]. Although the mechanism is not clear, systemic infection of PCV2 in piglets often causes an immune suppression by depleting peripheral lymphocytes [16]. Therefore, PMWS caused by systemic PCV2 infection can gradually decrease the immunity of piglets and cause a secondary infection by bacteria or other viruses [17]. Defining which open reading frame (ORF) of PCV2 modifies a host cell physiology by interacting with host cell proteins is very helpful for understanding the molecular pathology of PCV2. This is particularly significant when we exam the pathology of PMWS related to immune suppression, which is a well-known strategy used by PCV2 to escape from host immune surveillance [18]. In this review, we will summarize current knowledge of interactions between each ORF of PCV2 and host proteins and introduce several theories on how PCV2 causes immune suppression after infection.
GENOTYPES AND ASSOCIATED DISEASES OF PORCINE CIRCOVIRUSES
PCV belongs to the genus Circovirus in the family Circoviridae which has a non-enveloped icosahedral capsid [17]. The genome of PCV is composed of a single-stranded DNA with a closed circular shape (Fig. 1). It contains very small amounts of genetic information with a length of 1.7 to 2.0 kb [19]. Four PCV genotypes have been identified so far [20]. Recent phylogenetic analysis based on amino acid sequences of replicase (ORF1) of each PCV genotype has indicated that PCV1, PCV2, and PCV3 might be derived from bat circovirus because PCV1 and PCV2 share a common ancestor with bat-clade 2 circoviruses and replicase of PCV3 is most similar to bat-clade 1 circoviruses [21,22]. However, PCV4 shares a common ancestor with mink circoviruses having a distinct origin from PCV1 - PCV3 [22]. Therefore, pig circovirus might have emerged from cross-species transmission of other mammalian species [21].
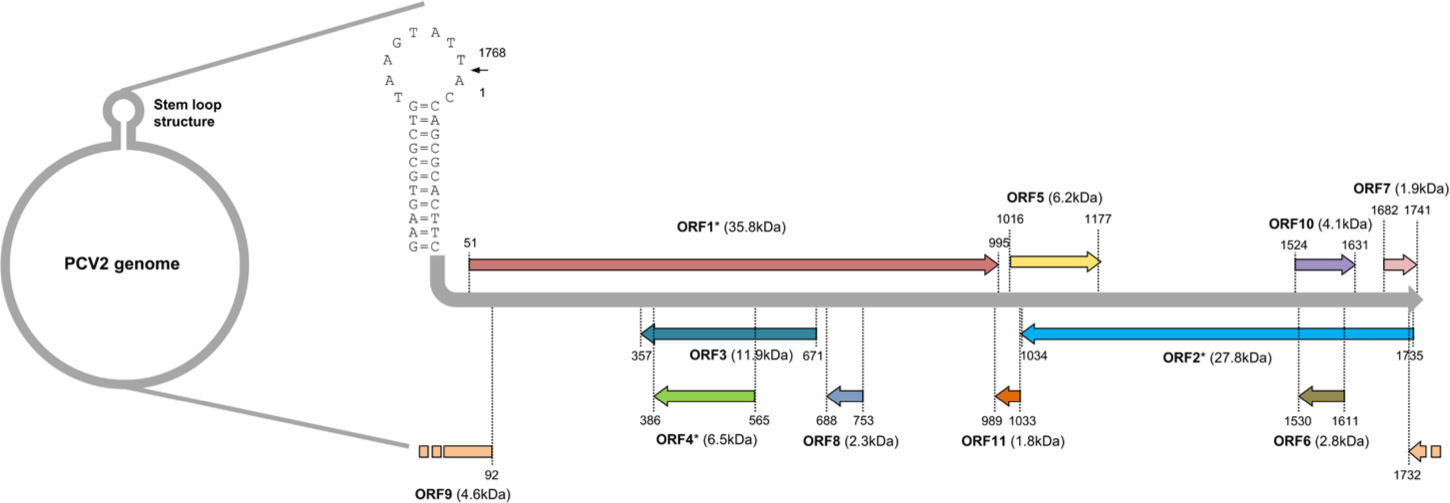
PCV1 was originally identified in the genome of immortalized porcine kidney cells (PK-15 cells) [19]. PCV1 infection does not cause any types of swine diseases [19]. In contrast to PCV1, PCV2 infection causes porcine circovirus-associated diseases (PCVAD) including porcine dermatitis and nephropathy syndrome (PDNS), PMWS, and porcine reproductive diseases [23-25]. PCV3 genome was first identified in 2015 [26]. PCV3 infection is also associated with various swine diseases including gastrointestinal, neurological, reproductive, and respiratory disorders [26-28]. PCV4 was first identified by serotyping as early as 2008, but the genome of PCV4 was fully characterized in 2019 [29]. Recent observations have indicated that PCV4 infection is also correlated with clinical symptoms of pigs such as gastrointestinal and respiratory distresses, and PDNS [22,30,31]. Therefore, all identified types of PCV except PCV1 are considered as major pathogens to threat pig industry worldwide.
