INTRODUCTION
The concept of probiotics is widely known as “live micro-organisms that confer a health benefit on the host when administered in adequate amounts” [1]. Probiotics are known to increase growth rate and nutrient utilization and to change immunity when it is used in pig diets [2–6]. However, when probiotics are added to animal feeds, there can be side effects, such as toxicity in the host caused by production of enterotoxins from micro-organisms included in the probiotics [5,7] and a risk of infection due to strong adhesion to the intestinal tract by probiotic micro-organism [8]. In addition, there is a concern to maintain the activity of probiotics and thus stability of probiotics is required in term of viability and metabolic and functional activities during shelf life [9].
Current research suggests that inactivated probiotics can have the same beneficial effects as probiotics [10–12]. Inactivated probiotics not only benefit the host by regulating immunity, suppressing pathogens through the increased intestine adhesion, and providing metabolites secreted from dead cells but also offer easier storage, longer product shelf life, and more convenient transportation [13,14]. Inactivated probiotics can also complement the risk and stability of probiotics [15]. Inactivated probiotics can be obtained by heat treatment methods and thus it is generally called heat-killed probiotics. Heat-killed probiotics can release components such as lipoteichoic acids, peptidoglycan, or exopolysaccharides (EPS) with immuno-regulating effects against pathogens [16].
Lactobacillus rhamnosus (LR) has been shown to increase growth rate of pre-weaning pigs, mitigate diarrhea incidence, and regulate immunity [17,18]. However, previous study reported LR isolated from humans reduced growth performance, increased diarrhea scores, and reduced immunoglobulins of the Escherichiacoli challenged pigs [19]. On the other hand, previous studies showed heat-killed LR improved growth rate, feed application, and immunity and reduced stress of fish [20,21]. In addition, heat-killed LR can act as an immune regulator by reducing lipopolysaccharide-induced pro-inflammatory cytokines in the human placenta and increasing anti-inflammatory cytokines in infant rats [22,23]. However, there are limited information about dietary heat-killed LR in animal diets.
Therefore, the objective of present study was to evaluate dietary effects of heat-killed LR on growth performance, nutrient digestibility, and immune responses of weaned pigs.
MATERIALS AND METHODS
All experimental procedures were reviewed and approved by the Institutional Animal Care and Use Committee of Chungnam National University, Daejeon, Korea (approval code: CNU-00832).
The LR stains in the study were isolated from pigs and cultured in MRS (DeMan-Rogosa-Sharpe) broth (BD DifcoTM, Franklin Lakes, NJ, USA) for 18 hours at 37°C in an anaerobic incubator before use. Heat-killed LR was processed by heating at 80°C for 30 min. The stain is a white flowable powder which contains 1 × 109 FU/g of EPS-cell particle (CJ CheilJedang Biotechnology Research Institute, Seoul, Korea).
Ninety-six weaned pigs ([Landrace × Yorkshire] × Duroc; 6.95 ± 0.25 kg body weight [BW]; 28 d old) were randomly allotted to four treatments: 1) a basal diet without heat-killed LR (CON), 2) T1 (CON with 0.1% heat-killed LR), 3) T2 (CON with 0.2% heat-killed LR), and 4) T3 (CON with 0.4% heat-killed LR). Each treatment had six pens with four pigs (2 barrows and 2 gilts; 6 replicates per treatment) in a randomized completely block design (blocks: BW and sex). The different inclusion levels of heat-killed LR were deiced based on the results from previous pilot studies and producer’s recommendations. All diets were formulated to meet and exceed the nutrient requirements for weaned pigs [24] and were provided in a two-phase feeding program with each phase lasting two weeks (Table 1). All pigs had ad libitum access to feed and water.
Provide per kilogram of diet: vitamin A, 12,000 IU; vitamin D3, 2,500 IU; vitamin E, 30 IU; vitamin K3, 3 mg; D-pantothenic acid, 15 mg; nicotinic acid, 40 mg; choline, 400 mg; and vitamin B12, 12 μg, Fe, 90 mg from iron sulfate; Cu, 8.8 mg from copper sulfate; Zn, 100 mg from zinc oxide; Mn, 54 mg from manganese oxide; I, 0.35 mg from potassium iodide; Se, 0.30 mg from sodium selenite.
