INTRODUCTION
Greenhouse gas concentrations in the atmosphere increased dramatically after the industrial revolution, and continued to increase to this day, leading to a rise in the average atmospheric temperature of the earth. According to the IPCC [1], rising temperatures in the atmosphere have enhanced global environmental changes and increased damage from climate change. Atmospheric temperatures are also predicted to continue rising due to global increases in greenhouse gas concentrations [2]. As a result, climate change has a large impact on livestock raising conditions, resulting in lower livestock productivity and economic hardship to farmers, and this trend will continue [3–5].
Increasing heat stress (HS) in livestock typically reduces feed intake (FI) and affects pig metabolism, leading to lower productivity [6]. According to Huynh et al. [7], reduced FI is the most consequential change in heat shock pigs as it decreases nutrient intake and growth. In Korea, livestock mortality reported from HS was 6,871,000 in 2018, an increase of ~368% from 1,867,000 in 2012 [8]. Pig FI decreases by 40 g for each 1°C above the thermal comfort zone, and the total quantity of heat is the heat produced by the body plus heat absorbed from the environment [9].
The mechanisms that account for the altered hierarchy in gradual tissue growth rates during HS are not fully understood in pigs. Metabolomics enables the identification of thousands of metabolites, providing the tools to determine molecular mechanisms of animal and human responses to environmental stress and the reason for the nutritional imbalances [4,10]. Metabolomics investigations have suggested that metabolites are potential biomarkers for observing animal stress and welfare [11]. Pigs are known to have an energy metabolism imbalance in their body when subjected to HS, which leads to a higher fat accumulation than protein accumulation [12]. This is because pigs respond to HS by altering several metabolites to sustain homeostasis in their body [12]. Nuclear magnetic resonance (NMR) based metabolomics has been used to effectively examine both long- and short-term effects of stress on the metabolic profile [13].
According to Pearce et al. [14], postabsorptive modifications to nutrient allocation in some heat-stress models reveal abnormal metabolic alterations in thermal-neutral (TN) animals on the same type of limited plane of nutrition. Wu et al. [15] found that several amino acids (AA) (e.g., Arg, His, Thr, and Met) seem to help restore the intestinal epithelia and eradicate reactive oxygen species (ROS) [16]. After pigs are exposed to HS for even a short period (less than 1 day), the AA metabolite levels change in pigs re-exposed to ambient temperature, although the ones that change may vary with different levels of HS.
The exact metabolic pathways by which HS affects pigs are currently unknown. There are also no accurate studies regarding protein accumulation and AA metabolism from plasma samples of pigs exposed to short-term HS. Moreover, little information on the effect of various recovery times following acute HS on plasma AA profiles and metabolites in growing pigs is available.
Therefore, the current study assessed the effects of short-term HS on free AA content and plasma metabolites in growing pigs using NMR. The ADFI (average daily feed intake), ADWD (average daily water drank), ADWL (average daily water loss), and RT (rectal temperatures) were also recorded to better understand metabolic changes during HS and HS recovery.
MATERIALS AND METHODS
The protocols used for the animal experimental procedures were reviewed and approved by the Institutional Animal Care and Use Committee of the National Institute of Animal Science (No. 2017-245).
This experiment was conducted in a controlled-environment room at the National Institute of Animal Science. The study used 6 castrated, male (Landrace × Yorkshire), 8-week-old growing pigs with an initial average body weight of 32.8 ± 4.1 kg. Before the experiment began, all pigs were kept in metabolic cages (2.1 m × 1.4 m) for 3 days in a controlled-environment room (20°C temperature and 80% relative humidity) to adapt. During the adaptation period, all animals were provided free access to feed and water. This experimental diet was formulated according to the nutritional requirements suggested by the Korean feeding standard for pigs as shown in Table 1 [17]. During the experimental period, all pigs were exposed to 36°C and 60% relative humidity for 24 h (HS), then returned to 20°C and 80% relative humidity. Thereafter the temperature and relative humidity were kept constant: 2-d TN recovery following HS (TN[2D]) and 5-d TN recovery following HS (TN[5D]). All pigs were given free access to water and 3 kg of feed twice daily (09:00 and 16:00) during the experimental period.
