INTRODUCTION
Summer heat stress (HS) is not only associated with a reduced milk production, but also with a lower fertility, including conception rate (CR), in dairy cattle [1,2]. The Korean peninsula, located in East Asia in the northern hemisphere, is experiencing severe climate changes, and in the summer of 2018 a continuously extreme heat wave with the highest temperatures (above 40°C) observed since meteorological records are available was recorded [3]. Particularly, high-producing cows such as the Holstein-Friesian breed (approximately > 99% the dairy cattle in Korea) are more susceptible to HS in terms of fertility, lactation, growth and disease [4–6]. HS can lead to reduced feed-intake and hormonal imbalance, resulting in a decrease in reproductive performance and birth weight of the calves in heat stressed pregnant cows. For instance, placental hormones especially estrone sulfate during the late gestation is decreased in heat stressed cows by placental dysfunction, leading to retarded fetal development [7]. Furthermore, survival rate before puberty and subsequent reproductive performance of these calves is reduced by impaired immunity [8,9].
From a cytological point of view, mitochondria are evenly distributed in cytoplasm of oocytes and produced adenosine triphosphate (ATP) under normal conditions. However, HS impairs the localization of mitochondria in oocytes. It affects ATP synthesis and therefore reduced ATP production is impairs oocytes maturation and, subsequently embryonic development. In additions, reactive oxygen species (ROS) is closely related to mitochondrial function. Increase of ROS levels above the required threshold by stressors are compromise the oocyte maturation [10].
In 2012, Jersey cow embryos were imported from Canada and the United States with the aim to improve the fat and protein contents of dairy products and to address climate changes, making dairy farms more sustainable. The Jersey breed was more tolerant to heat than the Holstein cattle in terms of milk production and reproductive performance [11,12]. For example, under HS conditions (temperature-humidity index [THI] > 68), the average daily milk yield significantly decreased from 35.6 to 34.2 kg/d in the Holstein cows, whereas it increased significantly in the Jersey cattle from 25.9 to 26.6 kg/d [11]. According to the reports of the U.S. Department of Agriculture, the Jersey breed had the highest reproductive performance (> 40%), including CR, compared to the Brown Swiss (~35%) and the Holstein cows (< 30%), due to their even performance throughout the year without a particular decrease in a specific season [12–14].
In this study, we first compared the CR of the Holstein and the Jersey cows in our institutional dairy farm and analyzed the association of the CR with the THI, calculated using information from a close local weather station. Subsequently, we studied oocyte maturation; oocytes were obtained from the same cows studied in the CR analysis, utilizing the ovum pick-up (OPU) technique. We focused on cytoplasmic oocyte maturation, as we had previously described several HS-related microRNA biomarkers [15] associated with the cytoskeleton, mitochondrial intracellular ROS, and ATP synthesis rate that can be used as indicators for oocyte quality [10].
The aims of this study were to investigate the effects of HS on the CR of the Holstein and the Jersey cows using public official meteorological data, and to assess the effects of HS on the quality of the oocytes obtained from both breeds used in the CR analysis. To the best of our knowledge, this is the first study to objectively explain why the fertility of the Jersey cows is higher than that of the Holstein breed under HS conditions.
MATERIALS AND METHODS
A retrospective epidemiological survey was performed using data from the CR of the Holstein and the Jersey dairy cattle in Korea. Animal data including cow identification, artificial insemination (AI) dates, parity, and pregnancy diagnosis results were collected from our dairy innovation center located in Cheonan, Korea (36°5967’ N, 127°1059’ E), from 2014 to 2021. The data were managed using Excel spreadsheets (MS Office 2021, Microsoft, Redmond, WA, USA). Ambient temperature (AT, °C) and relative humidity (RH, %) data collected from the closest official meteorological station (17.34 km; 36.7767 N, 127.1213 E; 24.89 meters above mean sea level) were used as a surrogate for on-farm environmental monitoring. The daily mean THI was calculated as follows [15–17]: THI = (0.8 × AT) + [RH × (AT – 14.4)] + 46.4.
