INTRODUCTION
Edible insects are expected to supplement and possibly replace traditional meat proteins in many parts of the world. Some studies have assessed the use of edible insects as a source of protein [1–3]. Edible insect consumption could not only reduce the amount of ozone produced by traditional meat production, thus reducing environmental pollution [4], but the production of protein using edible insects is less costly than traditional meat production and far more rapid due to the short breeding period of insects [5]. More than 1,900 species of edible insects are consumed worldwide [6] and edible insects are an important resource for future food security [2,3]. However, edible insects are being ignored by consumers because of their appearance, among other perceptions [7,8]. Therefore, edible insects cannot be used intact, and instead, the proteins from these insects are extracted, dried, and pulverized [4]. Edible insects have a high protein content, but processing them for consumption can be challenging due to the high levels of chitin present in their bodies [9]. Adult edible insects contain much larger amounts of chitin than their larvae; therefore, larvae may present a more suitable insect-based protein source [10,11].
The yellow mealworm (Tenebrio molitor) is a member of the Coleoptera family and is cultivated in Korea and worldwide [6]. T. molitor is mostly used as animal feed or as a protein supplement because it is rich in protein and essential fatty acids. The white-spotted flower chafer (Protaetia brevitarsis) is a carnivorous insect belonging to the Cetoniidae family that undergoes complete transformation from the eggs, larvae, and pupa, to the imago [12]. The Korean rhinoceros beetle (Allomyrina dichotoma), belonging to the Scarabaeidae family, is known to inhabit Korea, Japan, China, and Taiwan. A nutritional analysis of the larvae of these beetles found that they contained high levels of essential unsaturated fatty acids [13].
Among the various types of processed foods, emulsion-type sausages are a popular dish worldwide [14]. Therefore, the development of emulsion-type sausages formulated with edible insects is recommended. However, almost no studies have assessed the manufacture of meat products using edible insect emulsions, and studies have mainly focused on the use of simple protein substitutes.
The aim of this study was to evaluate the physicochemical characteristics of emulsions from T. molitor, A. dichotoma, and P. brevitarsis seulensis, to understand their potential as protein sources and meat substitutes.
MATERIALS AND METHODS
The larvae of T. molitor (moisture, 63.18 ± 1.04%; protein, 21.53 ± 1.53%; fat, 9.33 ± 0.31%; ash, 1.65 ± 0.11%), P. brevitarsis seulensis (moisture, 79.73 ± 0.59%; protein, 10.35 ± 0.35%; fat, 4.29 ± 0.09%; ash, 1.16 ± 0.02%), and A. dichotoma (moisture, 88.06 ± 0.71%; protein, 2.93 ± 0.16%; fat, 2.93 ± 0.16%; ash, 0.40 ± 0.01%) were purchased from a farm (Jeongeup, Korea), and pork back fat was purchased from a local processor. The larvae and pork back fat were ground (6 mm) with a grinder (PA-82, Mainca, Barcelona, Spain). Three different emulsions were produced: 65% T. molitor, 20% pork back fat, and 15% ice; 65% A. dichotoma, 20% pork back fat, and 15% ice; and 65% P. brevitarsis seulensis, 20% pork back fat, and 15% ice. The ground larvae were homogenized for 2 min using a silent cutter (Cutter Nr-963009, Scharfen, Witten, Germany). Ice water (1°C), 1.5% NaCl, and 0.15% phosphate were added to the ground larvae and mixed for 90 s. Pork back fat was added after 2 min, and the emulsions were homogenized for 2.5 min at temperatures below 10°C. The emulsions were stuffed into a collagen casing (diameter 24 mm) using a stuffer (IS-8, Sirman, Padua, Italy). These formulations were analyzed in triplicate for each emulsion type [14].
The pH of the emulsions was determined in a homogenate prepared from samples (5 g) and distilled water (20 mL) using an electronic pH meter (Model 340, Mettler-Toledo GmbH, Schwerzenbach, Switzerland).
The color of the emulsions was measured with a CR-410 Chroma Meter (Minolta, Osaka, Japan) using the CIE color system, and was calibrated using a standard white plate [CIE L* = +97.83, CIE a* = −0.43, CIE b* = +1.98].
Before analysis, the emulsions were cooked at 75°C for 30 min and cooled at 25°C for 2 h. The TPA was conducted using a texture analyzer (TA-XT2i, Stable Micro Systems, Godalming, UK). The TPA conditions were as follows: maximum load, 1 kg; pre-test speed, 1.0 mm/s; post-test speed, 1.0 mm/s; head speed, 1.0 mm/s; distance, 5.0 mm; and force, 1.0 g [15].