The values supplied per kg premix feed concentrations: Vit A, 5,000,000 IU; Vit D3, 1,000,000 IU; Vit E, 1,000 mg; Vit B1, 150 mg; Vit B2, 300 mg; Vit B12, 1,500 mg; Niacin amide, 1,500 mg; DL-calcium pantothenate, 1,000 mg; Folic acid, 200 mg; Vit H, 10 mg; Choline chloride, 2,000 mg; Mn, 3,800 mg; Zn, 1,500 mg; Fe, 4,000 mg; Cu, 500 mg; I, 250 mg; Co, 100 mg; Mg, 200 mg.
Blood samples were obtained via jugular venipuncture into heparinized vacuum tubes from all pigs at TN(-1D), HS, TN(2D), and TN(5D) before feed supplementation at 09:00. The blood samples were centrifuged within 1 h at 2,000×g for 15 min at 4°C to separate the plasma. All plasma samples were quick-frozen in liquid nitrogen and stored at −80°C for free AA determination and 1H-NMR.
FI was measured twice daily (09:00 and 16:00) during the experimental period. Water intake was measured twice daily using a water meter (Daesung, Korea), and water loss was collected in a water bottle during the experimental period. RT were taken with a digital thermometer (Microlilfe AG, Widnau, Switzerland) before the blood sample collection.
Free amino acids from the plasma were determined as described previously [18]. Aliquots of 100 μL of plasma were diluted with 100 μL of HCl (0.1 M), then deproteinized by adding 20 μL of cold (4°C) 30% sulfosalicylic acid and centrifuged at 2,500×g at 4°C for 30 min. To obtain the optimal pH for derivatization (8.2–10.0), 140 μL of deproteinized plasma was diluted with 800 μL of AccQ-Fluor borate buffer. AAs were detected by ion-exchange chromatography with post-column ninhydrin derivatization using a Hitachi L-8900 Amino Acid Analyzer (Hitachi High Technologies, Tokyo, Japan) and quantified based on authentic standards (Wako, Japan) using EZChrom Elite version 3.1.5b software.
45 µL of plasma sample was mixed with 5 µL of deuterium oxide (D2O) containing 20 mM of 3-(trimethylsilyl) propionic-2,2,3,3-d4 acid sodium salt (TSP-d4) and then transferred to NMR nanotubes. Samples were analyzed by high-resolution magic-angle spinning (HR-MAS NMR) spectroscopy. All spectra were acquired at 600.167 MHz using an Agilent NMR spectrometer equipped with a 4 mm gHX NanoProbe (Agilent Technologies, Santa Clara, CA, USA). All data were collected at a spinning rate of 2,000 Hz. Carr-Purcell-Meiboom-Gill (CPMG) pulse sequence was used to suppress the signals of water and high molecular mass compounds. The acquisition time and relaxation delay time was 3 sec. In total, 128 scans were collected and the total acquisition time was 13 min 2 sec for each sample.
For the 1H-NMR data, all spectra were processed using Chenomx NMR Suite 7.1 Professional. The Chenomx 600 MHz library database was used to identify and quantify the metabolites. TSP-d4 was used as an internal chemical shift reference (0.00 ppm) and the standard for quantification. All spectra were bucketed to 0.001 ppm from 0.6–8.6 ppm. The regions of water peak (4.5–4.9 ppm), ethanol peaks (1.14–1.2 and 3.612–3.675 ppm), and spinning sideband peaks (0.7–0.896, 1.105–1.43, and 8.1–8.25 ppm) were excluded. All samples were normalized to the total area. The normalized data were inputted into SIMCA-P+ 12.0.1 software for multivariate statistical analysis. Orthogonal partial least square discriminant analysis (OPLS-DA) was conducted using SIMCA-P+. Each spectrum was expressed as a spot on the score plot.
The statistical analyses for FI, water intake and loss, RT, free AAs, and metabolites data were performed by GLM (SAS Enterprise Guide Version 7.13 HF4, SAS Institute, Cary, NC, USA), followed by Duncan’s multiple-range test when appropriate. Variable importance in the projection (VIP) was obtained from the OPLS-DA model and VIP values > 1 were considered responsible for the differences between groups. Differences between treatment groups were considered significant when p < 0.05. Results are presented as mean and SEM.