All dairy cows were housed in a concrete floor facility, bedded with sawdust, and fed total mixed rations. Diet was formulated according to the guidelines included in “Nutrient requirements of dairy cattle (2001)” [18], and the cows were fed twice a day to meet their nutrient requirements. They were milked three times (interval 8 hr) with Lely Astronaut automatic milking system (Lely, Maassluis, Netherlands). Holstein and Jersey cows produced an average of 10,326 kg and 6,611 kg in 305 days, respectively. This study used a 2 × 2 crossover design (two dairy breed groups × two temperature values of in vitro maturation [IVM]), performing three consecutive OPU sessions, each separated by a 30-day interval, and conducting three replicates in each session. In all sessions, we evaluated the effects of HS on aspirated immature oocytes in the Holstein and the Jersey breeds by controlling the incubator temperature (38.5°C vs. 40.5°C) during the IVM.
Veterinarians at our dairy innovation center checked the medical condition of the animals before the start of each OPU session. Twelve dairy cows (Holstein-Friesian, n = 6; Jersey breed, n = 6) with ages between 2 and 4 years were deemed healthy to participate in the study. All cattle had body condition scores ranging from 3.0 to 3.25. They were managed in the same place and fed the same amount (2.5 kg) of hay twice a day, based on rice straw and tall fescue available for free to meet their nutrient requirements, as well as fresh water and minerals (mineral block) ad libitum. All experimental protocols performed on the animals were approved by the National Institute of Animal Science Animal Care and Ethics Committee in Korea (approval number: NIAS-068).
The selected animals were subjected to three consecutive OPU sessions with approximately a 30-day interval between each session, performed by the same operator in a cool season. On the day the OPU was performed, the animals were contained in a manual squeeze chute for cattle. All animals were administered a local anesthetic of 0.3 mL of 7 mg Xylazine (Rompun, Bayer Korea, Seoul, Korea) and 4 mL of 2% lidocaine HCl (Daihan Pharm, Seoul, Korea) before the procedure to ease the pain on the area and facilitate the handling of the ovaries through the rectum. The external area was cleaned using gauze soaked in 70% alcohol and dried with tissues. All visible ovarian follicles 2–5 mm in diameter were aspirated by transrectal ultrasonography using a portable scanner (4Vet Slim, Draminski, Olsztyn, Poland) with a 2–8 MHz convex array transducer, a 16-gauge needle, and a vacuum device. Aspirated immature oocytes were stored in 50 mL conical tubes containing 15 mL collection medium consisting of Hepes-buffered TCM 199 (Medium199, Sigma-Aldrich, St Louis, MO, USA) supplemented with 10% fetal calf serum (Gibco Life Technologies, Waltham, MA, USA), 50 ng/mL penicillin and streptomycin, and 10 IU/mL sodium heparin (Sigma-Aldrich) and were transported in a portable incubator at 37°C to be immediately moved to the laboratory for further in vitro experiments.
The retrieved cumulus oocyte complexes (COCs) were evaluated and classified into four grades according to their morphology: Good (the oocyte complex presents more than three compact and intact layers of cumulus cells and homogenous cytoplasm), regular (at least one layer of cumulus cells), denuded (partly or not covered by a cumulus cell layer), and atretic grade (dark cumulus oophorus and degenerated cytoplasm) [19]. After classification, the number of oocytes assigned to each grade was recorded, and atretic oocytes were discarded. Good, regular, and denuded oocytes were subjected to IVM. Retrieved immature oocytes were first washed three times with a commercially available washing media consisting of Hepes-buffered TCM 199 and other components (MKbiotec, Daejeon, Korea). The COCs aspirated in each OPU session were separated by breed (Holstein, Jersey) and divided into four groups according to the incubator temperature (non-HS [NHS]: 38.5°C, HS: 40.5°C) and the purpose of the staining (ROS, mitochondria). We set the NHS and HS conditions as 38.5°C and 40.5°C, respectively, based on the rectal temperature and the findings reported in our and other previous studies [15,20]. The COCs were transferred to a 4-well dish (Nunc, Roskilde, Denmark) containing 200 μL maturation medium (MKbiotec) supplemented with 10% fetal bovine serum (Sigma-Aldrich) and 1 μg/mL follicle stimulating hormone (Sigma-Aldrich) and were allowed to mature for 21 h at 38.5°C or 40.5°C, with a humid atmosphere of 5% CO2.