PROPOSED FUNCTION OF EACH PORCINE CIRCOVIRUS TYPE2 OPEN READING FRAME
Eleven ORFs have been predicted to encode PCV2 genome [32]. Among them, ORF 1, 5, 7, and 10 are likely to be located on the sense strand of PCV genome and the rest of ORFs are encoded by the anti-sense strand of PCV genome (Fig. 1) [32]. Until now, only six ORFs from ORF1 to ORF6 have been confirmed to produce polypeptides with their putative functions characterized [33,34]. The ORF1 encodes enzymes essential for the replication of PCV2 [35]. Several mRNA splicing variants have been detected from ORF1. These splicing variants are also involved in the replication of PCV2 [35]. The ORF2 encodes capsid, a major structural protein and the major source of PCV2 vaccine. It serves as a target of neutralizing antibodies to block viral entry to host cells [36]. The rest of identified ORFs (ORF3 – ORF6) are not essential for the replication of PCV2. They mainly modulate host cell physiology by interacting with host cell proteins [34,37-39].
The pathogenesis of PCV2 infection is not fully understood yet. Although the infection route of PCV2 is currently unclear, detectable amounts of PCV2 have been found in secretions and excretions of infected pigs [40-43]. Therefore, oral, nasal, and fecal contaminations might mediate horizontal transmission [44-46]. Vertical transmission could also occur through maternal-fetal interface [47,48]. One of the symptoms of PCVAD is abortion or mummification of fetus because infected fetus cannot survive due to PCV2 replication in heart, liver, lung, or lymphoid tissues of fetus [48-51].
PCV2 mainly infects hematopoietic lineage cells such as lymphocytes and macrophages [52,53]. Especially, massively proliferating lymphoblasts are known to be major host cells of PCV2 [53]. Since these major host cells of PCV2 are immune cells, PCV2 particles are preferentially found in lymphoid tissues [24]. However, several reports have provided evidence that PCV2 replication occurs in non-lymphoid tissues such as lungs, livers, and kidneys of piglets [54,55]. This may indicate that macrophages in non-lymphoid tissues can be infected when PCV2 infection is systemically disseminated in the pig body, or PCV2 itself can infect other cell types besides immune cells. Other cell types reported to be host cells of PCV2 include endothelial cells, epithelial cells, fetal cardiomyocytes, and primary hepatocytes [56-61]. However, these results are mainly acquired from in vitro infection studies using several cell lines or primary cells [56-61].
To study the life cycle of PCV2 or the interaction between PCV2 ORFs and host proteins, two types of pig cell lines, 3D4/31 and PK-15, have been extensively used. 3D4/31 cells are derived from pig alveolar macrophages and PK-15 cells are pig kidney epithelial cells [62,63]. Genetically engineered artificial PCV2 or natural PCV2 originally isolated from pigs with PMWS can infect and replicate within both cell lines [64,65].
Previous study using 3D4/31 or PK-15 cells has shown that the capsid of PCV2 can recognize heparan sulphate or chondroitin sulphate B glycosaminoglycans on host cells [66]. PCV2 can attach to these receptors expressed on porcine macrophages within 30 minutes [65]. A motif containing “98IRKVKV103” residues within PCV2 capsid has been identified as a putative heparin binding motif based on structural analysis using crystallography [67]. After attachment, PCV2 particle is internalized via clathrin-dependent endocytosis. Nucleic acid of PCV2 is then released by endosomal acidification [65]. These processes are further confirmed by treatment with a serine protease inhibitor which can abolish penetration of PCV2 genome into cytoplasm of both 3D4/31 and PK-15 cells [68]. After penetration, single-stranded DNA of PCV2 is transported into nucleus, converted to double-stranded intermediates, and replicated via a rolling-circle replication method [69].
The hallmark of PMWS mediated by systemic PCV2 infection is immunosuppression in pigs [14,24]. Major leukocytes including helper T cells, B cells and dendritic cells are significantly decreased in pigs with PMWS [70-72]. These observations have been further supported by the fact that PCV2 titers in periphery of pigs are correlated with the severity of immune cell destruction and antibody deficiency against PCV2 [72]. Immunosuppression due to depletion of immune effector cells can cause secondary infections in pigs with PMWS [16,73-75]. Therefore, pigs with PMWS, similar to immunocompromised people, are not directly killed by PCV2 infection, but sacrificed by secondary infections with other microbial pathogens targeting lung or digestive tracks [76,77].
HOST CELL MODULATION BY PORCINE CIRCOVIRUS TYPE2 OPEN READING FRAMES
Virus infection can significantly change physiological conditions of infected animals. Since host inflammatory responses are dependent on the titer and antigenicity of viral antigens and/or the ability of infiltrated immune cells in certain infectious sites, the clue explaining how virus can modulate host physiology might be found in specific interactions between viral proteins and host proteins. There are many examples indicating the virus itself has evolved to have several strategies to downregulate or escape immune surveillance of its host. Otherwise, viruses cannot survive inside their hosts. Several evidence has shown that PCV2 can also use this tactic inside its host. Each ORF’s function related to modulation of host cell physiology or immune surveillance by having a crosstalk with host proteins will be discussed below.