The molecular weight distribution of proteins in the insect-based emulsions was estimated using SDS-PAGE. Chemicals used in this experiment were obtained from Bio-Rad Laboratories (Hercules, CA, USA). After a 10-fold dilution, 20 μL of each sample and 4× sample buffer were mixed well and heated to 100°C for 5 min [16]. Cooled samples were loaded on a 12% Mini-PROTEIN® TGX at 80 mA with a standard marker (Plus Protein TM Dual Color Standards) and the gel was stained with Coomassie brilliant blue R-250.
The apparent viscosity of the emulsions was determined with a Brookfield viscometer (DV3T HB, SC4-29 standard cylinder sensor; Brookfield Engineering Labs, Stoughton, MA, USA). A metal cup filled with emulsion (5 mL) at 17°C was rotated under a continuous shear rate for 35 s [17].
A DSC 4000 was used to assess the thermal stability of emulsion samples (DSC 4000, PerkinElmer, Waltham, MA, USA). An empty pan was placed on one side of the sensor and a sealed pan containing 30 μg of sample was placed on the other side. A temperature range of 20°C–120°C was used and the heating rate was maintained at 10°C/min.
SPSS Statistics (SPSS, Chicago, IL, USA) was used to analyze the data. The fixed effects were considered when comparing differences among insect species. A one-way ANOVA test was performed with a Duncan’s multiple range comparison test (p < 0.05). All experiments were conducted in triplicate, and emulsions were separately manufactured for each replicate.
RESULTS AND DISCUSSION
The pH and color of the emulsions prepared from the edible insect larvae are presented in Table 1. The A. dichotoma emulsion had the highest pH and the T. molitor emulsion had the lowest (p < 0.05). These results are in accordance with those of the study by Kim et al. [8], who observed similar technical functional properties of extracts from edible insects. Kim et al. [18] concluded that the pH of edible insect protein can significantly improve the quality characteristics of the emulsion-type sausages. Mishyna et al. [11] indicated that a higher pH enhanced the quality of edible insect protein and protein functionality.
The color of the different emulsions is shown in Table 1. The A. dichotoma emulsion had the lowest lightness, redness, and yellowness values (p < 0.05), while the T. molitor emulsion had the highest values (p < 0.05). Similar results were reported by Kim et al. [8], who noted significant differences in the lightness, redness, and yellowness values of proteins extracted from edible insects. Mishyna et al. [11] and Wittkopp and Beldade [19] reported that the color of edible insects was influenced by the melanin content. In this study, the color of emulsions also appears to have been influenced by the melanin content of the insect species.
The effects of the insect species on the TPA of the emulsions are presented in Table 2. The T. molitor emulsion had higher hardness, gumminess, and chewiness values (p < 0.05) than the P. brevitarsis seulensis and A. dichotoma emulsions. The P. brevitarsis seulensis and A. dichotoma emulsions had higher springiness and cohesiveness values (p < 0.05) than the T. molitor emulsions. Of the three different larval emulsions, T. molitor showed the poorest protein functionality, which could be due to the poor protein-protein interaction networks among the proteins within this emulsion [9]. This poor interaction induces a low level of water holding capacity and increases the loss of moisture, which leads to an increase in the hardness and a decrease in the springiness and cohesiveness of the emulsions [17]. Kim et al. [2] observed that the heat-induced gel prepared with A. dichotoma was harder than that of the gel prepared with the other treatments, and that all emulsion sausages prepared with edible insects were harder than the control sausages without insects. In this study, T. molitor produced a rigid emulsion and the other species produced more flexible emulsions. These results confirmed that cooked insect emulsions differ in textural properties according to the insect species used. Therefore, to effectively replace meat products with edible insects, it is important that the textural properties of the emulsions are maintained as close to that of traditional meat products.
SDS-PAGE can be used to estimate protein distribution based on molecular weight [16]. High-molecular-weight proteins and hydrophobic groups may have stronger network systems [20]. In general, staining agents bind to the hydrophobic sites of proteins, and thus, staining agents can be used to reveal protein molecules [16]. The sample prepared using T. molitor was shown to produce the thickest bands (Fig. 1), followed by that prepared using P. brevitarsis seulensis. Although the P. brevitarsis seulensis emulsion samples had thicker bands compared to the A. dichotoma emulsion samples, a significant difference was not observed in the TPA (Table 2). The proteins extracted from T. molitor had a lower molecular weight than those from the other species, which may be attributed to the technical properties of the proteins [9]. A trend was observed whereby the bigger the size of the insect, the fainter the bands in the SDS-PAGE analysis; however, these differences might be species-specific. Ground edible insect products comprise various components, such as hemolymph, enzyme, muscular protein, and chitin [9]. Furthermore, different body sizes, molecular components, and protein concentrations might affect the molecular distribution of proteins. For example, a high protein concentration could induce an increase in the molecular weight and protein distribution. Of the emulsions prepared from the three insect species evaluated in the present study, the T. molitor emulsion was found to have the best gelling properties.
