INTRODUCTION
Since the 1950s, antibiotic growth promoters (AGP) have been utilized as supplements to improve broiler productivity in terms of growth performance, including body weight (BW) and feed efficiency (FE) [1]. Nevertheless, concerns over residual or antibiotic resistance have resulted in the restriction of AGP usage in broilers and from early 2006, AGP were banned in many countries, including the United States, European Union members, and Korea [2–4]. Consequently, various alternative feed additives, such as organic acids and phytogenic compounds, were developed to replace antibiotics as growth promoters but none of them have equaled the efficiency of AGP yet [5–9].
Many studies were conducted to determine how antibiotics enhance broiler growth. Elimination of pathogenic gut microbiota via antibiotic action promotes energy utilization for growth [10]. Antibiotics also modulate gut development by enhancing mucosal immunity, feed digestion, and nutrient absorption [11–13]. However, the mechanisms involved in its growth-promoting effects remain unclear. The recent application of next-generation sequencing technique in animal nutrition has accentuated the capability of antibiotics to regulate gut microbiota, leading to an in-depth understanding of the mechanisms of antibiotic action in growth improvement in animals, as correlations were observed between the microbiota and biological phenotype by microbial sequencing. For example, AGP supplementation improved broiler weight gain and increased the ratio of Firmicutes to Bacteroidetes. Hou et al. distinguished the fecal microbiota composition between obese broilers and a divergently lean line [14]. Despite these studies, the link between the capacity of antibiotics to reduce the prevalence of enteric pathogens and the mechanism behind growth improvement remains obscure [2], which is essentially one of the reasons behind the complexity of developing alternatives for AGP.
In this study, we have focused on the novel glycolipid-type biosurfactant, named Sophorolipid (SPL). Recently, SPL has attracted much attention with its lower toxicity and more biodegradability compared to diverse biological detergents in various industrial fields including hygiene, medicine, and pharmacological fields [15]. SPL also could act as an antimicrobial agent, an immune modulator, a depigment agent, a wound healing accelerator, and a skin fibroblast stimulator [16–18]. Therefore, we conducted an experiment with SPL and zinc bacitracin to validate the possibility of SPL as a valuable replacement for AGP in livestock industry. ZB has been a commonly used antibiotic and it can improve the growth performance of broilers by alteration of intestinal morphology of broilers [19]. We aimed to investigate the effects of dietary supplementation with SPL on growth performance, intestinal morphology, nutrient utilization, and cecal microbiota of broilers.
MATERIALS AND METHODS
A total of 180 broiler chicks (1-day-old Ross 308) were obtained from a local hatchery. Their basal diets followed the requirements of the National Research Council and involved a three-phase (starter, grower, and finisher) feeding program for 35 days (Table 1). The chicks were randomly assigned to 3 treatment groups with 6 replicates (10 chicks/pen). The experimental groups for growth performance evaluation consisted of three dietary treatments: CON, basal diet; ZB, 100 ppm of ZB supplemented basal diet; SPL, 250 ppm of SPL supplemented basal diet. From days 35 to 38, Cr2O3 was added to their diets for subsequent collection of excreta. The chicks were fed and provided with water ad libitum and raised under the following environmental conditions: gradually decreasing temperature from 32°C to 24°C, average room humidity of 60% to 65%, and 20 h of light and 4 h dark, as instructed in the Ross 308 manual. The methods pertaining to the use of animals in this study were approved by the Institutional Animal Care and Use Committee (IACUC, KU-2020-0083) of Korea University.
Provided per kilogram of complete diet: vitamin A, 6,300 IU; vitamin D, 2,800 IU; vitamin E, 35 mg; vitamin K3, 1.75 mg; vitamin B1, 2 mg; vitamin B2, 6 mg; vitamin B6, 3 mg; vitamin B12, 13 μg; biotin, 0.1 mg; calcium pantothenic acid, 15 mg; folic acid, 1.5 mg; niacin, 50 mg.
