INTRODUCTION
The transition period is commonly defined as the period from 3 weeks before to 3 weeks after calving and is critically important to the health, production, and profitability of dairy cows [1]. For 3 weeks before calving, the nutrient requirements of the fetus reach maximal levels, but dry matter intake (DMI) decreases by 10%–30% [2]. Until the first 2 to 3 months in early lactation, the energy requirements for milk yield increase rapidly, exceeding the energy intake [3,4]. For these reasons, transition cows experience a negative energy balance (EB), which occurs when the energy requirement for a cow cannot be met by the energy she consumes. Under negative EB, mobilization of body reserves to compensate for this energy deficit is associated with altered metabolic status, greater incidence of metabolic diseases such as ketosis, displaced abomasum, mastitis and decreased fertility [4,5].
Many studies have reported that a dry period (DP) is optimal for 42 to 60 days [6]. Decreasing the DP is associated with the reduction of milk production in subsequent lactation [3,7,8]. Some researchers have reviewed the effects of shortening or omitting the DP on lactation performance, metabolic status, health and fertility of dairy cows [9,10]. For high-yielding cows with shortening or omitting of the DP, dairy cows have to produce less milk the next lactation but could obtain less metabolic load and negative EB in early lactation [3,4,11]. However, studies on the effect of omitting the DP when applied to transition cows under heat stress are very limited.
Heat stress is a major factor that negatively affects milk productivity, reproduction, health, and welfare, leading to economic burdens on dairy farms [12–16]. Lactating dairy cows have significantly more metabolic heat and additional heat from radiant energy and greater difficulty dissipating the heat stress compared with nonlactating cows [17]. Heat stress during the DP carries over into the postpartum period, which causes a decrease in milk production and negatively affects hepatic metabolism [18]. It is unclear whether omitting the DP abates the negative effect of heat stress pre- and postpartum and the subsequent performance. The objective of the present study was to investigate the effect of traditional DP (60-d DP) versus no DP (0-d DP) on EB, milk production, milk composition, and metabolic status of dairy cows exposed to heat stress from 8 weeks before calving to 10 weeks after calving.
MATERIALS AND METHODS
Holstein dairy cows were housed at the Department of Animal Resources Development, which is located at the National Institute of Animal Science (NIAS) in Cheonan, South Korea. All dairy cows were maintained as stated in standard guidelines, and the experimental protocol involved in this experiment was approved by the Institutional Animal Care and Use Committee (IACUC) at NIAS (study approval number: IACUC 2020-435). Multiparous dairy cows (n = 15; Parity 2.33 ± 0.47) were selected with expecting calving dates between June and July. All cows were randomly allocated to two different DP lengths: no DP (0-d DP, n = 7) or a traditional DP of 60 days (60-d DP, n = 8). All cows were studied from 8 weeks before expected calving to 10 weeks postpartum and experienced heat stress during the transition period. All cows yielded at least 15 kg milk per day at 8 weeks prepartum and had a healthy udder. Cows in the 60-d DP group received the far-off ration (total mixed ration [TMR]-1) from 7 days before drying-off, were milked once a day for 4 days before drying-off, and were administered an intramammary antibiotic therapy (Cloxagel® 500, Virbac, Carros, France) in each quarter during the last milking to dry off. During the DP, the cows were fed ad libitum TMR-1 ration, and received the producing lactation ration after calving (TMR-2) (Table 1). Cows in the 0-d DP group received the TMR-2 lactation ratio in the period before and after parturition. All cows were housed in an open loose barn, which was designed with the overshot roof of a ridge exhaust and fans to move and exchange the air in summer. The feed was offered once a day at 09:00 hr. TMR samples were taken monthly and stored at −20°C until analysis. Water was provided ad libitum.
TMR-1 was fed to cows with a 60-d DP from 7 days before drying-off to calving; TMR-2 was fed to cows with a 0-d DP during the prepartum and postpartum periods and to cows with a 60-d DP after calving.
NEL, net energy for lactation; calculated using NRC [19] according to the chemical composition of the dietary ingredients.