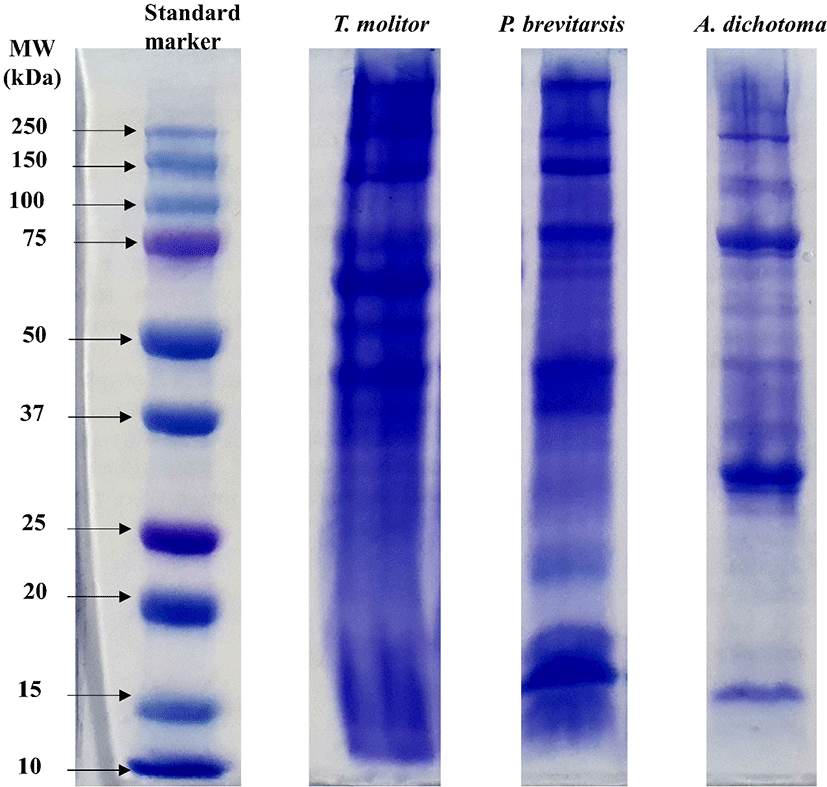
The apparent viscosity of the emulsions differed significantly among species (Fig. 2). The apparent viscosity of the T. molitor emulsion was higher than that of the other treatments. Kim et al. [2] reported that the apparent viscosity of a P. brevitarsis seulensis gel was higher than that of gels prepared using other treatments. Our results differed due to the correlation between protein and fat in the formulated emulsions. Choi et al. [5] reported that water and oil absorption capacities differ depending on the insect species used, and that this also affects the apparent viscosity, emulsifying capacity, and foaming ability of the emulsions. Therefore, T. molitor, the emulsion of which was shown to have the highest apparent viscosity in the present study, might be more suitable for use in the manufacture of insect based-emulsions compared to other species.
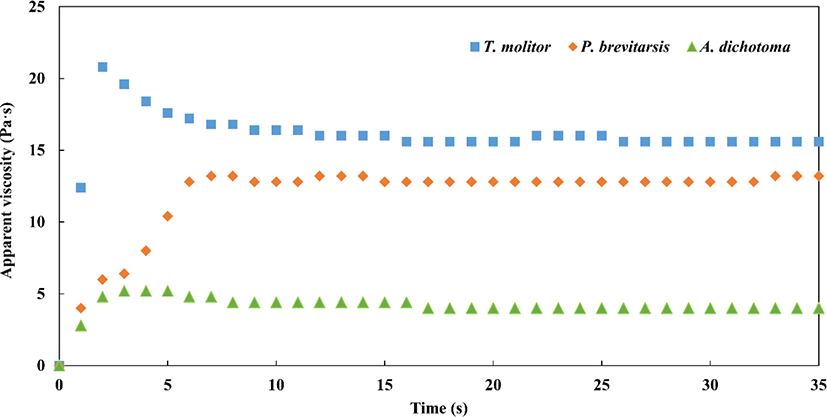
The thermal properties of emulsions are important indicators of the thermal stability of proteins [21]. The thermal properties of the insect-based emulsions are shown in Fig. 3. Protein denaturation was observed at temperatures ranging from 60 to 100°C. The distributions for T. molitor and A. dichotoma showed one peak each (at ~80°C and ~72°C, respectively) and that of P. brevitarsis seulensis showed two peaks (at ~80°C and ~72°C). These differences may be attributable to differences in the thermal stability of the proteins that the larvae of these species comprise. Proteins that denature at ~80°C may have a more critical impact on thermal-induced gelling properties than those that denature at ~72°C, as demonstrated by T. molitor showing the highest gelling properties (Table 2).