PORCINE CIRCOVIRUS TYPE2 OPEN READING FRAME1
Since PCV2 ORF1 encodes replicase of PCV2, its main role is focus on generation of progeny virus rather than interaction with host cell proteins [35]. Only two studies have identified PCV2 ORF1 interacting proteins from host cells by screening bacterial or yeast two hybrid libraries using PCV2 ORF1 as a bait [78,79]. Five PCV2 ORF1 binding partners have been identified in host cells (Table 1). Two of them, syncoilin and c-myc, have been identified in bacterial two hybrid libraries and the rest of them, thymine DNA glycosylase (TDG), angiogenic factor with G patch and FHA domains 1 (VG5Q), and zinc finger protein 265 (ZNF265), have been identified in yeast two hybrid libraries [78,79]. Especially, TDG, VG5Q, and ZNF265 can bind to both PCV1 and PCV2 replicase [79].
PCV2 ORF | Host cell protein | Function of host cell protein | Biological meaning between specific ORF and host cell protein | Reference |
---|---|---|---|---|
ORF1 (Rep) | Syncoilin | Intermediate filament protein involved in transport processes | Transports viral proteins to subcellular organelles in host cells | [78] |
c-myc | Transcription factor which promotes cell cycle progress and DNA replication | Initiates viral DNA replication | [78] | |
TDG | Thymidine DNA glycosylase associated in G/T mismatch repair | Participates in viral DNA replication | [79] | |
VG5Q | Angiogenic factor which regulates vascular development | Unknown | [79] | |
ZNF265 | Subunit of pre-mRNA spliceosome required for alternative splicing | Affects transcription and viral mRNA splicing | [79] | |
ORF2 (Cap) | P-selectin | Cell adhesion molecule involved in an interaction between endothelial cells and leukocytes | Facilitates viral attachment and entry into host cells | [78] |
C1qBP | Complement factor which binds with the globular heads of C1q | Mediates phagocytosis and production of proinflammatory cytokines | [78,79,103,104] | |
Hsp40 | Chaperone which delivers unfolded and misfolded proteins to Hsp70 | Assists Hsp70 to enhance progeny virus production | [79] | |
Hsp70 | Chaperone involved in protein folding | Increases progeny virus production by elevating PCV2 genome replication | [107] | |
MKRN1 | RNA-binding E3 ubiquitin ligase which modulates cell cycle or senescence | Unknown | [79] | |
NAP1 | Histone chaperone which participates in nucleosome assembly | Unknown | [79] | |
NPM1 | Nucleolar protein shuttling between nucleus and cytoplasm | Unknown | [79,116] | |
Par-4 | Caspase-3 substrate | Promotes apoptosis in host cells | [79] | |
DDX21 | RNA helicase involved in ribosomal RNA processing | Unknown | [116] | |
EIF4A2 | RNA helicase which is a component of eIF4F complex | Unknown | [116] | |
hnRNPC | RNA-binding protein which regulates RNA homeostasis | Unknown | [116] | |
IC1 | Protease inhibitor which has anti-inflammatory effects | Participates in local inflammation | [116] | |
IPO5 | Nuclear transport receptor which belongs to importin beta family | Unknown | [116] | |
MX2 | IFN-induced GTPase interfering with viral capsid transport | Blocks the progeny virus production | [116] | |
SNAI2 | Transcription factor upregulating cell differentiation and migration | Unknown | [116] | |
ORF3 | Pirh2 | E3 ubiquitin ligase involved in proteasomal degradation of p53 | Causes apoptosis by increasing half-life of p53 | [127,128] |
DDE-like transposase | Transposase catalyzing the movement of DNA segments | Unknown | [78] | |
RGS16 | G protein signaling inhibitor which suppresses NFκB translocation | Promotes inflammatory responses by degradation of RGS16 | [78,132,133] | |
ORF4 | FHC | Ferroxidase which removes reactive oxygen intermediates | Rescues host cells from apoptosis | [137] |
ANT3 | Translocase that mediates ADP/ATP exchange in mitochondrial matrix and cytosol | Leads to apoptosis by mitochondrial damage | [139] | |
COX8A | Component of COX involved in the hydrogen transfer in mitochondria | Unknown | [137] | |
SNRPN | Polypeptide associated with pre-mRNA splicing | Unknown | [137] | |
Lamin C | Type V intermediate filament protein which is a major component of the nuclear lamina | Unknown | [137] | |
ORF5 | CYP1A1 | Member of the cytochrome P450 which functions as monooxygenases | Unknown | [144] |
GPNMB | Type l transmembrane glycoprotein associated with cell proliferation and anti-inflammatory responses | Inhibits viral replication | [144,150] | |
SRSF3 | Pre-mRNA splicing factor which promotes exon-inclusion during alternative splicing | Unknown | [144] | |
YWHAB | Adaptor protein which facilitates cell proliferation | Prevents PCV2-induced autophagy and apoptosis | [144,151] | |
ZNF511 | Transcription factor interacting with RNA polymerase ll | Unknown | [144] |
Syncoilin is an intermediate filament protein found in various cell types including skeletal and cardiac muscle, hepatic stellate cells, and neurons [80-82]. It is valuable to test whether blocking syncoilin’s function can inhibit PCV2 replication because vimentin, another intermediate filament protein, is the target for a novel drug to block viral protein transport to specific organelle in host cells [83-85]. As a well-known transcriptional factor and an oncogene, c-myc can facilitate cell cycle progress [86]. It can also initiate DNA replication non-transcriptionally by binding with members of the prereplication protein complex [87,88]. Therefore, PCV2 replicase could bind with c-myc for the initiation of DNA replication in host cells.