After IVM, the oocytes were washed three times with Hepes-buffered TCM 199 supplemented with 0.3% BSA (washing media, MKbiotec) and the cumulus cells covering the oocytes were removed by slow and gentle pipetting. Intracellular ROS in the denuded oocytes were stained with 5-(and-6-)-carboxy-2’,7’-dichlorodihydrofluorescein diacetate (carboxy-H2DCFDA, Invitrogen, Carlsbad, CA, USA), a reliable ROS fluorogenic marker in living cells. We added 25 μL 10 mM carboxy-H2DCFDA to 10 mL IVM medium based on Hepes-buffered TCM 199 (working solution) to 4-well plates (Nunc), and the denuded oocytes were then transferred to these plates and incubated for 30 min at 38.5°C, protected from light. Stained oocytes were observed with an inverted fluorescent microscope (ECLIPSE Ti-DH, Nikon, Tokyo, Japan). The NIS-elements BR version 4.30 imaging software (Nikon) was used to capture the fluorescent images. The mean green fluorescent (ROS) intensity of each oocyte was calculated using the ImageJ software version 1.53a (National Institutes of Health, Bethesda, MD, USA) by drawing a circle around the border of the oocytes.
After IVM, the oocytes were washed three times with Hepes-buffered TCM 199 supplemented with 0.3% BSA (washing media, MKbiotec) and the cumulus cells covering the oocytes were removed by slow and gentle pipetting. The mitochondria of the denuded oocytes were stained with MitoTracker Red CMXRos (Invitrogen), a X-rosamine derivative. We added 1 μL of 1 mM MitoTracker supplemented with DMSO to 10 ml maturation medium (working solution), for a final concentration of 100 nM, to 4-well plates (Nunc), and the denuded oocytes were then transferred to these plates and incubated for 30 min at 38.5°C, protected from light. After incubation, the oocytes were washed three times with washing media (MKbiotec) and then fixed for 60 min at 38.5°C in 2% paraformaldehyde. Subsequently, they were transferred to a glass slide with a 1 μL-drop of Dulbecco’s phosphate-buffered saline solution supplemented with 10 μg/mL Hoechst 33342 (Invitrogen); we waited for 5 min at room temperature (25°C) in the dark before observing the nucleus of the oocytes. Four very small and thin drops of vaseline were placed on the glass slide, pressed down gently with a cover glass and sealed with clear nail varnish. The mitochondrial distribution pattern of each oocyte was observed with a confocal laser-scanning microscope (Zeiss LSM 710 META, Zeiss, Oberkochen, Germany) and the objective patterns and intensity were measured along a line drawn across MII oocytes using the Line Profile Tool (line width = 20) from the ImageJ software version 1.53a (National Institutes of Health).
The percentage of first polar body extrusion and the ROS levels in oocytes at different IVM temperatures and breeds were analyzed by ANOVA using the SPSS 26.0 statistical software (SPSS, Chicago, IL, USA) after a descriptive statistics analysis to check the normality and homogeneity of the variances. p < 0.05 was considered statistically significant. The data were expressed as mean ± SEM. The association between each THI threshold value (50 to 78) and the CR was evaluated in a general linear model (GLM) using R version 4.0.5. [21]. Significance was set at a p-value less than 0.05.
RESULTS
We first checked the parities of the Holstein and the Jersey cows in our retrospective study because the parities of the cows affect the CR. Total 2541 and 474 of AI data were recorded for Holstein (n = 584) and Jersey (n = 106), respectively. The overall parity proportions were similar between the two breeds, although the Holstein cows had more primiparous animals (35%) than the Jersey cows (25%) (Fig. 1A). Moreover, the Holstein and the Jersey cows employed in this CR analysis under HS conditions (THI > 72) had a similar parity proportion (from 22.5% to 30% in heifers or in 1st and 2nd parity cows) (Fig. 1B).