The BW and feed intake were measured weekly for 35 days, and thereafter, 2 chicks from each pen were sacrificed with CO2 gas to collect the blood and small intestine. The weight and length of small intestine from each chick were measured. The duodenum, jejunum, and ileum samples were collected and fixed in 4% formaldehyde for histological analysis. Jejunal tissues were also collected for gene expression analysis and stored at −20°C until further analysis. On day 38, excreta of 2 chicks were collected and pooled per pen and the cecal contents were immediately frozen in dry ice until further analysis.
Serum from the blood sample was obtained by centrifugation at 12,000×g at 4°C for 15 minutes. Serum glucose, blood urea nitrogen (BUN), triglycerides (TG), and total cholesterol levels were measured using an analytical kit by spectrometric method (Embiel, Gunpo, Korea).
After storage, the ileal digesta were thawed, dried at 105°C overnight, and ground prior to the energy digestibility test, which was conducted using a bomb calorimeter. The apparent total tract nutrient digestibility (AD) and apparent metabolizable energy (AME) were calculated using the equations described by Fenton and Fenton as follows [20]:
The fixed jejunum samples were processed for paraffin embedding, sectioning, and hematoxylin and eosin staining for intestinal morphology analysis. Images were obtained using microscope camera (ToupTek, Hangzhou, Zhejiang, China). Villus height and crypt depth were analyzed at 10 × magnification per slide using ImageJ v1.49 (NIH, Bethesda, MD, USA) [21].
Frozen jejunal tissue samples were homogenized in TRIzol reagent (Invitrogen, Waltham, MA, USA) and the total RNA from each sample was obtained according to the manufacturer’s protocol. Subsequently, cDNA was synthesized by reverse transcription using a high-capacity cDNA synthesis kit (Applied Biosystems, Waltham, MA, USA). The relative mRNA levels of each gene was determined using StepOnePlus (Applied Biosystems) and normalized to β-actin using 2−ΔΔCt method [22]. The primer sequences used in this study are listed in Table 2.
qRT-PC, quantitative real-time polymerase chain reaction; GAPDH, glyceraldehyde-3-phosphate dehydrogenase; GLUT2, glucose transporter 2; SGLT2, sodium-glucose transporter 2; FABP1, fatty-acid binding protein 1; CD36, cluster of differentiation 36; DGAT2, diacylglycerol o-acyltransferase; FATP4, fatty-acid transport protein 4; PepT1, peptide transporter 1; CAT1, cationic amino acid transporter; rBAT, neutral and basic amino acid transport protein; y+LAT1, y + L amino acid transporter 1; ZO-1, zona occludens-1; CLDN1, claudin 1; OCLD, occludin; IL-1β, interleukin-1β; TGF-β, transforming growth factor β; IFN-γ, interferon-γ.
Microbial DNA was extracted from the cecal samples using QIAamp DNA Stool Mini Kit (QIAGEN, Hilden, Germany) following the manufacturer’s instructions. The DNA samples were used as templates for polymerase chain reaction (PCR) amplification by targeting the V3–V4 hypervariable regions of the bacterial 16S rRNA gene using the primers 341F (5′-TCGTCGGCAGCGTCAGATGTGTATAAGAGACAG-CCTACGGGNGGCWGCAG-3′) and 805R (5′-GTCTCGTGGGCTCGG-AGATGTGTATAAGAGACAGGACTACHVGGGTATCTAATCC-3′). Secondary amplification was performed by attaching the barcodes with i5 forward primer (5′-AATGATACGGCGACCACCGAGATCTACAC-XXXXXXXXTCGTCGGCAGCGTC-3′) and i7 reverse primer (5′-CAAGCAGAAG ACGGCATACGAGAT-XXXXXXXX-GTCTCGTGGGCTCGG-3′), with X referring to the barcode region. The PCR conditions were as follows: denaturation at 95°C for 30 s, annealing at 55°C for 30 s, polymerization at 72°C for 30 s with 25 cycles for primary PCR, and 8 cycles for secondary PCR. The PCR amplicons were purified using CleanPCR (CleanNA, Waddinxveen, Netherlands) and the quality and size of the products were assessed using 2100 Bioanalyzer (Agilent, Palo Alto, CA, USA). Equal amounts of amplicons from each sample were pooled and subjected to Illumina MiSeq sequencing (Illumina, San Diego, CA, USA) at ChunLab (Seoul, Korea).
