INTRODUCTION
Corn is the major grain for concentrate feed in cattle diets. It is the primary energy source for livestock, and its primary constituent is starch [1]. Corn is surrounded by a hard pericarp, resistant to bacteria and enzymes; the starch-protein matrix in the granules is firmly bound, so it is degraded more slowly than barley and wheat [2,3]. However, the digestibility of corn can be increased through processing [1,4], and various processing methods have been suggested in this regard.
Processing methods can be classified as non-thermal and thermal. Non-thermal processing includes grinding and dry rolling, while thermal processing includes steam-rolling, steam-flaking, and roasting [5]. Grinding causes mechanical destruction of the pericarp, reduces particle size, and increases surface area, facilitating the attachment of microorganisms. It was reported that grinding increases ruminal starch digestibility by approximately 21%p compared to whole corn [6]. The improvement in starch digestibility increases as the degree of grinding increases (i.e., as the particle size decreases) [7–9].
Steam-flaking with high temperature and moisture also increases ruminal starch digestibility of corn grain. Steam-flaking, or simply flaking, also cracks the pericarp and starch granules to some extent, but more importantly, it gelatinizes the starch granules and increases degradation by bacteria and enzymes [3,4,10,11]. Flaked corn had a 30%p higher ruminal starch digestibility than whole corn [12], which can be more, depending on the degree of flaking [1,13]. Early studies that compared ground corn and steam-flaked corn in dairy or beef cattle reported that rumen starch digestibility was higher in steam-flaked corn than in ground corn [14–17], and feed efficiency was also higher in animals fed with steam-flaked corn [18–20]. Thus, it is commonly assumed that flaked corn is more degradable and provides more energy to ruminant animals than ground corn [21]. However, some studies have reported contrasting results that there was no difference in performance, such as growth and feed efficiency, between ground corn and steam flaked corn [22–25]. Thus, more comprehensive research is needed on the effects of flaking on the ruminal and total tract digestibility of corn and the consequent animal productivity. Furthermore, flaking corn requires additional costs, which increase as the degree of processing increases [26]. Therefore, the effect of degrees of flaking on the degradability of flaked corn also needs to be addressed.
The objectives of this study were two-fold: 1) to evaluate the effect of flaking on ruminal fermentability and degradability of corn grain and 2) to compare ruminal and total tract degradability of flaked corn with that of ground corn. In the present study, in vitro fermentation experiments, in situ degradability experiments, and in vivo metabolic experiments using goats were performed.
MATERIALS AND METHODS
This study was conducted at the Center for Animal Science Research, Chungnam National University, Korea. Animal use and the protocols for this experiment were reviewed and approved by the Chungnam National University Animal Research Ethics Committee (CNU-01022).
Seven flaked corn samples, along with whole and ground corn, were used in this study. Corn samples were obtained from multiple feed manufacturers to cover a wide range of flaking processes. Sampling was performed within a week to minimize seasonal and origin variations.
The dry matter (DM; ♯930.15), crude protein (CP; ♯927.02), crude fiber (CF; ♯962.09), and ash (♯942.05) content in feces, feed refusals, and experimental diets were determined as described by AOAC [27]. Ether extract (EE; ♯2003.05) content was determined as described by AOAC [28], and Ca and P (♯985.01) content was determined as described by AOAC [29]. The starch (a.k.a, naïve starch) content of feedstuffs was determined according to the Ewers polarimetric method (ISO 10520:1997). The extent of starch gelatinization was measured by analyzing the proportion of gelatinized starch to naive starch using an enzymatic method similar to that described by Zhu et al. [30]. More in detail, 0.5 g of ground corn with a particle size of 0.5 mm was boiled in 25 mL distilled water for 15 minutes and immediately cooled down. Another 0.5 g sample was added to 25 mL distilled water at ambient temperature for 15 min. Next, 10 mL of acetate buffer and 5 mL of starch saccharification enzyme solution were added to each beaker, and the samples were then incubated at 40°C for 70 min. After incubation, 5 mL of 25% trichloroacetate was added to cease the reaction, and the mixture was cooled for 10 min. After cooling, distilled water was added to a final volume of 100 mL, and the mixture was filtered through a filter paper (No. 5A). After the filtrate was transferred to a microtube, and the amount of glucose was measured using a YSI 2900 (YSI, Yellow Springs, OH, USA).