RESULTS
ADFI, ADWD, ADWL, and RT results in growing pigs are presented in Table 2. The ADFI of the HS pigs decreased (p = 0.002) to around half as much feed as under the TN conditions (-1D, 2D, 5D). The ADWL from pig bodies under the HS condition was higher (p = 0.048) than those of TN(-1D), TN(2D), and TN(5D), but there was no treatment effect on the ADWD between HS and TN conditions. Pigs exposed to short-term HS had significantly increased RT (p = 0.0001) compared to that under the TN conditions (-1D, 2D, 5D). Compared with TN pigs, HS pigs showed FI reductions of 198%, 214%, and 214% under TN(-1D), TN(2D), and TN(5D) conditions, respectively.
Blood metabolite analyses showed that both TN conditions and short-term HS affected protein metabolism. The plasma concentrations (PC) of indispensable AAs are shown in Table 3. We found that threonine (Thr) and methionine (Met) levels were significantly higher (p = 0.011, p = 0.016) in HS to TN(5D) than TN(-1D). Valine (Val) level was also significantly higher (p = 0.044) during HS treatment than TN(-1D) and TN(2D), but the concentration under the TN(5D) condition was only slightly lower than during HS treatment. Other indispensable AAs did not show any significant differences between HS and TN conditions during the experimental period. The PC of dispensable AAs are indicated in Table 3; cysteine (Cys) and tyrosine (Tyr) were significantly higher (p = 0.046, p = 0.041) in HS to TN(5D) than TN(-1D), even though the concentrations of both AAs were slightly higher in TN(2D) than HS or TN(5D). No other dispensable AAs had significant changes in concentrations during the experimental period. The PC of some nonprotein AA metabolites are shown in Table 4. Here, the α-aminobutyric acid (α-ABA) concentration was significantly higher (p = 0.013) under the HS condition than under any TN conditions (-1D, 2D, 5D). However, β-alanine (β-Ala) concentration was significantly higher (p = 0.040) from HS to TN(5D) conditions than under TN(-1D). No other nonprotein AAs showed any significant differences between the HS and TN conditions.
Thr, threonine; Val, valine; Met, methionine; Ile, isoleucine; Leu, leucine; Phe, phenylalanine; Lys, lysine; His, histidine; Arg, arginine; Asp, aspartic acid; Ser, serine; Glu, glutamic acid; Gly, glycine; Ala, alanine; Cys, cysteine; Tyr, tyrosine; Pro, proline.
P-Ser, phosphoserine; Tau, taurine; PEA, phosphoethanolamine; Sar, sarcosine; α-AAA, α-aminoadipic acid; Cit, citrulline; α-ABA, α-aminobutyric acid; Cysthi, cystathionine; β-Ala, β-alanine; β-AiBA, β-aminoisobutyric acid; GABA, γ-aminobutyric acid; Orn, ornithine; 1-Mehis, 1-methylhistidine; 3-Mehis, 3-methylhistidine; Car, carnosine.
The mean quantitative relative concentrations of metabolites in plasma acquired by 1H-NMR from short-term HS exposure in growing pigs are presented in Table 5. Among the marker metabolites examined for comparing the effects of HS, three metabolites had significantly different levels. The level of alanine was significantly higher (p = 0.034) under TN(2D) and TN(5D) than under TN(-1D) and HS conditions. The level of creatinine was significantly elevated (p = 0.002) under the HS condition than under TN conditions (-1D, 2D, 5D). The level of leucine was significantly higher (p = 0.038) in TN(2D) and TN(5D) than TN(-1D).
HR-MAS 1H-NMR spectroscopic data were analyzed to detect changes in metabolites between plasma samples under HS and the TN conditions TN(-1D), TN(2D), and TN(5D). A two-dimensional array (2D) of the OPLS-DA data derived from the plasma samples of growing pigs did not show a separation between the treatments (Fig. 1A). However, the three-dimensional (3D) OPLS-DA plots of the plasma samples showed a clear separation between the TN and HS groups (Fig. 1B). The OPLS-DA model was also validated by 200 random permutation tests to avoid model overfitting (Fig. 1C). Thus, a total of three metabolites (alanine, creatinine, and leucine) with significantly different levels (VIP values > 1, p < 0.05) from the plasma samples of growing pigs were considered relevant for group discrimination (Table 5, Fig. 1D).