The raw sequence data were analyzed using mothur v.1.38 [23]. The barcoded fusion primers and low-quality reads with read lengths below 300 bp were eliminated. Subsequently, the sequencing reads were compared using basic local alignment search tool (BLASTN) as a reference to remove chimeric sequences. Microorganisms were taxonomically identified using EzBioCloud database at the species level with a 97% similarity cutoff (ChunLab).
All values are presented as mean ± SE and were derived from triplicate experiments. Quantitative data were subjected to one-way ANOVA. Multiple comparisons were performed using Tukey’s honestly significant difference (HSD) test and the results were considered statistically significant at p < 0.05. Analyses were performed using the SAS 9.4 software (SAS Institute, Cary, NC, USA). The Wilcoxon rank-sum test was used to analyze the differences in alpha diversity indices and the taxonomy of microbial populations.
RESULTS
The BW of the chicks improved in ZB and SPL treatments on day 14, and both final BW (day 35) were significantly heavier compared to the CON group. Moreover, average daily gain (ADG) throughout the experimental period (days 1 to 35) also significantly increased in both ZB and SPL groups (Table 3). The final BW improved in ZB (16.54%), and SPL (14.59%) compared to that in the control (p < 0.05). Although their average daily feed intake (ADFI) did not differ among the treatments, their ADG significantly increased in ZB and SPL in every growth period except in the control (p < 0.05). This resulted in greater FE in ZB and SPL compared to that in the control during the overall period (day 0–35).
The levels of serum glucose, BUN, TG, and total cholesterol marginally differed among the treatments. However, TG levels in ZB and SPL treatment groups were significantly increased compared to that of CON group (Table 4). The concentrations of BUN, total cholesterol and glucose were not affected by dietary AGP and SPL supplementation in 35-day-old broilers.
The AD levels in the chicks did not vary considerably among the treatments (Table 5). The length and weight of each section of the small intestine were measured by histological staining (Table 6). Representative images of jejunum are shown in Fig. 1. The ratio of small intestine weight to BW did not considerably differ among the treatments, however, the intestinal weight per length was significantly lighter compared to the other treatments. Moreover, villus height, crypt depth, and villus height to crypt depth (V:C) ratio in the duodenum and ileum did not substantially differ among the treatments, however, villus height in the jejunum was significantly higher in the SPL treatment group compared to the other treatments. Relative gene expression levels of various nutrient transporters in the jejunum, namely glucose and amino acid transporters and fatty acid binding proteins are summarized in Table 7. The relative transcript levels of nutrient transporters in the jejunum were not differed among the treatments, nevertheless, expression levels of glucose transporters including GLUT2 and SGLT1 were significantly increased in ZB and SPL supplemented diet groups. mRNA expression levels of tight junction proteins were not different among treatments, however, the relative expression level of il-1β was significantly decreased by dietary SPL supplementation in broiler chickens (Table 8).