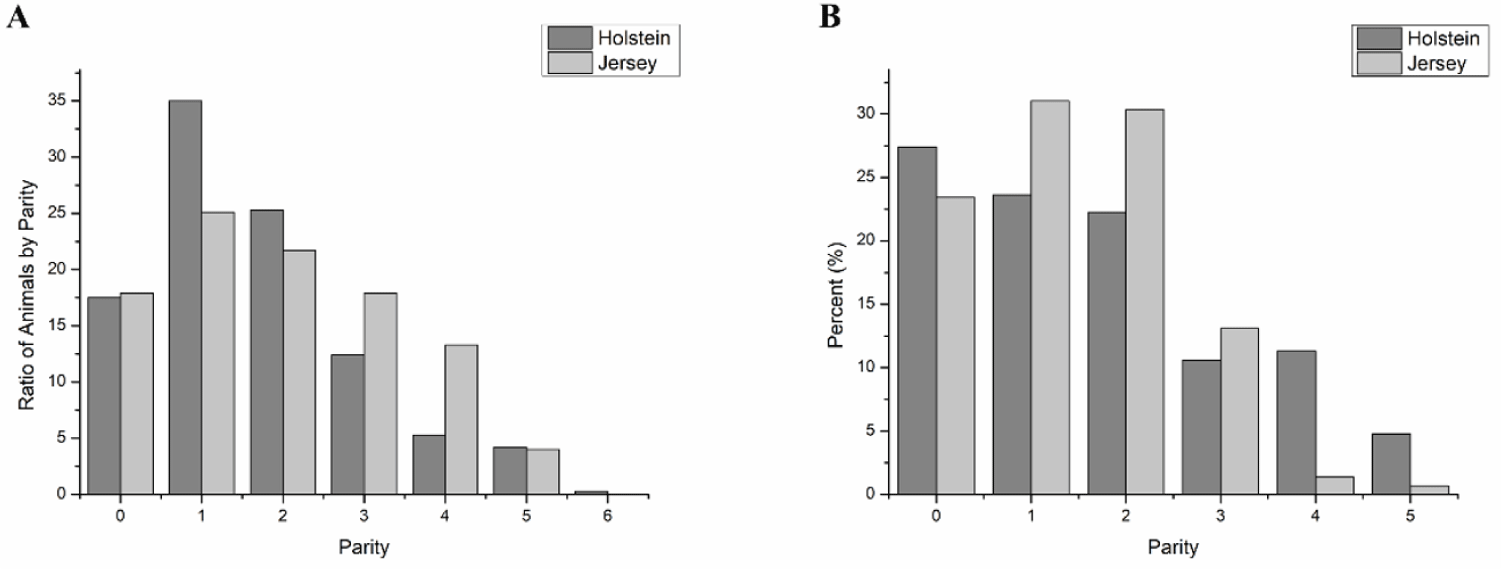
The maximum, minimum, and mean temperature values in this study were 36°C, −18°C, and 11.3 ± 9.8°C, respectively, and the maximum, minimum, and mean relative humidity values were 100%, 5%, and 68.8 ± 12.8%, respectively. The overall CR values were 30.0% in the Holstein cattle and 39.9% in the Jersey cows.
The CR was calculated from the herd reproductive data. We set a THI interval from 50 to 78 to determine the point where the CR decreased according to the mean THI on the day of AI. An increase in the THI threshold was associated with a gradual decline in the CR. In our GLM, the B slope started to decrease considerably at a THI threshold of 75 in both breeds. However, the decrease of the CR in the Holstein breed as the THI threshold increased was steeper than that of the Jersey breed (Table 1 and Fig. 2).