The effect of gelatinization of flaked corn on in vitro ruminal fermentation kinetics was assessed using an automated gas production system [31]. Gelatinization refers to the amount of gelatinized starch as a percentage of the naïve starch. The extent of gelatinization of flaked corn in each sample was 32% (Flake_32), 41% (Flake_41), 48% (Flake_48), 66% (Flake_66), 86% (Flake_86), and 89% (Flake_89) (Table 1). The samples were ground to pass through a 2 mm screen using a cyclone mill (Foss, Hillerød, Denmark). Subsequently, 0.2 g of the substrate was weighed into a serum bottle in triplicate for each treatment group, and 16 mL of in vitro buffer solution prepared as described by Goering and Van Soest [32] was added under strictly anaerobic conditions. After adding the anaerobic buffer solution, the bottles were capped with a butyl rubber stopper and sealed with an aluminum cap to maintain anaerobic conditions.
Before morning feeding, rumen fluid was collected from two non-pregnant and non-lactating Holstein cows, fitted with a permanent fistula. The animals were fed 700 g/kg corn silage and 300 g/kg commercial concentrate mix twice daily (Table 2). After collection, the two rumen fluids were mixed, placed in an icebox, delivered to the laboratory within an hour, and filtered through eight layers of sterilized cheesecloth and glass wool while maintaining strict anaerobic conditions. Subsequently, strained rumen fluid was transferred into a serum bottle and stabilized for 1–2 h in an incubator at 39°C. The substrate and in vitro buffer solution in the serum bottle were inoculated with 4 mL of the strained rumen fluid and incubated for 48 h.
The pressure generated by the gas accumulated in the headspace of the serum bottle was automatically measured every 20 minutes over 48 h of incubation using a pressure sensor. Fermentation kinetics were analyzed using a single-pool and single-lag exponential model as follows:
where Vt is the total gas production at time t (mL), t is the time after initiation of incubation (h), L is the discrete lag time (h), kd is the fractional rate of gas production (h−1), and Vmax is the asymptotic gas production (mL).
After 48 h of incubation, the pH of the fermentation fluid in the bottle was measured, and neutral detergent fiber (NDF), analyzed using a heat-stable amylase and expressed inclusive of residual ash, was determined to estimate true dry matter digestibility (TDMD) as described by Goering and Van Soest [32].
The in situ ruminal digestion experiment was performed using two cannulated, non-lactating Holstein cows. Whole-shelled corn (whole), ground corn (ground), and two unground intact flaked corns (62% gelatinized flaked corn [Flake_62] and 66% gelatinized flaked corn [Flake_66]) underwent in situ ruminal degradation. The intact corn samples were not ground to assess the degradability of the corn samples without reducing their particle size by mastication. Ground corn was ground to pass through a 2 mm sieve. Seven sets of triplicate nylon bags (10 × 20 cm, pore size 50 μm, Ankom Technology, Macedon NY, USA) containing 20 g (with a surface area of 15 mg/cm2) of the corn samples were placed in the ventral rumen of each Holstein cow. The bags were removed at the end of each incubation period (0, 3, 6, 9, 12, 24, and 48 h) and washed with cold tap water until the water appeared clear. Three bags from each time period were dried at 65°C for 72 h and weighed to determine in situ DM degradability (DM; #930.15) [28]. The starch content of the residue was also analyzed.
In situ rumen DM degradability was estimated by applying the time-series data to the model of Ørskov and McDonald [33] as follows:
where a is the soluble fraction, b is the slowly degradable fraction (potentially degradable), kd is the fractional rate of disappearance (per hourh), and t is the time of incubation (h).
The effective degradability (ED) was calculated as:
where Y is the potential degradability, a is the soluble fraction, b is the slowly degradable fraction, kd is the fractional rate of degradation, and kp is the fractional rate of passage (assumed to be 0.04, 0.06, and 0.08 h−1).