DMI was determined daily on an individual basis by subtracting the weight of the leftovers at 08:30 h the next day from the total amount of feed offered at 09:00 h each day. Rectal temperature (RT), respiratory rate (RR), and the surface temperature of rumen (STR) and udder (STU) were first measured at 13:30 h every week, blood samples were collected, and then body weight (BW) was measured. Daily milk yield was the sum of the morning (06:00 h) and afternoon (17:00 h) milking. The EB was calculated according the method of the National Research Council [19]. Net energy for maintenance (NEM, Mcal/d) was calculated as 0.08 × BW0.75. The energy requirement for milk production (NEL) was calculated as daily milk production (kg) × [(0.0929 × Fat) + (0.0563 × Protein) + (0.0396 × Lactose)]. Net energy intake (NEI) was estimated by multiplying feed intake (kg) with Mcal per (kg) according to the feed analysis of each delivered ration. EB was calculated as EB = NEI × (NEL + NEM).
Milk samples of individual cows were collected at each morning (06:00 am) and afternoon (17:00 pm) milking for one day every week. Milk samples were immediately analyzed with LactoScop (MK2, Delta Instruments, Drachten, The Netherlands). From the results of this analysis, fat and protein corrected milk (FPCM) was calculated as FPCM = (0.337 + 0.116 × Fat % + 0.06 × Protein %) × milk production (kg/d). Blood samples were taken once weekly at approximately 14:00 h from the last 8 weeks before expected calving until 10 weeks after calving in vacutainer tubes (containing heparin). Serum samples were decanted from the collected blood samples and stored at -20°C until later analysis. Serum was analyzed for glucose, urea, nonesterified fatty acids (NEFA), and β-hydroxybutyrate (BHBA) by a Blood Antoanalyzer (Hitachi 7180, Hitachi, Tokyo, Japan). Cortisol, insulin and insulin like growth factor (IGF)-I concentrations in serum were analyzed using the Bovine ELISA kit (Cusabio Biotech, Wuhan, China).
Ambient temperature (Ta, °C) and relative humidity (RH, %) were continuously measured every 30 min using a thermohygrometer (Testo 174H, Testo, West Chester, PA, USA) with an accuracy of ± 0.5°C and ± 3% RH. The thermohygrometer was installed in the feeding area at a height of 2.0 m above the ground. The temperature and humidity index (THI) was calculated based on the mathematical equation proposed by Mader et al. [20]: THI = (0.8 × Ta) + [RH × (Ta – 14.4)] + 46.4. The maximum and minimum means of Ta, RH, and THI were calculated from the maximum and minimum daily values, respectively.
Statistical analysis was performed using SAS Enterprise Guide 7.1 (SAS Institute, Cary, NC, USA). Data were analyzed separately for the pre- and postpartum data. One-way ANOVA was performed for differences in daily Ta (°C), RH (%), and THI between pre- and postcalving, the gestation period and birth weight of calf between 0-d and 60-d DP. Repeated measures ANOVA was performed for the differences in milk yield, milk composition, DMI, BW, EB, RT, RR, STR, STU, and blood metabolites using PROC MIXED. Prepartum, milk production and milk composition were analyzed for the 0-d DP treatment but were not available for the 60-d DP treatment. Milk yield, FPCM, and milk composition variables after calving, DMI, BW, EB, RR, RT, STR, STU, and blood metabolites variables were analyzed with DP length (0-d or 60-d DP), week (wk -8 to -1 for prepartum; wk 0 to 10 for postpartum), and the relevant interaction terms included in the model as fixed effects. For comparison of DP lengths, p values are presented after a Bonferroni adjustment. Duncan’s multiple range test was used to separate the means when significance was indicated. Differences were considered significant if p < 0.05.
RESULTS AND DISCUSSION
The environmental conditions, including Ta, RH, and THI, from -8 to 10 weeks relative to calving of dairy cows were similar during this study, as shown in Table 2. The daily average Ta and THI were higher in the postcalving period (26.02 ± 0.30°C and 76.22 ± 0.46, respectively) than in the precalving period (22.37 ± 0.31°C and 70.30 ± 0.53, respectively; p < 0.05), which indicates heat stress.