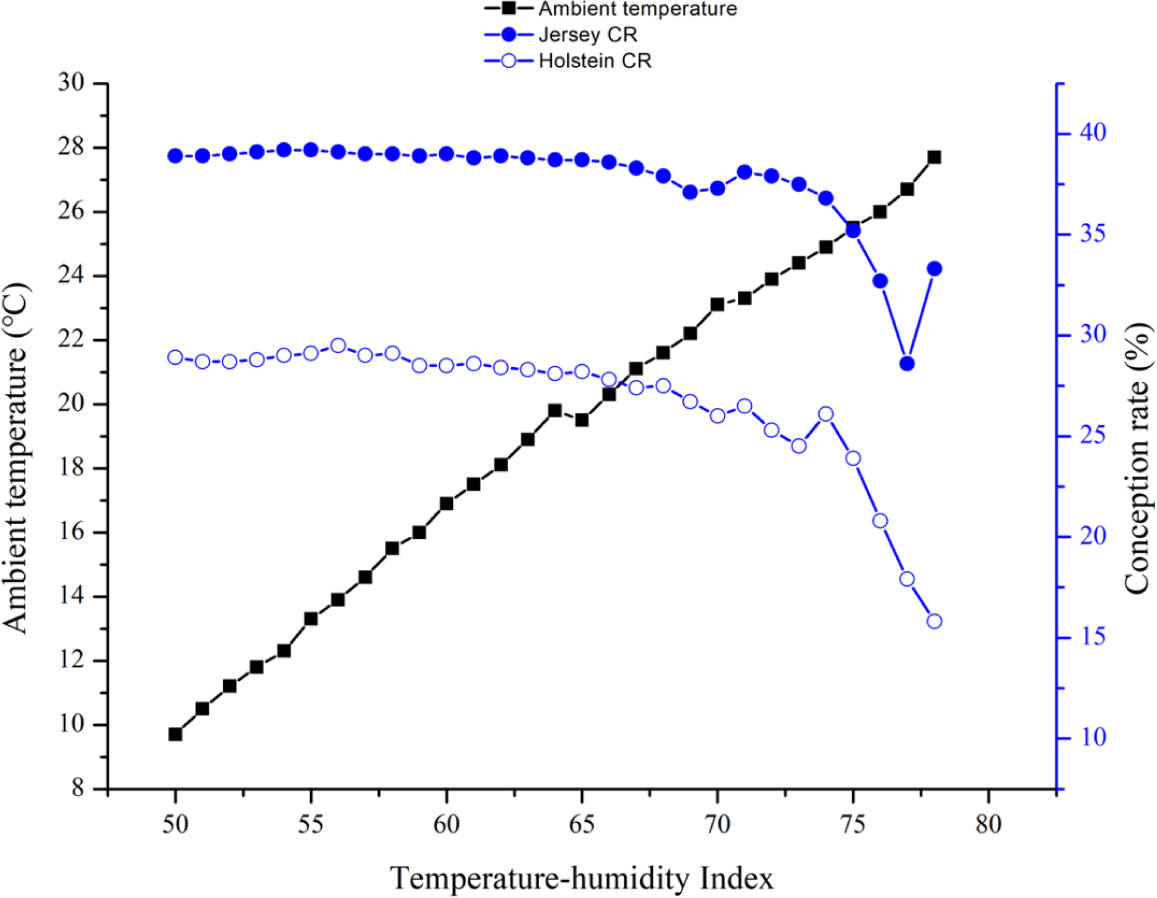
Germinal vesicle (GV) stage immature oocytes were retrieved by OPU from the Holstein and the Jersey cattle and were subsequently exposed to two different temperatures (NHS: 38.5°C, HS: 40.5°C) during their IVM. After 21 h, nuclear maturation was assessed by first polar body extrusion. There were no statically significant differences in the nuclear maturation rates between the two breeds or between the HS and NHS conditions. However, the rate was slightly lower in the Holstein cows (77.5%, p = 0.0521, Table 2) under HS conditions.