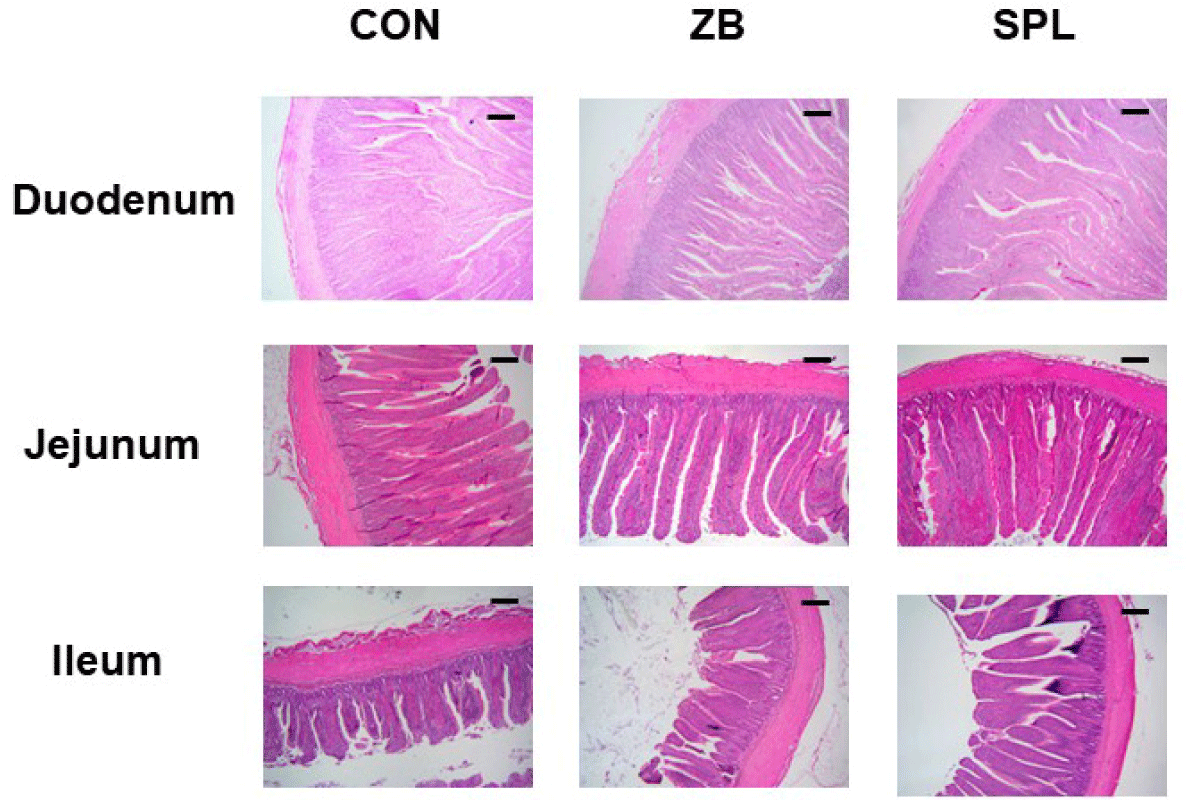
Treatment | CON2) | ZB | SPL | SEM | p-value |
---|---|---|---|---|---|
Energy digestibility (%) | 79.55 | 78.45 | 84.54 | 1.766 | 0.640 |
CON, basal diet; ZB, 100 ppm of zinc bacitracin supplemented group; SPL, 250 ppm of sophorolipid supplemented group.
GLUT2, glucose transporter 2; SGLT2, sodium-glucose transporter 2; FABP1, fatty-acid binding protein 1; CD36, cluster of differentiation 36; DGAT2, diacylglycerol o-acyltransferase 2; FATP4, fatty-acid transport protein 4; PepT1, peptide transporter 1; CAT1, cationic amino acid transporter; rBAT, neutral and basic amino acid transport protein; y+LAT1, y + L amino acid transporter 1.
At the phylum level, all the bacterial sequences were classified into 5 phyla; the ceca were dominated by Firmicutes (70.3%) and Bacteroidetes (26.7%). The population of Firmicutes increased by ZB supplementation, whereas that of Bacteroidetes concomitantly decreased. Consistent with the results of taxonomic composition analysis, the ratio of Firmicutes to Bacteroidetes increased in the ZB-supplemented groups relative to that in the control group. We further analyzed the cecal microbial taxonomy at the genus level (Fig. 2B). Of the 712 genera, the bacterial sequences were classified into 18 genera. The dominant genera in the ceca of the chicks were Barnesiella (23.47%) and Faecalibacterium (15.38%), which belong to Bacteroidetes and Firmicutes, respectively. The proportion of Barnesiella, Faecalibacterium, and JN713889 were increased by dietary SPL supplementation, and the population of Romboutsia, Blautia, Lactonifactor, and Turicibacter was decreased. On the contrary, dietary ZB addition decreased the portion of Barnesiella, Faecalibacterium, and Lactonifactor, but increased those of ZN713389, Turicibacter compared to the CON group.