The actual DP was 56.13 ± 0.9 d (means ± SEMs) for cows with the conventional DP. Gestation period and calf birth weight did not significantly differ for cows with 0-d and 60-d DP (p > 0.05; Table 3). The birth weight of male calves tended to be higher in cows with 0-d DP (47.8 kg) than in cows with 60-d DP (42.8 kg; p < 0.10). Gulay et al. [21] reported no differences in calf birth weight for cows with 30-d and 60-d DP. Other studies reported that cows exposed to heat stress during the DP were reduced to several days (3 to 4 days) of gestation length and 6%–14% of fetal weight compared to nonstressed controls [22–25]. Heat stress during late gestation compromises fetal growth, which is caused by the small decrease in DMI of heat-stressed cows (10%–15%) [18,23–25]. As will be mentioned later (Table 4), these results may be related to the fact that the BW of calves born in the cows with 0-d DP was greater compared to cows with 60-d DP.
Item | 0-d DP (n=7) | 60-d DP (n=8) | SEM | p-value |
---|---|---|---|---|
Gestation length (day) | 276.86 | 277.87 | 1.63 | 0.776 |
Birth weight of calf (kg) | ||||
Female | 39.0 | 38.6 | 5.28 | 0.307 |
Male | 47.8 | 42.8 | 7.66 | 0.092 |
Total | 42.9 | 40.7 | 6.58 | 0.497 |
DMI during this experimental period was affected by the DP length, calving, and DP × calving (p < 0.05; Table 4 and Fig. 1). The DMIs of all DP lengths increased after calving compared with before calving (p < 0.05). The DMI at postcalving did not differ between 0-d and 60-d DP but was greater for cows with 0-d DP (17.55 ± 0.11 kg/d) than for cows with 60-d DP (14.98 ± 0.10 kg/d) at precalving (p < 0.05). BW was affected by the DP length pre- or postcalving (p < 0.05) and was significantly varied as it passed up to 10 weeks after calving (p < 0.05). EB decreased from precalving to postcalving in both DP lengths (p < 0.05; Fig. 2). At precalving, the EB of dairy cows with 60-d DP (4.64 ± 0.25 Mcal NEL/d) was higher than that of cows with 0-d DP (−0.55 ± 0.94 Mcal NEL/d) (p < 0.05). However, postpartum dairy cows with 0-d DP (−3.87 ± 0.68 Mcal NEL/d) had a lower EB reduction than cows with 60-d DP (−6.80 ± 0.65 Mcal NEL/d) (p < 0.05). Heat-stressed dairy cows reduce their DMI to decrease metabolic heat production and lead to large BW loss, which ultimately pushes the animal into negative EB [26]. In contrast, our study showed that BW change from prepartum to postpartum did not differ between cows in the 0-d or 60-d DP group (123.36 kg vs. 123.29 kg, respectively). Additionally, the average birth weight of calves tended to be higher in cows with 0-d DP (42.9 kg) than in cows with 60-d DP (40.7 kg; p > 0.10). These results were explained by Seyed Almoosavi et al. [27], who observed that heat stress in late pregnancy has a greater effect on fetal growth than on the BW loss of maternal cows. In this study, for postpartum, the EB of dairy cows with 0-d DP had a lower EB reduction than cows with 60-d DP, which is in line with previous studies [4]. The results showed that the more negative EB of cows with a 60-d DP after calving occurred earlier than in cows with a 0-d DP.