A total of 12 growing crossbreed goats (Korean native goat × Boer goat, 29.8 ± 4.37 kg, eight months old) were used for this study. A replicated-crossover design with three periods and three treatments was used. Each period lasted for three weeks, with 17 days for adaptation and four days of sampling. The wash-out period was set for one week between each period to minimize possible carry-over effects. During this period, goats were fed a control diet. Goats were housed in individual metabolic cages in an environmentally controlled animal research facility. All goats were male and castrated two weeks before the start of the experiment. Goats were randomly allocated and received different treatments during each experimental period. To counteract possible carry-over effects, the sequences of the treatment were randomized and balanced to increase the probability of every possible treatment [34]. Ground corn supplementation was used as a control. Ground corn was replaced by flaked corn (Flake_48, 48% gelatinized; Flake_62, 62% gelatinized) in the concentrate mix for other treatments. To meet the NRC [35] requirements for indigenous goat diets were formulated comprising 40% oat hay and 60% concentrate mix (Table 3). The goats were fed twice daily at 08:00 and 18:00 h, with half of the amount adjusted to achieve 10% refusal based on the intake of the previous day. Oat hay chopped to 5 cm was given before the concentrate mix at each feeding to make the goats consume forage as much as possible before ingesting concentrates. Drinking water was freely accessible to the animals throughout the experiment. Individual daily feed intake was recorded by measuring the feed offered and refusals.
Body weights were measured before morning feeding before and after each period. During the sampling period, feed refusals were collected daily, and each experimental diet (oat hay and concentrate mix) was sampled. Daily feed refusals and diet samples were pooled for each period and stored at −20°C until subsequent analysis. Total feces and urine from each animal were collected, weighed, and sub-sampled daily before the morning feeding during the sampling period. Urine was collected in a glass bottle containing 200 mL of 2.8% HCl and then stored at −20°C until N was measured as described by AOAC [28]. Sub-sampled feces, feed refusals, and experimental diets were dried at 60°C for 96 h and ground through a cyclone mill (Foss) fitted with a 1 mm screen before chemical analysis.
Data were analyzed using PROC MIXED (SAS Institute, Cary, NC, USA). Pair-wise comparisons of the least square means were conducted using the PDIFF option with Tukey–Kramer adjustment. Moreover, whenever applicable, a linear contrast between ground corn and flaked corn was performed. Statistical significance was declared at p < 0.05, and a trend was discussed at 0.05 ≤ p < 0.1.
The linear model used for the analysis of the in vivo experimental data was:
where Yijk is an observed dependent variable, µ is the overall mean, αi is the fixed effect of period, bj is the random effect of animal, τk is the fixed effect of treatment, and εijk is the unexplained error.
Based on the interaction between time period and treatment, the potential carry-over effect was not significant in any of the tested variables. This implied that the response of the goats to treatment was consistent during each period, even though different animals received individual treatment. Thus, the interaction term was omitted from the statistical analysis.
RESULTS
The in vitro fermentation characteristics and kinetic parameters after 48 h of incubation are presented in Table 4. The pH was significantly different between treatments and decreased as the degree of gelatinization increased (p < 0.01). According to the degree of gelatinization, TDMD did not significantly differ (p > 0.05). Although there was no significant difference in the maximum gas production (Vmax), the fractional rate of gas production (kd) linearly increased as the degree of gelatinization increased (p = 0.046). Moreover, the discrete lag time (lag) decreased linearly with increasing degree of gelatinization (p < 0.01). Consequently, the maximum volume of gas production (Vmax) reached quicker in the higher degree of gelatinization (Fig. 1).
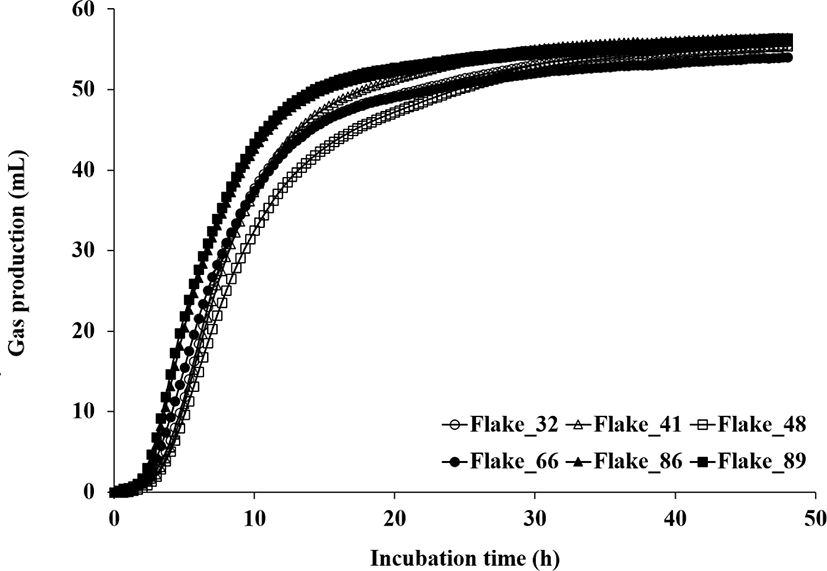
The in situ DM degradability of ground corn was significantly higher than that of the other treatments at all times, whereas DM degradability of whole corn was significantly lower than that of the other treatments at all times (p < 0.05) (Fig. 2). There was no statistically significant difference between the two flaked corn samples (p > 0.05). The same trend was observed for ruminal starch degradability. The starch degradability of ground corn was higher than that of the other treatments at all times, while that of whole corn was significantly lower than that of the other treatments (p < 0.05) (Fig. 3). There was no statistically significant difference in starch degradability between the corn flake treatments (p > 0.05).