Growth performance (average daily gain [ADG], average daily feed intake [ADFI], and feed efficiency [gain to feed ratio, G:F]) was calculated by measuring the weight of individual pigs and pen feeders on day 1, 14, and 28. Daily visual diarrhea scores of individual pigs (1 = hard and dry normal feces, 2 = well formed, 3 = moist feces, 4 = diarrhea, 5 = watery diarrhea) were recorded for 2 weeks after weaning. The frequency of diarrhea was calculated by counting pens per day with an average diarrhea score over 4 in each pen [25,26]. On day 1, 3, 7, and 14 of the experimental period, one pig was randomly chosen from each pen for blood collection. Whole blood and serum were collected from the jugular vein of pigs using EDTA and non-EDTA tubes, respectively (10 mL; BD Vacutainer Systems, Franklin Lakes, NJ, USA). The blood was kept at room temperature to coagulate and centrifuged to separate the serum at 3,000×g at 4°C for 15 min and stored at −20°C. Dietary treatments contained 0.2% chromic oxide as an indigestible marker for the last week of the experimental period. Fecal samples were collected by rectal palpation from randomly chosen one pig in each pen for the last 3–4 days of experiment after the 4 adaptation days and immediately stored at −20°C.
Feed and fecal samples were dried in a drying oven for 72 h at 65°C and ground with a cyclone mill (1.0 mm screen; Foss Tecator cyclotec 1093, Foss, Hillerød, Denmark). Samples were analyzed for dry matter (DM), and crude protein (CP; N × 6.25) following the method of the AOAC [27]. Gross energy (GE) was analyzed by automatic bomb calorimeter (PARR 1261 Bomb Calorimeter, PARR Instrument, Moline, IL, USA). Chromium content was determined by the absorption spectrophotometer (Hitachi Z-5000 Absorption Spectrophotometer, Hitachi High-Tech, Tokyo, Japan) based on the method of Williams et al. [28]. Nutrient digestibility (apparent total tract digestibility [ATTD]) was calculated according to the index method formula [29].
The number of white blood cell (WBC) was determined by an eight-parameter hematology analyzer (Scil vet abcTM Animal Blood Counter, Scil Animal Care Company, Altorf, France). Immune responses such as serum tumor necrosis factor-α (TNF-α), transforming growth factor-β1 (TGF-β1), C-reactive protein (CRP), and cortisol were measured using ELISA kits according to the manufacturer’s protocol (TNF-α, TGF-β1, and CRP [Genorise Scientific, Berwyn, PA, USA]; cortisol [Cusabio Biotech, Wuhan, China]).
Data were subjected using the PROC MIXED procedure of SAS version 9.4 (SAS Institute, Cary, NC, USA) in a randomized completely block design (blocks: BW and sex). Experimental unit was the pen. Statistical model for growth performance, ATTD of nutrients, immune responses included dietary treatment as a fixed effect and blocks as random effects. Contrasts were performed to verify linear and quadratic effects of heat-killed LR. Frequency of diarrhea was analyzed by the chi-square test. Data were given as least square mean ± SEM. Values of p < 0.05 and 0.05 ≤ p ≤ 0.10 were considered as statistical significance and tendency, respectively.
RESULTS AND DISCUSSION
During the first two weeks of this study, the heat-killed LR group increased growth rate (ADG; p < 0.05) and feed efficiency (G:F; p ≤ 0.10) of weaned pigs compared with CON (Table 2). In addition, there were linear improvements (p < 0.05) of growth rate and feed efficiency of weaned pigs as the addition levels of heat-killed LR were increased in the diet (Table 2). Growth rate (ADG; p < 0.05) and feed efficiency (G:F; p < 0.05) of weaned pigs were increased in the heat-killed LR group for overall experimental period compared with those in CON (Table 2). Moreover, growth rate and feed efficiency of weaned pigs during overall experimental period were linearly improved (p < 0.05) when the inclusion levels of heat-killed LR were increased in the diet (Table 2). The heat-killed LR group decreased (p < 0.05) post-weaning diarrhea of weaned pigs compared with CON (Fig. 1). Previous studies also showed live and heat-killed Lactobacillus strains increased weight gain in pigs [30–32], mice [33], and fish [34] and mitigated diarrhea in human and animals [18,35,36]. When pigs are infected by enterotoxigenic E. coli (ETEC) after weaning, it increases cell fluid and electrolytes into the lumen and decreases absorbing them into intestinal cells by ETEC, leading post-weaning diarrhea [37]. In addition, the ETEC infection increases the paracellular permeability and further the penetration of pathogens and toxins into the lumen, thereby increasing the inflammatory immune responses and reducing the growth of animals [37]. The results from present study may be due to the mechanism of live and inactivated probiotics that prevent adherence of pathogens to the intestinal barrier and regulate the immune system of animals [14,16,32,38].