TDG is involved in G/T mismatch repairs by removing thymine residues from sugar-phosphate DNA backbone [89]. VG5Q is highly expressed in activated endothelial cells. It can promote angiogenesis by interacting with proteins involved in vascular development [90]. Overexpression of VG5Q can lead to poor prognosis by increasing cancer metastasis [91,92]. ZNF265 is a zinc finger protein that contains an arginine/serine-rich domain and a subunit of pre-mRNA spliceosome [93]. Therefore, ZNF265 is involved in mRNA splicing [93]. Since TDG and ZNF265 participate in DNA replication and mRNA splicing, PCV2 replicase may interact with these proteins during replication or splicing of viral DNA or mRNA. However, the reason why PCV2 replicase binds with VG5Q, an angiogenetic factor, is still elusive.
PORCINE CIRCOVIRUS TYPE2 OPEN READING FRAME2
Initial binding partners of PCV2 capsid, an ORF2 encoding protein, have been screened using bacterial two hybrid libraries and PK-15 cDNA [78]. P-selectin and complement component 1, q subcomponent binding protein (C1QBP or gC1qR) have been found to be able to bind to capsid (Table 1) [78]. P-selectin is one of the selectins that belong to C-type lectin, a carbohydrate binding protein [94]. P-selectin is mainly expressed on activated endothelial cells and platelets [95]. Although additional example showing that viral capsid can bind to P-selectin has not been documented yet, L-selectin, its closest paralog, is critical for human immunodeficiency virus (HIV)-entry into host cells by interacting with gp120, an HIV spike protein [96]. Therefore, PCV2 capsid might interact with P-selectin during attachment to host cells.
C1QBP is a receptor of globular heads of C1q which is expressed on a broad range of cell types [97]. C1QBP is mainly expressed in the mitochondrial matrix. It is also detected in nucleus, endoplasmic reticulum (ER), and cell surface [98]. Therefore, C1QBP could play multifunctional roles in various cellular events based on its multi-subcellular distributions [98]. Indeed, C1QBP can mediate various inflammatory responses including blood clotting, recruitment of leukocytes, phagocytosis, and production of reactive oxygen intermediates [99-102]. Another study has also identified C1QBP as a binding protein of PCV2 capsid protein through screening using a yeast two-hybrid system [79]. Further study has revealed that PCV2 capsid can facilitate phagocytic activity and secretions of proinflammatory cytokine in porcine macrophages by inhibiting ubiquitin-mediated proteasomal degradation of C1QBP via direct interactions [103]. This finding is further supported by additional reports demonstrating that the interaction between C1QBP and PCV2 capsid can induce IL-10 production by activating PI3K/Akt and p38 MAPK pathways in porcine alveolar macrophages [104]. Additional host proteins identified as binding partners of PCV2 capsid by screening a yeast two-hybrid library include heat shock protein 40 (Hsp40), makorin-1 RING zinc finger protein (MKRN1), nucleosome assembly protein-1 (NAP1), nucleophosmin-1 (NPM1), and prostate apoptosis response-4 (Par-4) (Table 1) [79]. Hsp40 is a molecular chaperone that can assist the function of Hsp70 with nucleotide-exchange factor (NEF) [105,106]. Hsp40 can bind to misfolded proteins and deliver these proteins to Hsp70, and NEF can catalyze the dissociation of adenosine diphosphate (ADP) from Hsp70 to allow ATP binding to Hsp70 [106]. Therefore, Hsp40 and NEF are essential for the function of Hsp70, the common molecular chaperone for protein folding in mammalian cells [106]. In agreement with this observation, an independent study has demonstrated that PCV2 capsid can interact with Hsp70 to enhances progeny virus production of PCV2 by increasing PCV2 genome replication in 3D4/31 cells [107].
Among host proteins that can bind to PCV2 capsid, MKRN1, NAP1, and NPM1 are nuclear proteins or proteins with nucleotide binding motifs [108-114]. MKRN1 is an RNA-binding E3 ubiquitin ligase with an RNA binding property and conserved zinc finger domains [108]. MKRN1 also has an E3 ubiquitin ligase activity that can regulate cell cycle or cell senescence by regulating target protein turnover rates [108-110]. NAP1 is an evolutionally conserved protein. It might be involved in a nucleosome assembly because it has been found in several different chromatin remodeling complexes [111,112]. NPM1 is often found in nucleolar ribonucleoprotein structures. It serves as an abundant nucleus-cytoplasmic shuttling protein to transport nucleic acid [113,114].