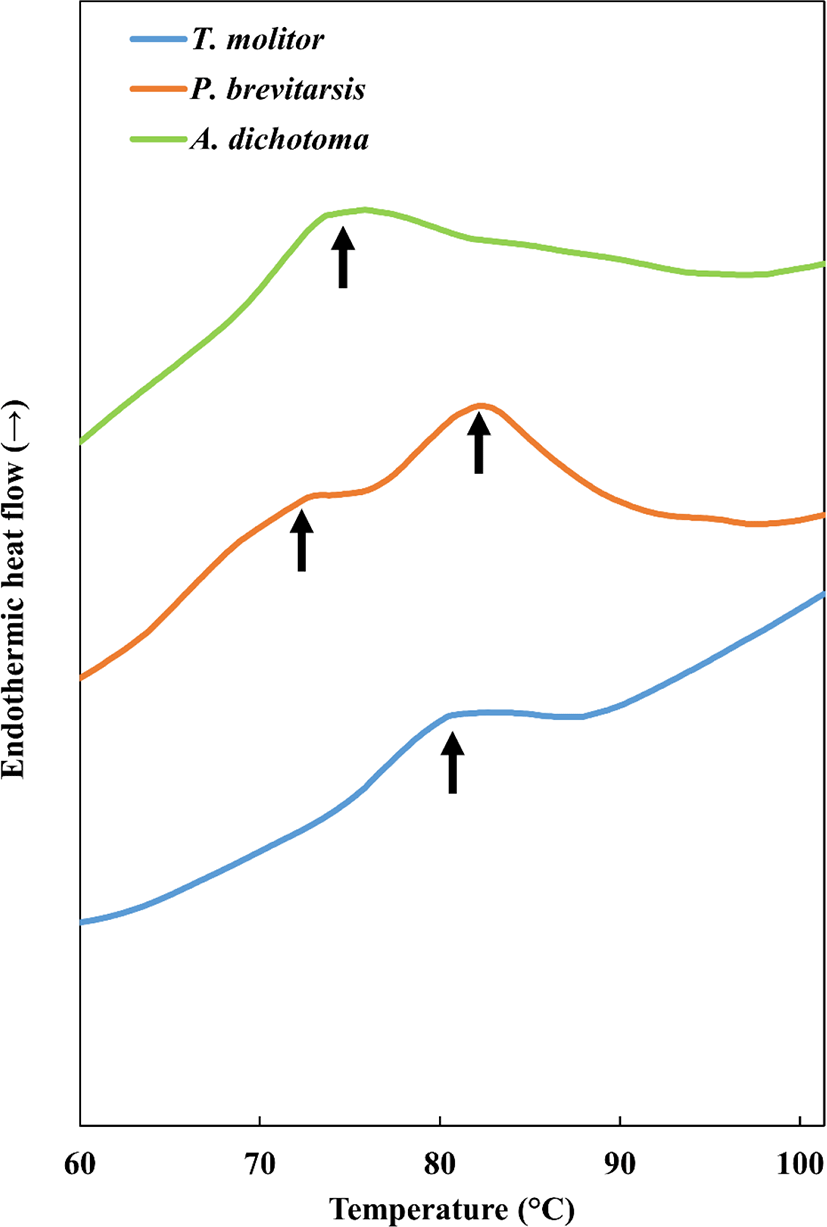
CONCLUSION
The physicochemical characteristics and rheological properties of emulsions prepared with various edible insect larvae were investigated. Collectively, our findings suggest that emulsions from T. molitor larvae may be a suitable meat-replacement candidate; specifically, in terms of the texture profile, apparent viscosity, SDS-PAGE results, and DSC findings. Here, we not only confirmed the potential of edible insects as meat protein replacements, but we also provided a technical foundation for the manufacture of various meat products, specifically emulsion-type foods, from edible insects.