Breed | Temperature | No. of GV oocytes | No. of oocytes with the 1st PB (%) |
---|---|---|---|
Holstein | 38.5°C | 78 | 69 (88.4) |
40.5°C | 80 | 62 (77.5) | |
Jersey | 38.5°C | 59 | 52 (88.1) |
40.5°C | 64 | 53 (82.8) |
We selected in vitro-matured oocytes with a polar body and evaluated their intracellular ROS levels to determine the effect of HS on cytoplasmic maturation. The ROS levels differed slightly between the Holstein (19.30 ± 0.74) and the Jersey (16.46 ± 1.10) oocytes under normothermic conditions (p = 0.029). However, there was a greater difference in the ROS levels between the two breeds when their oocytes had been exposed to HS (40.5°C) during IVM, with the Holstein oocytes showing increased average ROS levels (32.20 ± 0.91) compared to the Jersey oocytes (20.34 ± 1.07) (p = 0.000). Interestingly, HS induced an increase in the intracellular ROS levels in the Jersey oocytes, but the levels were similar to those of NHS Holstein oocytes (Figs. 3A and 3B).
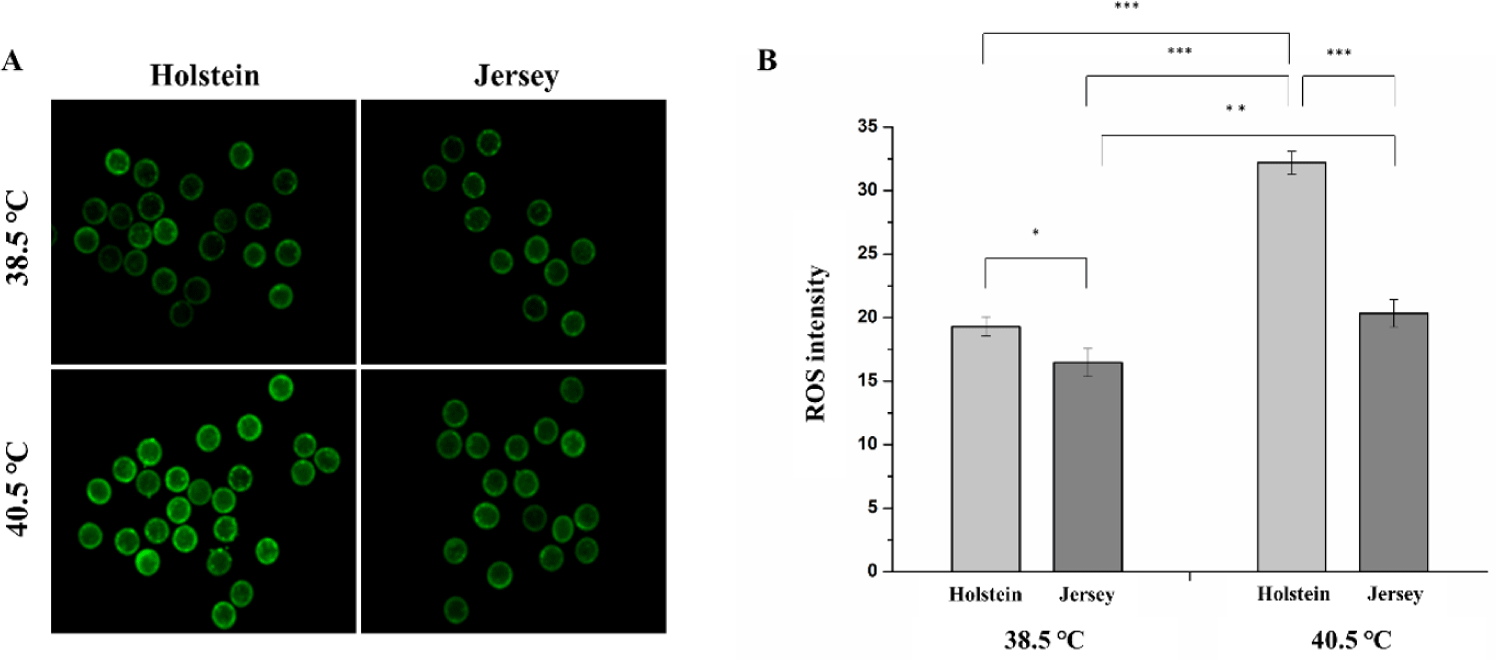
Subsequently, we investigated the effect of HS on the distribution of the mitochondria in matured oocytes via fluorescence intensity after MitoTracker Red CMXRos uptake. Overall, the intensities were not different between the breeds or the IVM conditions. Under NHS conditions, the mitochondria were homogeneously distributed in the matured oocytes, regardless of the breed. However, we observed two different patterns in the distribution of active mitochondria in the cytoplasm of the Holstein oocytes compared with the Jersey ones in the IVM under HS condition. The HS-Holstein oocytes showed a more intense peripheral distribution in the ooplasm, while the mitochondria of the Jersey oocytes were more homogenously distributed in the cytoplasm (Figs. 4A and 4B).