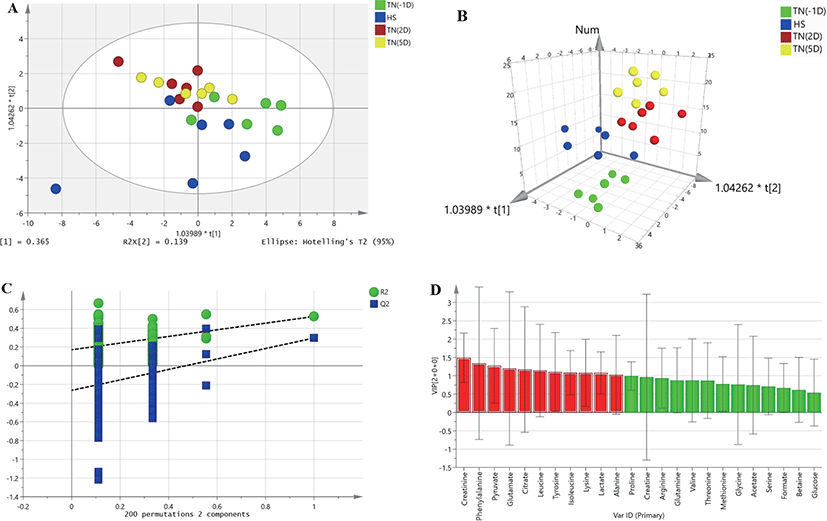
DISCUSSION
HS decreases farm animal production parameters and adversely affects the global agriculture economy [3]. Heat-induced stress leads to economic problems related to increased illness, mortality, halted growth rate, suboptimal growth, unpredictable market weights, inefficient nutrient use, poor performance, reduced carcass rate, and carcass processing problems. The influence of HS is robust at the initial stage and seems to decrease as the animal acclimates [3,12].
The two immediate effects of HS in pigs were decreased ADFI and increased RT, which may have decreased metabolic heat production and increased ADWD by 13% and ADWL from the pigs’ bodies, which in turn may have maximized heat debauchery. However, the pigs at TN(2D) and TN(5D) showed a gradual increase in ADFI and a decrease in RT, ADWD, and ADWL. HS in pigs led to reduced FI in the present study and previous studies [19,20]. According to Xin and Harmon [21], recorded ambient temperatures and relative humidity suggested that pigs housed in a HS room were under natural HS for at least 12 h daily. On average, the HS pigs showed a 1.2°C higher average body temperature (BT) and two-fold higher respiratory frequency than did the TN pigs [22]. Therefore, high BTs and respiratory rates are indicators of HS in pigs [9,23]. For this reason, short-term HS pigs in our study showed a sudden drop in voluntary FI, but the FI of TN(2D) to TN(5D) pigs gradually increased because they were recovering from the HS. However, HS pigs drank much more water compared to TN pigs. This is because HS pigs increase their BT by evaporative body cooling. According to Peng et al. [24], HS pigs increase their water drinking behavior because they are uncomfortable and trying to decrease their BT. In the current study, we found an RT of ~41°C in short-term HS pigs, similar to Pearce et al. [12], who also found a high RT under 35°C HS condition pigs. Earlier studies have revealed that nutrient limitations are not the only drivers of altered metabolism during HS, as heat stresses directly affect pig metabolism [12]. Therefore, we exposed pigs to HS for 1 day and supplemented them with feed, maintained them under TN conditions with the same diet for the remaining time, and elucidated the effects of HS on metabolism. This model used a metabolic approach to determine the mechanisms essential for pig responses to HS.
In the current study, the PC of indispensable AAs (e.g., Thr, Val, and Met) were affected and showed higher concentrations in HS pigs than those in the TN(-1D), TN(2D), and TN(5D) conditions. However, in contrast to the results of our study, a previous study found that Thr and Val concentrations decreased under HS conditions [25]. We believe that this is because the HS condition, duration of exposure, diet condition, and age of the pigs might have played a major role in these differences. Comparison of the metabolites of the HS and TN condition groups showed that HS altered the levels of AAs, some of which are involved in AA-metabolizing pathways, including serine and Thr metabolism. Pearce et al. [23] found that acute HS considerably increases mucin-2 secretion in pigs. Generally, mucins are glycoproteins rich in Thr, Pro, and Ser [26]. Morales et al. [25] found a higher recovery of endogenous Thr at the terminal ileum of HS pigs compared to TN ones, thus indicating that the former had increased mucin secretion.