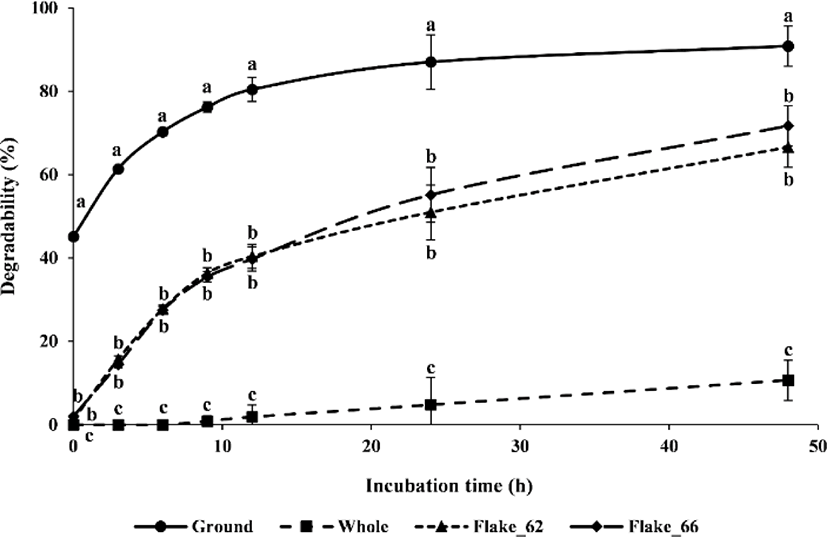
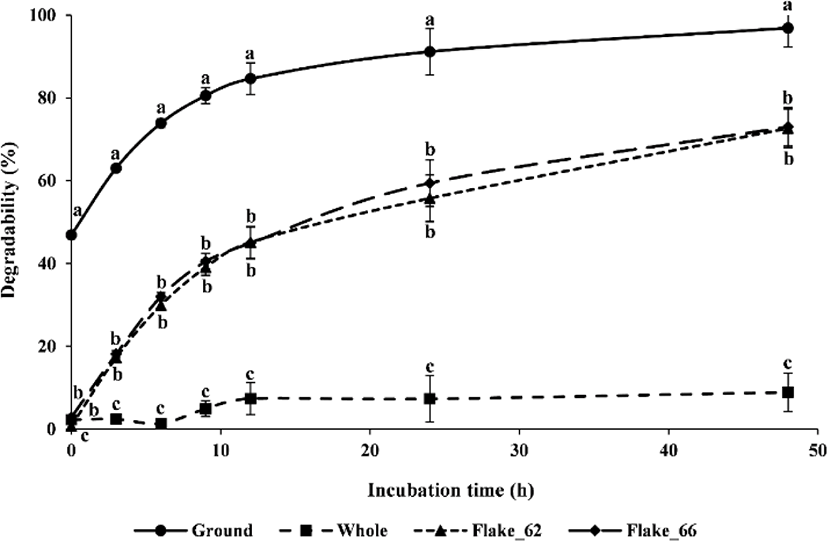
The degradation kinetics of the corn samples were assessed without whole corn as only limited degradation occurred with whole corn (Figs. 2 and 3). The proportion of the soluble fraction (fraction A) was greater in ground corn than in flaked corn (p < 0.001) (Table 5). The proportion of the slowly degradable (potentially degradable; fraction B) fraction tended to be greater in flaked corn than in ground corn (p = 0.07). The fractional rate of digestion (kd) tended to differ among the treatments (p = 0.07). Assuming the fractional rate of passage out of the rumen was 0.04, 0.06, and 0.08 h−1, the effective DM degradability was statistically significantly different (p < 0.001) for different corn processing methods (ground corn > flaked corn). There was no statistical difference in ED between the two flaked corns with different degrees of gelatinization (p > 0.05).