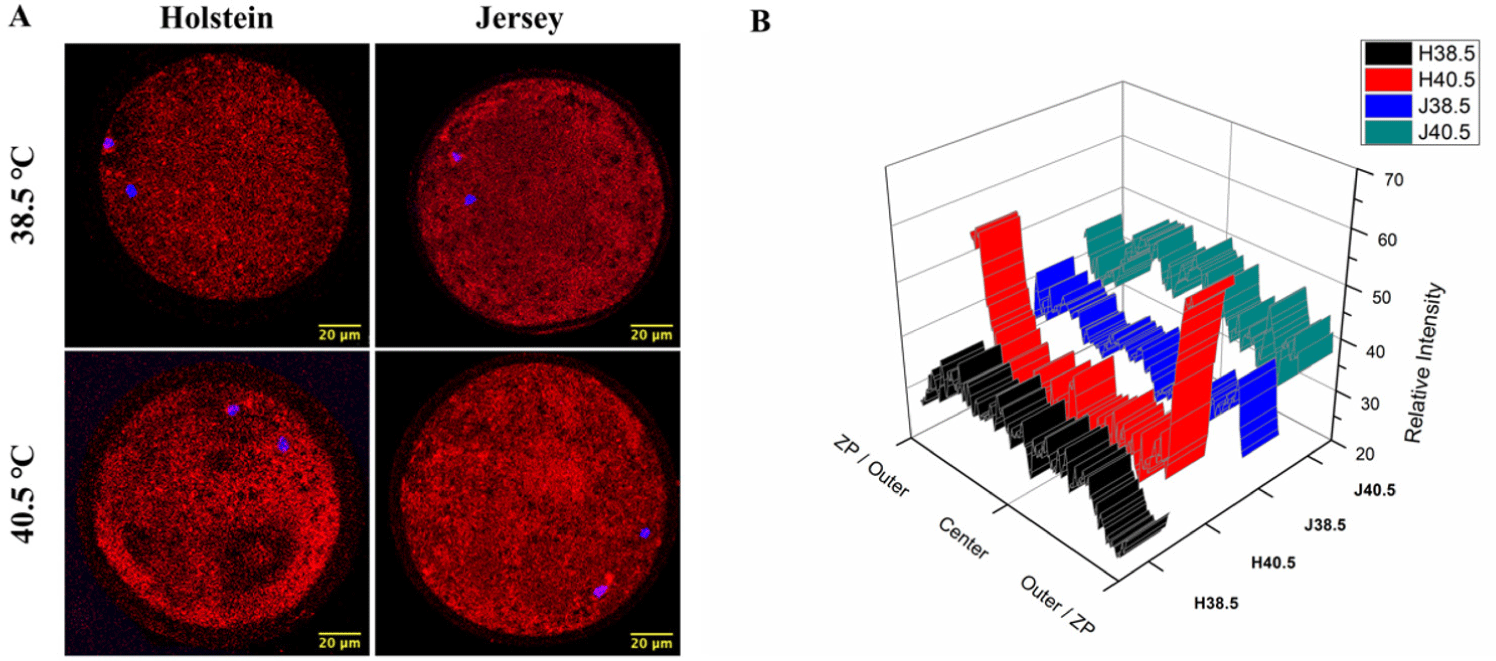
DISCUSSION
We first analyzed the CR of the Holstein and the Jersey cattle in Korea from 2014 to 2021, particularly focusing on the association between HS and the CR, and showed that the Jersey cows have a greater tolerance to heat in terms of their reproductive performance than the Holstein breed. In this study, THI values were calculated based on public data available from a close weather station to estimate the effects of HS on the CR of the Holstein and the Jersey cattle. The THI thus calculated were not accurate, as the weather conditions on-farm were different from those at the weather station, and HS can be underestimated [22]. However, several studies reported that the THI calculated from the weather station data could be used as a HS indicator [23–25]. In addition, the CR and the weather data were obtained from the same experimental farm and its closest weather station, and AI was performed always following the same procedures, suggesting that the weather data can be used to determine the THI threshold at which the CR is affected, and to compare the heat tolerance between the Holstein and the Jersey cattle. In our retrospective study, we found that the overall CR was approximately 10% higher in the Jersey breed compared to the Holstein cows. Interestingly, the differences in the CR between the Holstein and the Jersey cows increased as the THI increased, indicating that the two breeds may have different responses to HS in terms of oocyte or early embryo development.