Valine is a branched-chain AA; valine catabolism begins with the elimination of the amino group by transamination, giving alpha-ketoisovalerate, an alpha-keto acid, which is then transformed to isobutyryl-CoA through oxidative decarboxylation by the branched-chain α-ketoacid dehydrogenase complex [27]. Similar to the present study, Xu et al. [28] observed that the valine concentration was increased in Apostichopus japonicus during short-term acute HS. We argue that valine is a potential marker for short-term HS. The role of valine in HS is very limited in pigs, and therefore further studies are needed on how it works in HS animals.
Met is generally involved in the cellular antioxidant mechanism, and Met donates a methyl group during creatine synthesis; creatine seems to consume more Met methyl groups than all the remaining methylation reactions combined [29]. Besides, based on Luo and Levine [30], free and protein-bound Met functions as an antioxidant by defending other proteins and macromolecules. Bender et al. [31] found that Met is rich on the surface of mitochondrial and nucleic acid binding proteins that show high oxidant fluxes. Similarly, in the previous study, the postabsorptive serum concentration of Met was higher in HS than in TN pigs [25]. Thus, the antioxidant function of Met may be higher based on the Met PC of short-term HS pigs than that of TN(-1D) ones.
In the present study, the PC of the dispensable AAs Cys and Tyr were affected and remained significantly increased during recovery time than TN(-1D) group. However, Cervantes et al. [32] found that the serum concentration of Cys decreased by 38% in HS-growing pigs. Cys reduction might be associated with the antioxidant role of this AA as a component of glutathione, as Cys, Glu, and Gly are the three components of glutathione. ROS production increases in cells under HS, and thus the need for antioxidants may also increase [33]. In the current study, we assume that HS conditions may increase the use of Cys for glutathione synthesis, a key intracellular antioxidant, mainly in HS situations. Cervantes et al. [32] also found that the serum concentration of the dispensable AA Tyr was affected and significantly decreased in HS-exposed growing pigs. This response may also suggest that cells remove these dispensable AAs from the blood to synthesize body proteins and functional proteins that counteract the effects of HS. However, the PC of Cys and Tyr were remained increased during recovery time, which means that absorptive alteration occurred.
The PC of nonprotein AAs such as α-ABA and β-Ala are significantly higher in HS pigs than in TN(-1D), TN(2D), and TN(5D) pigs. The production of α-ABA is a response to various environmental stresses in plants, including environmental HS; however, to date, there has been no convincing evidence that it responds to HS in animals. We hypothesized that these AAs may play a major role in protecting under HS conditions. Our PC data specified that HS increases the concentrations of β-Ala. In contrast, primiparous sows showed significantly lower β-Ala levels under HS (28°C–32°C) than TN [34]. β-Ala metabolites can be diverted for pantothenic acid and coenzyme A (CoA) biosynthesis, and impact acetyl-CoA levels. This consequence is also supported by the fact that metabolic pathway enhancement analysis revealed that β-Ala metabolism and pantothenate and CoA biosynthesis pathways are enriched under HS. Interestingly, HS modulates β-alanine levels, which in turn regulate excitotoxicity responses and prevent neuronal cell death. We believe that the protective role of β-alanine may include cellular preservation of enzyme structure and function, indicating that β-alanine may act as a chemical chaperone.
In this study, considerable changes in alanine, creatinine, and leucine metabolite concentrations were found in growing pigs exposed to short-term HS as shown in Fig. 2. Alanine is an α-AA used in protein biosynthesis [35]. In mammals, alanine plays an important role in the glucose-alanine cycle between tissues and the liver [35]. This cycle plays an important role in animal physiology and permits pyruvate and glutamate to be removed from the muscles and be safely transported to the liver [35]. This is also a prominent method for eliminating toxic ammonium ions from the muscle tissue, and also indirectly supplying glucose to energy-deprived muscle tissue [36]. Results of the current study suggest that HS affects the glucose–alanine cycle, thus increasing the alanine concentration in the plasma during recovery time.