A, soluble fraction (% DM); B, slowly degradable fraction (% DM); kd, fractional rate of degradation (h−1).
With regard to rumen starch degradability, fraction A was the highest in ground corn (p < 0.001) (Table 6). There was no statistically significant difference between the flaked treatments (p > 0.05). The B fraction showed a statistically higher trend in the flaked treatment group than in the ground corn (p = 0.080). There was no significant difference in kd between treatments (p > 0.05). Effective starch degradability was higher in the flaked treatment than in the ground corn treatment at all assumed flow rates of 0.04, 0.06, and 0.08 (p < 0.01), and there was no significant difference between flaked treatments (p > 0.05).
A, soluble fraction (% DM); B, slowly degradable fraction (% DM); kd, fractional rate of degradation (h−1).
There was no statistically significant difference in average daily gain, DM intake, and feed efficiency among the treatments (p > 0.05) (Table 7). Crude fat intake was higher in the ground corn group than in the flaked corn group (p < 0.01). The apparent total tract digestibility of DM, organic matter, and ash did not differ between the treatments (p > 0.05) (Table 8). However, the digestibility of CF was different among the treatments; Flake_48 showed significantly higher crude digestibility than Flake_62 (p = 0.04). CP digestibility was different among the treatment groups, and the goats that were fed ground corn had higher CP digestibility than those fed with flaked corn (p = 0.03). Crude fat digestibility was significantly higher in the ground corn group than in the flaked corn groups (p < 0.01), even though crude fat intake was greater in control than flaking. Nitrogen intake and nitrogen excreted in urine did not differ between the treatments (p > 0.05) (Table 9). In addition, nitrogen retention and biological values were not significantly different (p > 0.05).
DISCUSSION
Flaking causes structural and physical changes in the starch granule and its matrix in corn, and it is widely accepted that flaking increases starch digestibility [3,4,10,11,36] and animal performance [18,19,26]. However, some inconsistent results also have been reported [22–25], and it is necessary to study the effect of flaking on the digestibility of corn compared to grinding.
The ruminal fermentability of flaked corn increased as the degree of starch gelatinization increased, based on the in vitro rumen fermentation study. This can be inferred from the results of a significant decrease in pH, increase in kd, decrease in the lag time as the gelatinization of corn starch increases. Ruminal pH is an good indicator of the production of organic acids from ruminal fermentation, and it decreases due to the rapid fermentation of starch [37,38]. Hales et al. [39] also showed that the pH decreased until 12 h of in vitro fermentation as the degree of steam-flaking increased (e.g., a decrease in bulk density from 386 g/L to 283 g/L). In their study, however, the pH at 48 h did not differ by the degree of flaking unlike the present study, which was probably because the pH of cultured fluid after 12 h in their study was already too low (e.g. < 6.0) to differentiate the treatment effect. An increase in the rate of degradation was consistent with Qiao et al. [40] which reported a significantly higher rate of degradation of corn with 78% gelatinization. A decrease in the lag time may be due to an enhancement of microbial attachement [41]. Steam-flaking destructs the most outer part of corn grain, pericarp. As the degree of flaking increases, the degree of destruction increases, resulting in more rapid attachment of microorganisms [14]. Hence, as the degree of gelatinization increases, attachment by microorganisms becomes more rapid, which makes corn more easily degradable.
Nontheless, a higher degree of flaking did not increase the total digestible portion of the flaked corn. The higher gelatinization did not alter the TDMD or the maximum volume of gas production (Vmax). Since gas production in the rumen occurs due to the degradation of feed by microorganisms, the gelatinization of starch does not increase its availability in the rumen. This is similar to a previous study by Kokić et al. [42] which reported no significant difference in organic matter digestibility as the degree of gelatinization increased from 21% to 64%. Therefore, an increase in the degree of flaking likely facilitates attachment of microorganisms and increases the rate of ruminal degradation of flaked corns without altering their potential of ruminal degradation.