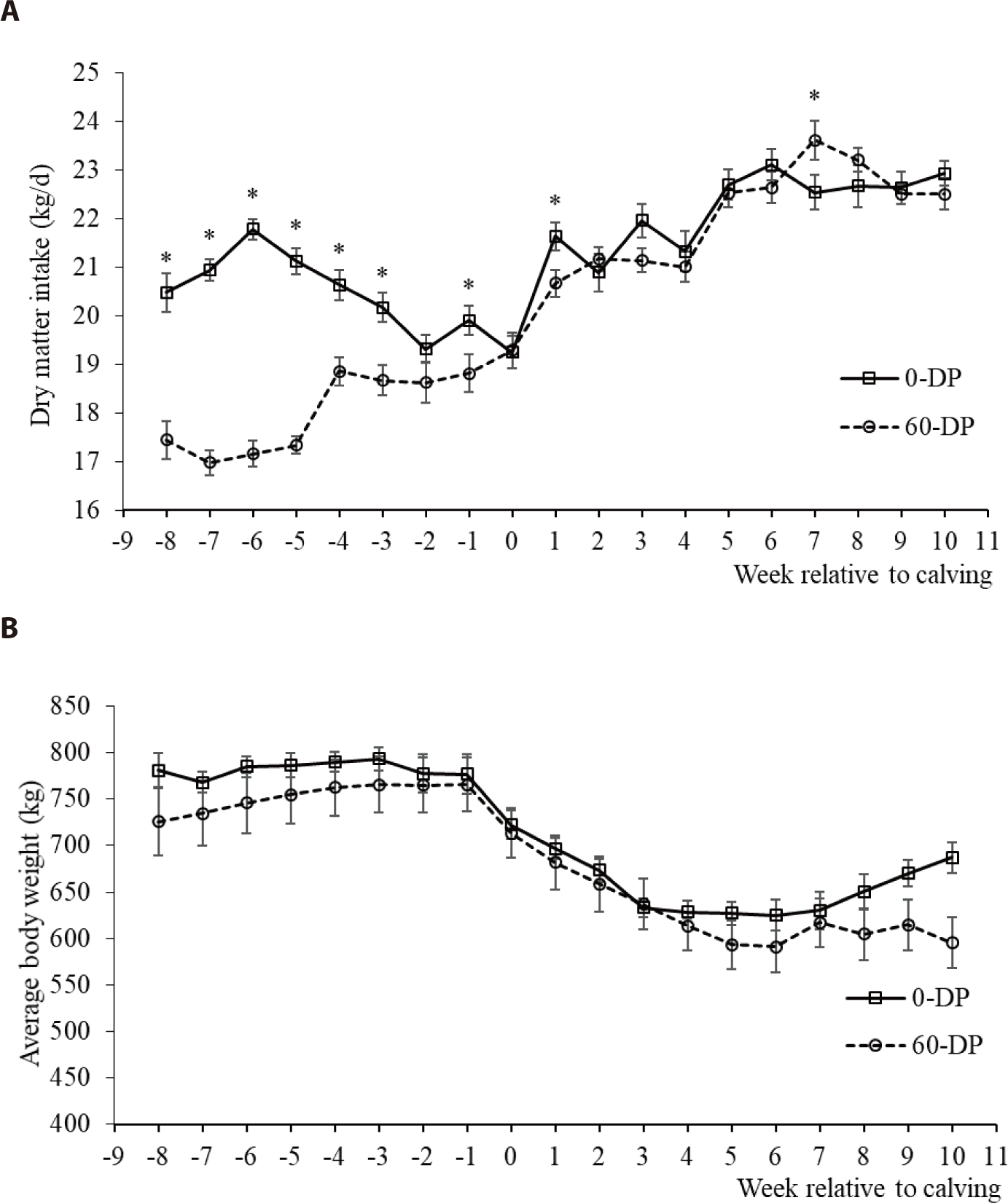
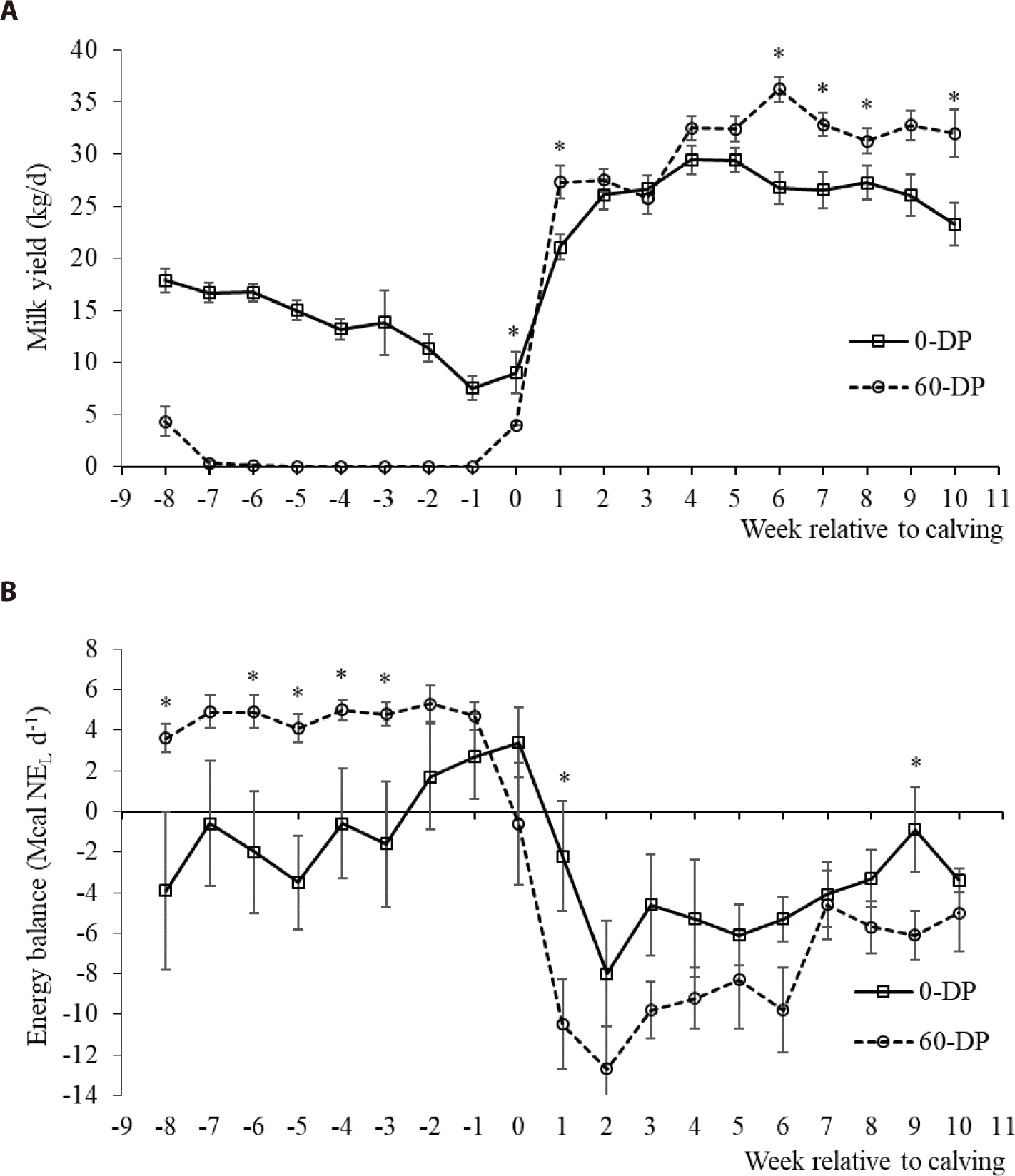
RT, RR, and the STR were higher in cows with 0-d DP than in cows with 60-d DP for the prepartum period (p < 0.05; Table 4) but were not significantly different for the postpartum period (p < 0.05). During the prepartum period, RT and RR increased with week relative to calving (p < 0.05). After calving, the RT, RR, STR, and STU decreased with week relative to calving (p < 0.05). The interaction of DP length with week relative to calving was present for the STU during the prepartum period (p < 0.05) and for the RR and STR during the postpartum period (p < 0.05). RT and RR increased when evaporative heat loss from the skin surface was not sufficient [28] and were the main physiological indicators of heat stress in dairy cows [18]. Also, West [17] reported that lactating dairy cows have significantly more metabolic heat and additional heat from radiant energy and greater difficulty dissipating heat stress than nonlactating cows. Regardless of the DP length or heat stress at late gestation, heat stress in early lactation had no significant effect on physiological responses, such as RT, RR, and ST, between cows with 0-d and 60-d DP.