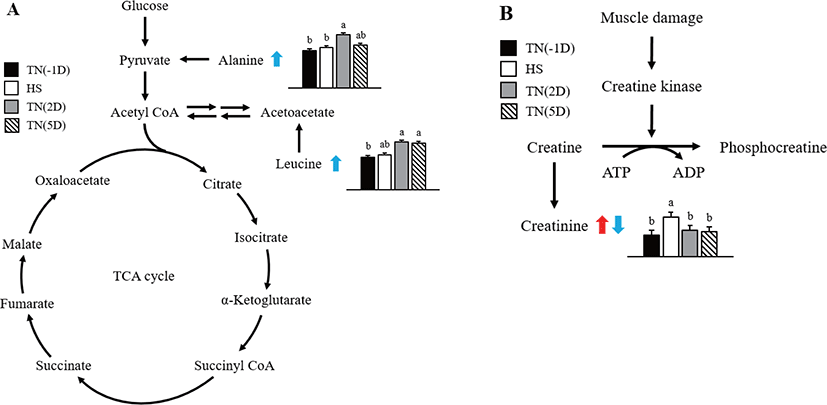
The relative percent change in creatinine was higher under HS than under TN(2D) and TN(5D) conditions. According to earlier studies, creatinine in the HS group leads to phosphocreatine in the muscle tissue being used for energy in the animal [37,38], a finding that is in line with the up-regulation of creatinine amidohydrolase. In general, proline, glycine, isoleucine, threonine, and arginine concentrations were higher in the HS group. According to Li et al. [39], the above compounds may be indicators for glucose production through gluconeogenesis or energy via deamination and oxidation. The improved mobilization of AAs also augmented the formation of urea in the HS group.
Leucine is an essential AA that is used in protein biosynthesis. In this study, the concentration of leucine metabolite in plasma was significantly increased under recovery. Similarly, Morales et al. [25] found that leucine concentration was lower in the serum of HS growing pigs than untreated ones. The reduction in leucine concentration in HS pigs suggests that HS also decreases the activity or abundance of the neutral AA transporter B0 [40]. Leucine concentration in the plasma was also found to be reduced under HS in chicks [41]. Leucine has higher concentrations in the adipose and muscle tissues and tissues that use leucine to form sterols and other compounds. We assume that protein synthesis was disturbed by HS, and subsequently, the leucine concentration might have also increased in the plasma of pigs under recovery.
OPLS-DA plots of the metabolomic data showed a clear separation between the HS and TN(-1D) groups, but the HS group overlapped with some of the TN(2D) and TN(5D) condition groups, indicating that the metabolites had not fully recovered from the HS conditions. As expected, the ADFI was considerably lower and ADWL was higher in HS than the TN conditioned pigs. The PC of some indispensable and dispensable AAs were higher in HS, TN(2D), and TN(5D) than in TN(-1D) pigs. In HS pigs, the PC of indispensable AAs such as Thr, Val, and Met were higher than in TN(-1D) pigs. At the same time, the PC of dispensable AAs such as Cys and Tyr were also higher in HS, TN(2D), and TN(5D) than TN(-1D) pigs. These variances might be a carryover effect of the differential AA absorption among HS, TN(2D), TN(5D), and TN(-1D) pigs. It is possible, however, that cells of TN pigs primarily removed AAs from circulation to retain body growth, while HS pigs lost AAs to restore their body and protect themselves from HS.
In conclusion, HS significantly decreases the FI and increases the BT in growing pigs. The physiological and metabolic responses of pigs to HS conditions are extremely difficult to explain. The PC of AA and some of their metabolites give new information on HS and its influence on TN(2D) and TN(5D) conditions. Overall, the metabolites identified in this study vary significantly among TN(-1D) samples, HS samples, and TN(2D) and TN(5D) samples, and HS conditions have apparent impacts on the metabolite levels during the recovery period (TN[2D] and TN[5D]). Caloric restriction by decreased voluntary FI is to minimize heat production and post-absorptive metabolic changes by adaptive mechanism were inevitable to maintain their body from HS [42]. Therefore, the PC of AA and some of their metabolites showed that pigs under HS conditions are forced to alter their metabolism in some indispensable and dispensable AAs, especially Thr, Val, Met, Cys, and Tyr. These results provided information to understand the mechanisms underlying acute HS responses over time. However, further studies are required to better elucidate the role that these metabolites and their metabolic pathways play in HS adaptation and whether they could be developed to track the adaptive responses of growing pigs to short-term HS.