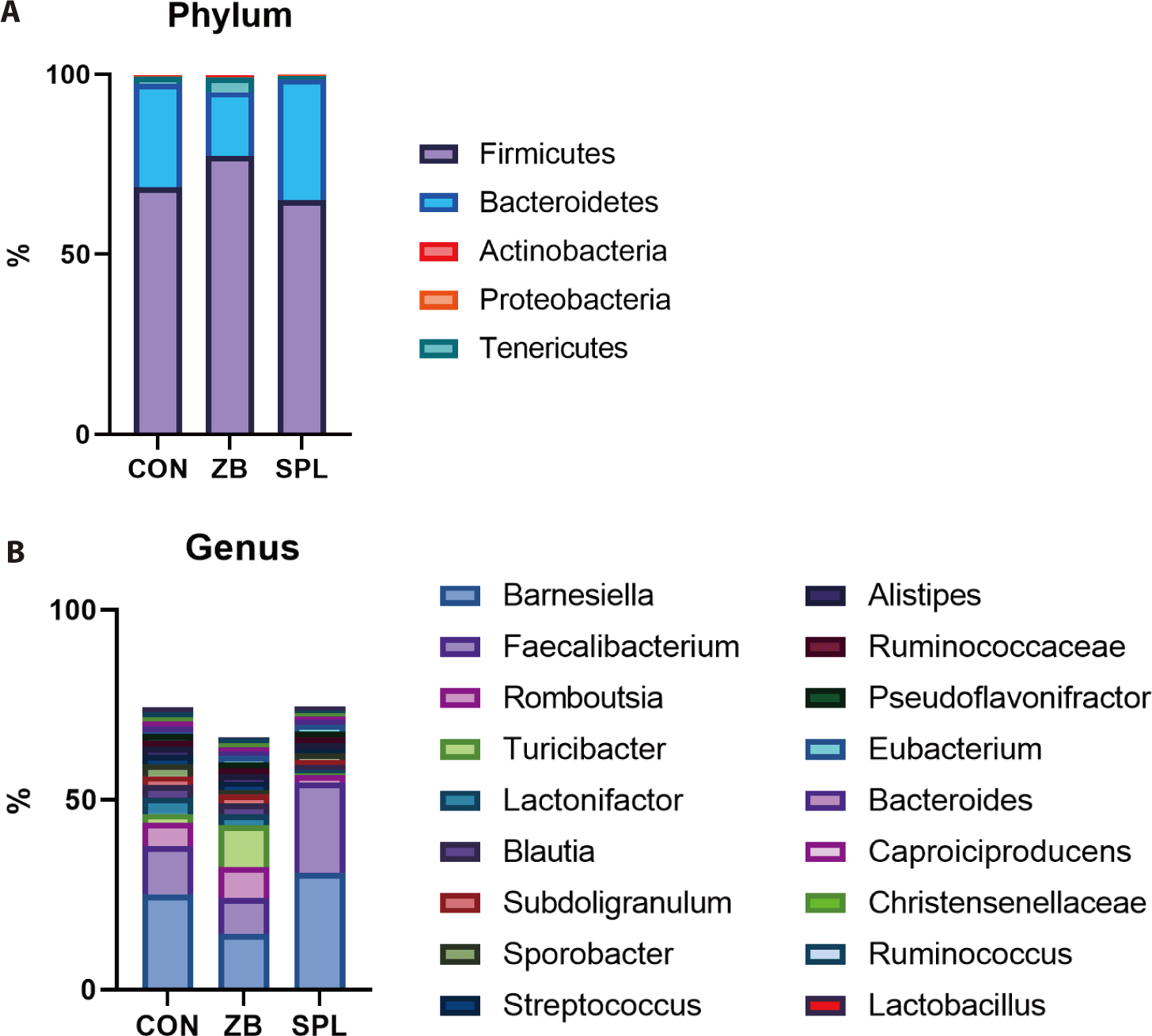
DISCUSSION
This study demonstrated that SPL and ZB supplementation possibly promotes growth and alters the cecal microbiota towards the dominance of Firmicutes relative to Bacteroidetes with upregulated jejunal glucose utilization and upregulated intestinal inflammation. Previous studies have reported the growth-promoting effects of SPL and ZB in poultry industrial field [11,15]. They commonly proposed that dietary SPL would be able to modify intestinal microbial population in a proper trend to promote gut microenvironments [15]. Particularly, this study indicated that SPL in broilers feed could increase glucose utilization capacity by upregulation of relative expression levels of carbohydrate transporters, and energy utilization including lipid metabolism in liver.