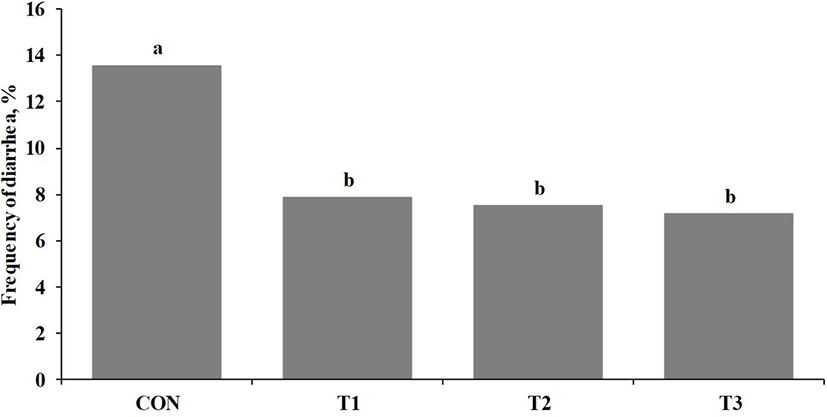
The ATTD of GE of weaned pigs was higher (p < 0.05) in the heat-killed LR group than that in CON (Table 3). There was also a linear improvement (p < 0.05) of ATTD of GE of weaned pigs related to the increased inclusion concentrations of heat-killed LR in the diet (Table 3). However, no differences were found on ATTD of DM and CP of weaned pigs among dietary treatments (Table 3). These results are similar to the results from previous studies using live probiotics [39,40]. The EPS produced by Lactobacillus strains exists for a long time in the intestine and promotes colonization of Lactobacillus in the intestinal tract, thereby alleviating lactose intolerance, modulation of immunity against pathogens, and reducing mutagenic enzymes such as β-glucuronidase, nitroreductase and chologlycine hydrolase [41]. In addition, Lactobacillus strains increased nutrient utilization by enzymes such as β-galactosidase that are potentially activated and helps nutrient digestion [40].
Serum TNF-α of weaned pigs was decreased in the heat-killed LR group (d 7; p < 0.05) compared with that in CON (Table 4). In addition, there was a significant linear decrease (p < 0.05) in serum TNF-α of weaned pigs as the inclusion levels of heat-killed LR were increased in the diet (Table 4). The heat-killed LR decreased serum TGF-β1 (d 7; p ≤ 0.10) and cortisol (d 3 and 7; p < 0.05) of weaned pigs compared with CON (TTable 4). There was also a significant linear decrease (d 7; p ≤ 0.05) in serum cortisol of weaned pigs when the inclusion levels of heat-killed LR were increased in the diet (Table 4). However, there were no significant differences on the number of WBC and serum CRP among dietary treatments (Table 4). Previous studies reported the live and heat-killed Lactobacillus stains decreased TNF-α [22,42,43], TGF-β1 [44,45], and cortisol [46,47] in human and mice. Cortisol is a substance released in responses to acute stress. Generally, weaned pigs can face social, physical, and physiological stresses and contribute to increasing intestinal permeability and depressing immune responses [48]. It is known that live LR decreases cortisol by secreting neurotransmitters [48] and that the EPS produced by Lactobacillus strains shields adhesive surface polymers to prevent pathogens from sticking to intestinal barrier and regulates immune responses to inhibit the releases of pro-inflammatory cytokines. [43,49,50]. The CRP is the first acute-phase protein that can be used as an inflammatory response indicator and pro-inflammatory cytokines such as TNF-α and TGF-β1 are important inducers of CRP synthesis [51]. In present study, the CRP of weaned pigs was no significant difference among dietary treatments, but the concentration of CRP was decreased during the experimental period. Therefore, heat-killed LR may be thought to provide anti-inflammatory effects in the immune system [44,45].
CONCLUSION
Dietary heat-killed LR improved growth rate, modified immune responses of weaned pigs, and alleviated post-weaning diarrhea. Supplementation of dietary heat-killed LR in weaner diets may reduce post-weaning diarrhea by protecting the intestinal barrier from pathogens and attenuating pro-inflammatory immune responses and post-weaning stress.