Par-4 serves as a caspase-3 substrate that can enhance apoptosis under various apoptotic signals [115]. Since PCV2 infection can lead to lymphocyte depletion by inducing host cell apoptosis, how the interaction between PCV2 capsid and Par-4 regulates apoptosis in host cells might be a necessary research subject [70-72].
A recent study using co-immunoprecipitation with anti-PCV2 capsid antibody in PK-15 cells has revealed that 222 additional host proteins might interact with PCV2 capsid [116]. Host proteins that interact with PCV2 capsid might participate in a broad range of cell physiology including nucleic acid homeostasis, transcriptional regulation, several metabolic pathways, and innate immunity [116]. Among them, host proteins such as DExD-Box Helicase 21 (DDX21), eukaryotic translation initiation factor 4A2 (EIF4A2), heterogeneous nuclear ribonucleoproteins C1/C2 (hnRNPC), plasma protease C1 inhibitor (IC1), Importin-5 (IPO5), MX Dynamin Like GTPase 2 (MX2), NPM1, and snail family transcriptional repressor 2 (SNAI2) have been confirmed to be able to bind with PCV2 capsid in PK-15 by pull-down assays (Table 1) [116].
Several PCV2 capsid binding proteins including DDX21, EIF4A2, hnRNPC, IPO5, NPM1, and SNAI2 are often found in the nucleus [117-122]. DDX21 is a putative RNA helicase that can modify secondary RNA structure during RNA splicing or translational initiation [117]. EIF4A2 is a component of eIF4F translation initiation complex that exhibits RNA helicase activity [118]. The hnRNPC has a putative RNA-binding domain. It might participate in RNA homeostasis such as processing heterogeneous nuclear RNAs and stabilizing mRNAs [119]. IPO5 is a member of the importin beta family known to transport materials from cytosol into nucleus [120]. NPM1 is a nuclear protein. It is also identified as a PCV2 capsid binding protein in a previous study using a yeast two-hybrid library [79]. SNAI2 is a transcription factor that possesses a zinc finger domain. It can promote differentiation and migration of mammalian cells [121].
IC1 and MX2 are two proteins that can interact with PCV2 capsid. They are involved in innate immunity [122,123]. IC1 belongs to the serpin family of protease inhibitors that can suppress local inflammation by decreasing vascular permeability [122]. MX2 is one of the dynamin superfamilies of large GTPases. It is an interferon (IFN) induced GTPase that might prevent the assembly of progeny virus in host cells by blocking viral capsid transport [123].
PORCINE CIRCOVIRUS TYPE2 OPEN READING FRAME3
The ORF3 gene is about 315 bp in length. It is located within the ORF1 gene and transcribed in the opposite direction of ORF1 mRNA transcript (Fig. 1) [124]. The ORF3 encoding protein was firstly identified in PCV2 infected PK-15 cells [124]. A further genomic study has revealed that non-virulent PCV1 has a truncated ORF3 genome [125]. This may imply that ORF3 possibly encodes a virulence factor of PCV2. Indeed, three independent studies have demonstrated that PCV2 ORF3 is the major protein which induces apoptosis of host cells after PCV2 infection [37,124,126]. The proposed mechanism of how PCV2 ORF3 induces apoptosis is that ORF3 competes with p53, tumor suppressor, for the binding with Pirh2, an E3 ubiquitin ligase (Fig. 2A) [127,128]. Therefore, PCV2 ORF3 can increase half-life of p53 by blocking ubiquitin-dependent proteasomal degradation of p53 (Fig. 2A) [127,128].