Prepartum, dairy cows with a 0-d DP had an extra milk production of 14.85 ± 0.5 kg/d (p < 0.05; Table 5 and Fig. 2). The total milk yield was 648.4 ± 92.5 kg for cows with a 0-d DP in the last 8 wk before calving. Postcalving, the mean daily milk yield was lower for cows with 0-d DP (26.07 ± 0.5 kg/d) than for cows with 60-d DP (30.60 ± 1.0 kg/d) (p < 0.05; Table 6). However, total milk production from the last 8 weeks before calving to the first 10 weeks after calving was 628.5 kg greater for 0-d DP (2,529.3 ± 208.53 kg) than for 60-d DP (1,900.8 ± 102.54 kg). FPCM yield and milk lactose yield were greater, whereas milk fat and lactose contents were lower, in cows with 0-d DP compared to those with 60-d DP (p < 0.05). Milk yield, FPCM yield, and milk composition contents and yields were differenced with week relative to calving, and were an interaction between treatment and week relative to calving during the postpartum period (p < 0.05). Many studies reported that milk yield decreased for cows that had 0-d DP compared with a 60-d DP. The milk yield loss of cows with a 0-d DP resulted in an 11% decrease during 10 wk [3], or 15.5% until 14 wk [4] of the subsequent lactation lower milk yield compared with a short DP. Capuco et al. [29] explained that the milk yield loss after calving occurred to reduce renewal of mammary epithelial cells in the last weeks before calving when cows had a conventional DP. Milk yield losses of cows with omitting DP are partially compensated by the additional milk yield before calving within the range from 438 to 1,186 kg [3,7,8,10]. Boustan et al. [30] reported that accounting for additional milk yield before calving completely compensated for the loss of milk production resulting from shortening the DP to 30 days or 45 days in high-producing dairy cows under heat stress. Milk fat content tended to increase in the subsequent lactation for cows with a 30-d DP compared with cows with a 60-d DP [3], but milk fat yield was not different between 30-d and 60-d DP [3] or between 0-d and 60-d DP [31]. The milk protein percentage for cows with shortened or omitted DP is higher than that for cows with a traditional DP [3,4,30]. Watters et al. [32] reported that there was a significant difference in lactose content for cows with shortened DP compared with cows with 60-d DP.
Prepartum, the glucose concentration was lower for cows with a 0-d DP than for cows with a 60-d DP (p < 0.05; Table 6). Urea, BHBA, cortisol, and insulin concentrations prepartum were greater in cows with a 0-d DP than in cows with a 60-d DP (p < 0.05). NEFA concentrations at prepartum were significantly different from the week related to calving. Postpartum, urea concentrations were lower for cows with a 0-d DP than for cows with a 60-d DP (p < 0.05). Cortisol concentration was higher in cows with a 0-d DP than in cows with a 60-d DP (p < 0.05). Urea and NEFA concentrations at postpartum were significantly different from the week related to calving (p < 0.05). There was no difference in the interaction of the DP and the week related to calving pre- and postpartum. Negative EB occurs when the nutrient requirements of dairy cows exceed feed intake in early lactation [2]. Negative EB induces the mobilization of body reserves, such as body fat, in dairy cows [5] and is characterized by an increase in NEFA and BHBA [1]. Anderson et al. [8] reported that omitting DP reduced the concentrations of NEFA and BHBA. Other studies reported that DP length did not affect the concentrations of NEFA, BHBA, glucose, and urea [3,9]. Boustan et al. [30] reported that urea concentrations were higher and glucose concentrations were lower for cows treated with 60-d DP than for those treated with 30-d DP under heat stress, similar to the results of the present study. Gao et al. [33] suggested that decreasing glucose and increasing urea concentrations occurred by increasing the amino acid utilization of dairy cows under heat stress.
CONCLUSION
The current study examined the effect of DP length (0-d and 60-d DP) on physiological responses, milk production, and metabolic status of dairy cows exposed to heat stress during the transition period. It was found that dairy cows that continuously milked by omitting the DP (0-d DP) were more susceptible to heat stress during the DP, showing higher RT, RR, and surface temperature than dairy cows that went through the typical DP (60-d DP). Dairy cows with 0-d DP had lower milk production in early lactation than dairy cows with 60-d DP, but this early reduced milk yield of cows with 0-d DP was compensated by the milk yield produced before calving. Additionally, cows with 0-d DP improved their EB without any difference in heat stress in early lactation compared to cows with 60-d DP. From our results, the omission of the DP for dairy cows expected calving in heat stress may improve the EB in the early period. To support these results, additional research will be needed in heat stress-associated consideration of the appropriate length of the DP, party, and additives for milk production of dairy cows as well as greenhouse gas emission in the research of dairy science [34,35,36].