The CR of dairy cattle that has experienced HS reportedly decreases by 20% to 30% [26], due to a reduced dry matter intake and an alteration of the hormonal balance, including circulating estradiol [27,28], luteinizing hormone, and progesterone levels [29,30], which lead to a poor oocyte quality [31,32]. We were interested in the oocyte maturation process as immature oocytes must undergo a correct nuclear and cytoplasmic maturation to develop into MII oocytes. To evaluate the effects of HS on the competence of the oocytes, we first examined the nuclear maturation by the 1st polar body extrusion, and we observed no significant differences between the breeds or the IVM conditions, although HS seemed to affect the nuclear maturation of the Holstein oocytes. Subsequently, we investigated the effects of HS on cytoplasmic maturation by observing the mitochondrial distribution and ROS levels. Nuclear maturation (extrusion of the 1st polar body) does not imply that the MII oocytes will also accomplish cytoplasmic maturation [33,34], as this involves the reorganization of organelles such as the mitochondria, that play a key role in determining the quality of the oocytes [33–35].
Here, we showed that the HS-exposed Holstein oocytes produced more ROS than the Jersey oocytes, suggesting that excessive ROS may lead to cytoplasmic defects and abnormal chromosomal segregation in the oocytes in the summer, as an imbalance between ROS production and the antioxidative defenses can induce DNA damage and apoptosis [10,36,37]. In addition, we also found a more intense peripheral distribution of the mitochondria rather than a homogeneous one in the Holstein oocytes exposed to HS during IVM. Previous studies reported that bovine oocyte quality can be classified into four grades according to the mitochondrial distribution pattern in the cytoplasm of their oocytes [10,38]. Moreover, mitochondria were homogeneously distributed in the cytoplasm of good-quality oocytes, whereas they were heterogeneously distributed and clustered around the periphery of the ooplasm in poor-quality oocytes [10,36,38]. Interestingly, the proportion of poor-quality oocytes that contained unevenly distributed mitochondria in their cytoplasm increased under HS conditions in the Holstein cows [39], as cytoskeletal elements such as microtubules and actin filaments in the oocytes are closely related to the mitochondrial distribution pattern, and also are highly sensitive to HS [10,39].
Our in vitro assessment of the nuclear and cytoplasmic maturation of the oocytes obtained by OPU can explain why the Jersey cows had a higher CR under HS conditions in our retrospective study, and our findings are consistent with the results of previous studies in which more ROS were generated, and the mitochondria were not homogenously distributed in the MII oocytes of the Holstein cows and other cattle after IVM under HS conditions [10,36,39].
This is the first report comparing the fertility of the Holstein and the Jersey breeds both in vitro (oocytes) and in vivo (CR) under HS conditions. In this study, we demonstrated that weather information from a local meteorological station can be used to calculate the THI threshold for the influence of HS on the CR of cattle, and that the Jersey breed is more tolerant than the Holstein one to HS in terms of their CR under HS conditions. Moreover, the incomplete cytoplasmic maturation of the Holstein oocytes exposed to HS, including an increase in ROS production and a peripheral distribution of the mitochondria, supports our observation of a lower CR of the Holstein cows with a THI over 75.