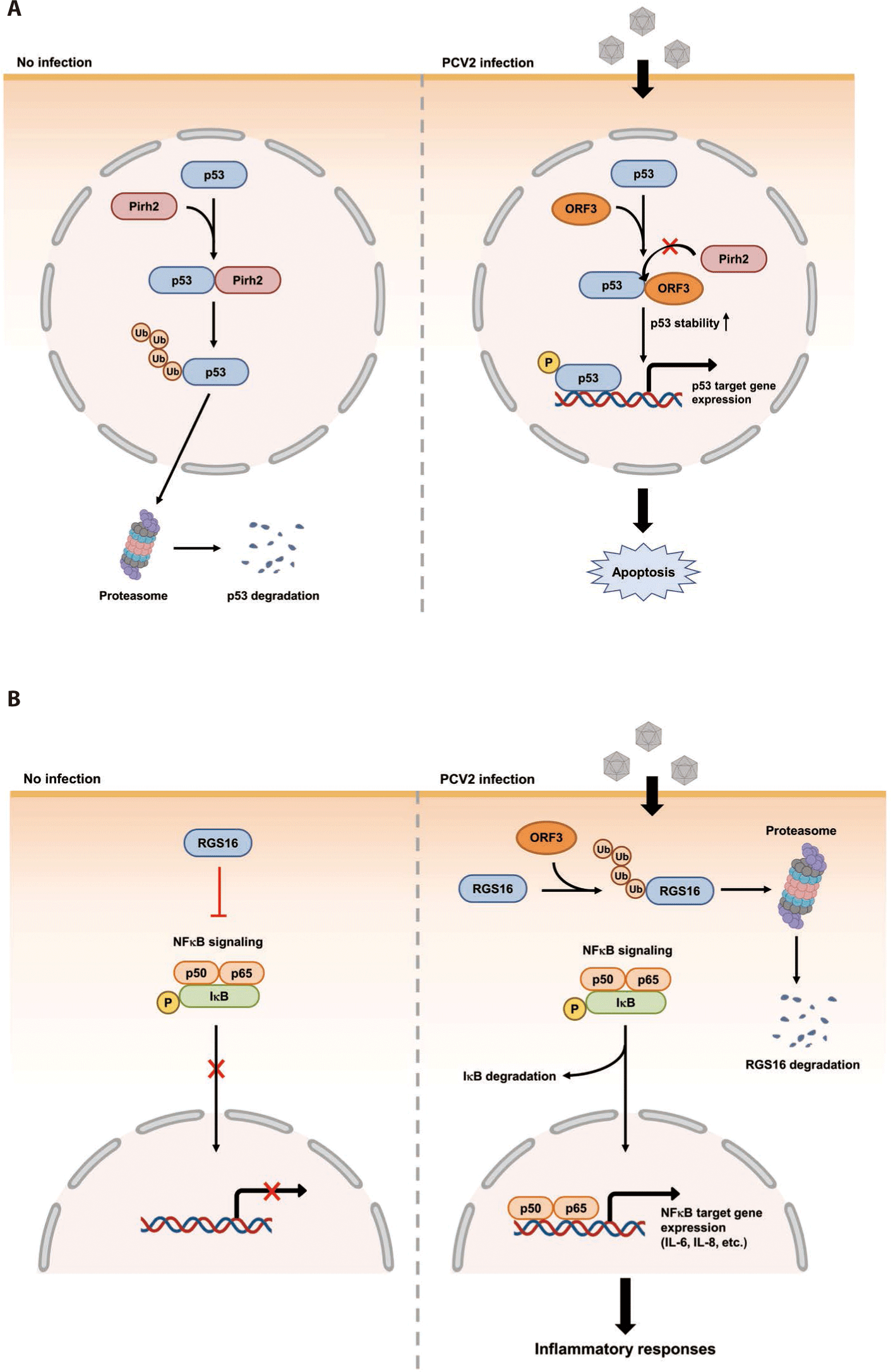
Since ORF3 protein can induce apoptosis of PCV2 infected host cells in vitro, several studies have attempted to estimate the pathological significance of ORF3 during a real PCV2 infection [129-131]. Initial two studies using PCV2 infected animals have revealed that ORF3-null mutant of PCV2 is less virulent in mice and piglets [129,130]. However, an independent study of PCV2 infection model later reported that clinical symptoms in piglets were not significantly changed when they were infected by ORF3-null mutant of PCV2 compared to those infected by wild-type PCV2, although ORF3-null PCV2 mutant infected piglets exhibited a delayed antibody production and lower serum levels of viral load [131].
Besides Pirh2, Tn10 transposase (DDE-like transposase) and regulator of G protein signaling 16 (RGS16) have also been identified as host binding proteins of PCV2 ORF3 by screening a bacterial two-hybrid library using cDNA of PK-15 cells (Table 1) [78]. Although the biological meaning of the interaction between Tn10 transposase and PCV2 ORF3 has not been documented yet, two independent studies have tried to find out changes of host cell physiology after interaction between RGS16 and PCV2 ORF3 [132,133]. The first study regarding interaction between RGS16 and PCV2 ORF3 confirmed the interaction between these two proteins by in vitro pull-down assay and showed that these two proteins were co-localized within nucleus [132]. The second study demonstrated that PCV2 ORF3 could facilitate proteasomal degradation of RGS16 by direct interaction [133]. Since RGS16, an inhibitor of large G protein signaling, suppresses the production of proinflammatory cytokines via attenuation of NFκB signaling, the degradation of RGS16 can lead to enhancement of NFκB translocation into the nucleus via the ERK1/2 signaling pathway and transcription of IL-6 and IL-8 in porcine epithelial cells, PK-15 cells (Fig. 2B) [133-135].
PORCINE CIRCOVIRUS TYPE2 OPEN READING FRAME4
The ORF4 gene is about 180 bp in length. It is located within the ORF3 gene and transcribed in the same direction as ORF3 mRNA transcript (Fig. 1) [38]. Initial studies using PCV2 ORF4 deletion mutant have revealed that PCV2 ORF4 might prevent apoptosis of host cells [38,136]. A further study using a yeast two-hybrid system has identified that PCV2 ORF4 can bind with ferritin heavy chain (FHC) (Table 1) [137]. FHC has a ferroxidase activity. It serves as an anti-apoptotic factor via suppressing the accumulation of reactive oxygen intermediates [138]. Therefore, PCV2 ORF4 might play a role in rescuing host cells from apoptosis by coordinating with FHC which removes accumulative reactive oxygen stress [138]. However, a recent study has argued that PCV2 ORF4 rather induces host cell apoptosis via interaction with mitochondrial adenine nucleotide translocase 3 (ANT3) [139]. In brief, PCV2 ORF4 possesses amphipathic α-helix targeting mitochondria at N-terminus and ANT3 can help ORF4 protein anchor to mitochondria [139]. After migration to mitochondria with an assistance by ANT3, PCV2 ORF4 could directly induce mitochondria-mediated apoptosis of host cells (Fig. 3) [139].