The results of biochemical analyses indicated that dietary supplementation with ZB and SPL did not affect the serum glucose, BUN, and total cholesterol levels in broilers. These data correspond to the findings of Manafi et al. and Attia et al., conversely, the concentration level of TG was increased by dietary addition of SPL in this study [19,24]. We assumed that dietary SPL could increase the carbohydrate uptake in jejunum by increment of expression level of transporters, including GLUT2 and SGLT, and it might lead to boosted lipid biosynthesis in liver metabolism.
We also evaluated whether the improved growth performance by SPL supplementation could be attributed to nutrient utilization and found that SPL supplementation showed increasing tendency in energy digestibility and significantly raised jejunal villus height of the chicks. On the other hands, it remains to be clarified whether antibiotics alter intestinal structure and function. In a study by Manafi et al., energy digestibility improved, and villus height was elevated with 50 ppm of bacitracin methylene disalicylate (BMD) supplementation, but their study differed from ours in terms of experimental duration; they examined the broilers 42 days after supplementation [19]. Nonetheless, our results are consistent with the findings of Miles et al., and Ao et al., as the villus height in their BMD-supplemented treatments did not differ from those of the control groups at 5 weeks [25,26]. Considering the variation in the forms of bacitracin in BMD and ZB, Daghighian et al. compared their corresponding effects on the growth performance of turkeys and found that BMD supplementation at 55 ppm increased BW in female turkeys, relative to those supplemented with the same amount of ZB at 4 weeks [27].
Previous studies have investigated the effects of AGP and SPL on cecal microbiota. We observed that ZB supplementation increased the population of Firmicutes, as opposed to Bacteroidetes, which is consistent with the findings of Gong et al. [11]. Furthermore, there was a remarkable shift in the population of Barnesiella at the genus level with a decline in Bacteroidetes. The functional role of Barnesiella is yet to be described in detail, but Wang et al. reported a negative correlation between the abundance of Barnesiella and the high-fat diet-induced metabolic syndrome phenotype, which is highly associated with increased BW. Some species of Barnesiella were found to produce acetate, suggesting that they suppress body fat accumulation [28,29]. Despite the indirect relationship, our results corroborate the potential of Barnesiella to attenuate weight gain in broilers.
Our findings indicate that a possible mechanism of SPL and ZB supplementation linked to increased nutrient absorption for growth improvement in broilers. Turnbaugh et al. reported that enhanced energy harvest and nutrient utilization via the modulation of cecal bacteria may be associated with the abundance of Firmicutes, which are consistent with our findings [30]. Numerical increment in the AD levels of SPL-supplemented chicks could be attributed to our experimental period, which was relatively sufficient for microbial populations to modify energy digestibility.
ZB and SPL has proven to be effective against gram-positive bacteria [15,31]. In contrast, we found that the population of Barnesiella, a gram-negative bacterium, dramatically decreased as ZB supplementation increased. Given that Barnesiella is the largest population of Bacteroidetes at the genus level in the chicken cecal content, ZB supplementation in our study may have largely affected Barnesiella among other populations [32]. A similar occurrence was observed in the study by Kim et al., in which tylosin, another antibiotic, decreased the gram-negative bacteria population despite the distinct ability of the antibiotic to target gram-positive bacteria. Further studies are needed to elucidate the role of Barnesiella in broiler energy utilization and nutrient metabolism [33].
Overall, the results of our study indicate that optimal dosage of ZB and SPL supplementation promote growth performance and modulate cecal microbial composition. Moreover, SPL supplementation may increase the glucose utilization capacity by upregulation of relative expression level of carbohydrate transporter and gut morphological index and lowering inflammation in jejunum.