The availability of corn was more affected by the particle size than the degree of gelatinization. Solubility of unground flaked corn is almost zero, which was reflected in the zero A fraction. Mainly because of this, the effective degradability of starch in flaked corn was 34.3%p–39.6%p less than that of ground corn even though insoluble but degradable fraxction (B fraxction) of flaked corn was 21.3%p higher than ground corn. Thus, particle size plays a significant role in ruminal degradability of corn grain, and without sufficient reduction in particle size, ruminal starch degradability of corn grain can be low even after intensive gelatinization. This result is consistent with previous studies. Lee et al. [11] reported that 1 mm ground corn had about 35% higher in situ DM degradability than intact flaked corn (not ground) after 48 h of in situ rumen fermentation. 4 mm ground corn showed a high degradability than intact flaked corn at 12 h and afterward, and eventually showed a 14%p higher degradability. However, in a previous in vivo experiment [43], calves fed with cracked (2.83 mm of mean particle size) and intact corns showed no significant difference in apparent digestibility. It was observed that the particle size is reduced through mastication by animals, and the reduced size helps to increase the availability. Another in situ rumen fermentation study reported that corn masticated by cattle also increased fraction A by about 4%p and fraction B by about 47%p compared to intact whole corn [3]. This is consistent with our result where, whole corn barely degraded, and the difference in degradability with ground corn was about 70%p (data not shown). Therefore, the grain particle size, which significantly influences the increase in grain availability, is considerably reduced by mastication, so the extent of mastication should be considered as an important factor in feed availability. However, the reduction in particle size by mastication may be a rate-limiting step in the digestion of flaked corn in the rumen, and if mastication is not sufficient to reduce the particle size of feeds, flaked corn may have lower availability than ground corn.
The nutritional value of ground corn might be higher than that of flaked corn. In the in vivo experiment, ground corn showed higher digestibility of EE and CP, and EE intake than flaked corn (Tables 7 and 8). The higher digestibility of EE and CP in ground corn implies that there is more potential value that can be used by animals. These differences in CP digestibility between ground corn and flaked corn is apparently caused by changes in the rumen availability of CP in corn during flaking. The amount of rumen degradable protein (RDP) decreases during flake processing [44–46], and this decrease in RDP alters the activity of microorganisms in the rumen and consequently affects the total tract CP digestibility [47]. In previous studies that used goats, CP digestibility decreases significantly as the amount of RDP in the diet decreases [48,49]. In addition to the high CP digestibility of ground corn, the high digestibility of EE appears to be affected by EE intake. Previous studies reported that the digestibility of fat increases quadratically as the intake of fat increases, which is consistent with our results [50–53]. However, the result of high EE intake in ground corn treatments contrasts with early studies that flaking increases the total tract digestibility of nutrients [16,19,54]. Others reported that feeding steam-flaked corn did not change fat intake [24,55]. Zhong et al. [24] conducted a study to substitute ground corn in the feed with flaked corn using lactating Holstein cows, but there was no difference in fat digestibility according to the substitution level. Rastgoo et al. [55] compared the nutrient digestibility of Holstein dairy calves fed with ground and steam-flaked corn and observed no difference in fat digestibility. However, Joy et al. [56] reported a decrease in fat intake, similar to our results but nonetheless, stated that the reason for this was unclear. Most of the previous studies focused on CP or starch for flaked corn and ground corn, and since the research results on fat intake are not clear, further studies are needed to determine the cause for differences in fat intake.
To summarize, an increase in gelatinization through more intensive flaking increased the rate of rumen fermentation of flaked corn but did not increase the total ruminal available fraction. Compared with ground corn, flaked corn may be less digestible without sufficient particle size reduction by mastication, leading to lower total tract nutrient digestibility. Considering the additional processing cost of flaking [57], feeding flaked corn may not be a beneficial feeding practice, especially when sufficient chewing is limited (e.g., a high intake level and feeding for a short period).
In conclusion, although an increase in gelatinization enhances the ruminal fermentability of flaked corn, flaking by itself may not sufficiently improve corn’s degradability compared to grinding. Without proper particle reduction by cracking or chewing, ruminal degradability and total tract nutrient digestibility of flaked corn may be lower than that of ground corn. Therefore, unlike the common assumption that flaked corn provides more energy to ruminant animals than ground corn, the energy content of flaked corn needs to be discounted when mastication is limited, for example, at a high intake level.