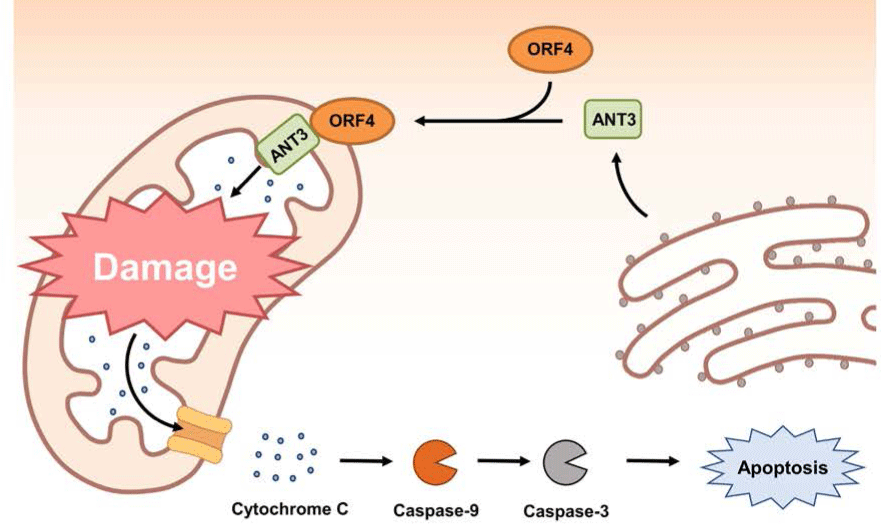
Other host proteins identified as ORF4 binding partners by yeast two-hybrid screening include cytochrome c oxidase subunit 8A (COX8A), lamin C, and small nuclear ribonucleoprotein polypeptide N (SNRPN) (Table 1) [137]. COX8A is the smallest component of COX (Complex IV), the inner membrane attached enzyme complex which participates in hydrogen (electron) transfer from the matrix to the intermembrane space in mitochondria [140]. Although COX8A deficiency can lead to severe impairment of COX stability, the relationship between COX8A and apoptosis is still elusive [141]. Lamin C belongs to type V intermediate filament. It is mainly detected in the nuclear lamina. It might participate in maintaining the shape of nucleus by providing nuclear interior or regulating gene expression via chromatin remodeling [142]. SNRPN is involved in pre-RNA processing including alternative splicing within the nucleus [143]. Further studies are needed to find out the biological significance of the interaction between PCV2 ORF4 and these proteins in host cells.
PORCINE CIRCOVIRUS TYPE2 OPEN READING FRAME5
The ORF5 gene is about 180 bp in length. It is located within the ORF1 gene. Its mRNA transcript is synthesized in the same direction as ORF1 (Fig. 1) [144]. The function of ORF5 protein is not necessary for PCV2 replication. However, ORF5 contributes to the pathogenesis of PCV2 by inducing activation of NFκB, induction of autophagy, and inhibition of type I IFN production in host cells [144-148].
An initial study of ORF5 function in host cells has revealed that ORF5 protein can induce ER stress, increase proinflammatory cytokine production by activation of NFκB, and inhibit host cell growth by increasing the S-phase of the cell cycle [144]. A subsequent study has revealed that PCV2 ORF5 protein can induce ER stress and unfolded protein response via the RNA-activated protein kinase-like ER kinase (PERK), activating transcription factor 6 (ATF6) and inositol requiring enzyme 1 (IRE1) signaling pathways [145]. After induction of ER stress by ORF5, PERK-eIF2α-ATF4 pathway can induce autophagy through autophagosome formation in host cells (Fig. 4) [146]. ORF5 expression in host cells can also enhance autophagy by inhibiting phosphorylation of mammalian target of rapamycin (mTOR) via activation of AMP-activated protein kinase (AMPK) pathway (Fig. 4) [146]. The reason why certain viruses can induce autophagy in host cells is still under debate. However, PCV2 has an advantage by inducing autophagy because PCV2 ORF5 also promotes viral replication [146,147]. In addition, PCV2 ORF5 might enhance replication of PCV2 in host cells by inhibiting type I IFN production via transcriptional repression of genes facilitating type I IFN expression or by increasing the expression of RNF128 which down-regulates type I IFN production [148,149]. Overall, PCV2 ORF5 can facilitate PCV2 replication in host cells by inducing autophagy and inhibiting host immune surveillance [144-149].
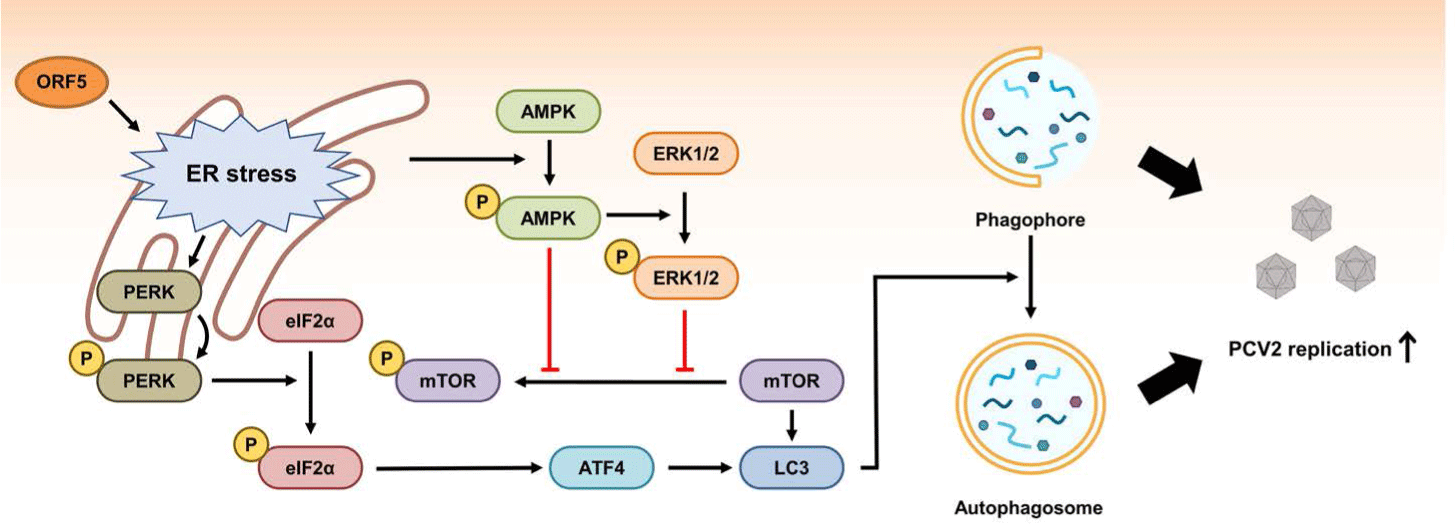
Five host proteins including cytochrome P450 1A1 (CYP1A1), glycoprotein-NMB (GPNMB), serine and arginine rich splicing Factor 3 (SRSF3), tyrosine 3-monooxygenase/tryptophan 5-monooxygenase activation protein β (YWHAB), and zinc finger protein 511 (ZNF511) have been found to be able to interact with PCV2 ORF5 by yeast two-hybrid screening (Table 1) [144]. Among them, both GPNMB and YWHAB can inhibit PCV2 replication by binding with ORF5 [150,151]. GPNMB might be involved in cell proliferation and anti-inflammatory responses [152]. Further studies are needed to elucidate the mechanism of how GPNMB inhibits PCV2 replication after interaction with ORF5. YWHAB can inhibit the function of M-phase inducer phosphatase 2 (CDC25B) which facilitates cell proliferation [153]. By interacting with PCV2 ORF5, YWHAB can prevent PCV2-induced autophagy and ER stress-mediated apoptosis [151].
PORCINE CIRCOVIRUS TYPE2 OPEN READING FRAME6
PCV2 ORF6 encoding region is located within ORF2. Its transcriptional direction is the same as that of ORF2 [34]. However, the detection of ORF6 encoding protein using an anti-ORF6 polyclonal antibody has confirmed that ORF6 can make its own polypeptide [34]. Therefore, it is important to know the regulatory mechanism of transcriptional variation between ORF2 and ORF6. There is no known host protein that can interact with ORF6. However, the replication of ORF6 deletion mutant of PCV2 is reduced in PK-15 cells compared to that of wild-type PCV2 [34]. Also, ORF6 possibly can regulate caspase activity and down-regulate the expression of proinflammatory cytokines such as type 1 IFN (IFN-β), IL-10, IL-12, and TNF-α in PK-15 cells based on an ORF6 deletion mutant study [34]. Further study is needed to determine which host protein can interact with PCV2 ORF6 and how PCV2 ORF6 modulates host cell physiology.
CONCLUSION
Interactions between PCV2 ORFs 1–6 and host proteins can trigger various phenotypic changes of host cells, including the induction of apoptosis and autophagy, or blocking anti-inflammatory responses. Currently, PCV2a vaccine can efficiently reduce the incidence and ameliorate the severity of PCVAD. The majority of PCV2 vaccines are based on inactivated PCV2 or subunit vaccines containing PCV2 capsid protein [5,19]. However, these vaccines could not cover all genotypes of PCV2 infection and might be a driven source of antigenic variation of PCV2. In addition to targeting PCV2 capsid, the identification of specific ORFs that control PCV2 infection by modulating host signaling network will be helpful for designing novel anti-PCV2 drugs that can selectively inhibit multiplication of PCV2 in host cells. These novel anti-PCV2 drugs are expected to aid in controlling PCVAD, which threatens the pig industry. Further studies are needed to define the proposed role of PCV2 ORF7–ORF11 or identify host proteins that interact with them.