INTRODUCTION
Lipopolysaccharide (LPS) is a major cell wall component of Gram-negative bacteria and is a physiologically well-known endotoxin derived from them, and one of the representative pathogen associated molecular patterns (PAMPs) [1–3]. LPS infection has been associated with increased cloacal temperature, depression, lethargy, diarrhea, avoidance of feed, hypercholesterolemia, hypouricemia and hyperphosphatemia occurred in broiler chickens [4,5]. It also reduced leukocyte and thrombocyte counts and induced leukocytosis in piglets [6]. It further contributed to a diverse range of mild and severe inflammatory diseases in human and animals such as ulcerative colitis [7], acute pneumonia [8] and systemic sepsis [9]. Surprisingly, in animal husbandry, swine farms and poultry houses are vulnerable to LPS contamination [1,5], especially detected as a dust suspension at higher concentrations of 4.9 μg/m3 at the former [1].
Structurally, the LPS carries phosphorylated lipid A moiety, which accounts for the toxicity and immunostimulatory effects of the intact molecule [6]. Therefore, the degradation of LPS by phosphatase activity disrupts its function [6,10,11]. Phytase is a specific type of phosphatases exclusively hydrolyzing phytate that exists as primary phosphorus reserve in edible plants such as cereals, legumes and oilseeds and acts as an anti-nutritional factor to non-ruminants [12]. Phytase is a well-established and commercially available feed additive enhancing phosphate bioavailability in animals, and reducing phosphate pollution in the environment [12]. Intriguingly, the function of wheat phytase that is classified as a unique multiple inositol polyphosphate phosphatase (MINPP) is attractive due to its non-specific phosphatase activity against other phosphorylated substrates such as p-nitrophenyl phosphate and 2,3-bisphosphoglycerate, a negative allosteric regulator of hemoglobin, even if it is much less known than microbial counterpart [13,14].
Therefore, we hypothesized that wheat phytase can dephosphorylate LPS. Until now, little has been reported about the hydrolysis of LPS by phytases. The objective of this study was to characterize the enzymatic hydrolysis of LPS by wheat phytase and to investigate the effects of the wheat phytase-treated LPS on in vitro toxicity, cell viability and the release of a pro-inflammatory cytokine, interleukin (IL)-8 from target cells when compared with the intact LPS.
MATERIALS AND METHODS
Wheat phytase (Sigma-Aldrich, Saint Louis, MO, USA) was reconstituted in endotoxin-free water (Sigma-Aldrich). The phosphatase activity of the enzyme (28.6 mU/mL) against the substrate, LPS (100 μg/mL) was determined in acetate buffer (pH 5.0) at 37°C for the given duration (15 min or 1 h) in the presence or absence of inhibitors (10 mM L-phenylalanine or L-homoarginine). In addition, LPS (100 μg/mL) was treated with different units of wheat phytase (14.3 and 57.2 mU/mL) in acetate buffer (pH 5.0) at 37°C for 1 h and the phosphatase activity of the enzyme against LPS was assayed with different concentrations (5 and 20 mM) of the inhibitors. The inorganic phosphate release was measured at optical density (OD) 635 nm using the malachite green-based PiColor Lock gold phosphate detection kit (Innova Biosciences, Cambridge, UK), according to the manufacturer’s instructions.
LPS (20 ng/mL) was hydrolyzed with wheat phytase (11.44 × 10−3 mU/mL) for 1–3 h, respectively and different levels of LPS (10 and 40 ng/mL) were treated with the enzyme for 3 h. The residual toxicities of the LPS were assayed at OD 545 nm using ToxinSensor chromogenic LAL (limulus amebocyte lysate)-based endotoxin assay kit (GenScript, Piscataway, NJ, USA), according to the manufacturer’s instructions.
Human aortic endothelial (HAE) cell and human colorectal adenocarcinoma HT-29 cell were purchased from American Type Culture Collection (ATCC, Manassas, VA, USA). These two cells were used as well-established systems to measure LPS cytotoxicity and gut-induced IL-8 secretion, respectively. The former was maintained in cascade biologics medium 200 (Gibco Life technologies, Carlsbad, CA, USA) containing low serum growth supplement kit (Gibco Life technologies), and the latter in McCoy’s 5A medium (Gibco Life technologies) containing 10% fetal bovine serum and 1% penicillin-streptomycin solution (Gibco Life technologies). These cells were cultured at 37°C in a humidified incubator with 95% air and 5% CO2.
HAE cells were initially seeded onto a 96-well plate at a concentration of 2 × 104 cells per well and cultured until 80% confluency. LPS (2 mg/mL) was incubated at 37°C for 1 h with or without wheat phytase (28.6 mU/mL). Aliquots (10 μL) of the reaction mixtures were added to the cells with final concentration of 200 μg/mL LPS at 37°C for 24 h in the CO2 incubator. In addition, aliquots of the reaction mixtures were applied to the cells with final concentration of LPS (100, 200, and 400 μg/mL), respectively. The cell viabilities were measured at OD 450 nm using EZ-CYTOX kit (DogenBio, Seoul, Korea), according to the manufacturer’s instructions.
HT-29 cells were initially seeded onto a 96-well plate at a concentration of 2 × 104 cells per well and cultured until 80% confluency. LPS (100 μg/mL) was incubated at 37°C for 2 h with or without wheat phytase (286 mU/mL) and aliquots (10 μL) of the reaction mixtures were added to the cells with final concentration of 10 μg/mL LPS at 37°C for 24 h in the CO2 incubator. In addition, aliquots of the reaction mixtures were applied to the cells with final concentration of LPS (5, 10, and 20 μg/mL), respectively. The levels of IL-8 secreted into the culture media were assayed at OD 450 nm using Cymax™ human IL-8 ELISA kit (Ab FRONTIER, Seoul, Korea), according to the manufacturer’s instructions.
Statistical significance between groups was determined by a one-way analysis of variance using PROC GLM (SAS 9.4, SAS Institute, Cary, NC, USA) followed by Duncan’s multiple range test or by Student’s t-test. A p-value < 0.05 was considered as statistically significant difference.
RESULTS
As shown in Fig. 1A, wheat phytase hydrolyzed LPS, significantly releasing inorganic phosphate for 1 h (p < 0.05). In addition, the enzyme dephosphorylated LPS in a dose-dependent fashion (p < 0.05) (Fig. 1B). Previously, alkaline phosphatase was regarded as a sole enzyme that degraded LPS, whether it is a tissue-specific or tissue-non-specific type [10,11,15]. Nevertheless, our novel finding is remarkable considering that wheat phytase has highly strict substrate specificity for phytate with little or no phosphatase activity against other physiologically-relevant phosphorylated conjugates such as simple sugar phosphates, ATP, adenosine diphosphate, adenosine monophosphate, guanosine triphosphate and phosphoenolpyruvate [13]. Furthermore, the degradation of LPS by wheat phytase was nearly unaffected by the addition of L-phenylalanine, the inhibitor of tissue-specific alkaline phosphatase or L-homoarginine, the inhibitor of tissue-non-specific alkaline phosphatase (Figs. 2A and 2B). Indeed, bovine intestinal alkaline phosphatase inhibited the dephosphorylation of LPS in the presence of L-phenylalanine, but not L-homoarginine [16], while murine uterine alkaline phosphatase inhibited the dephosphorylation of LPS in the presence of L-homoarginine, but not L-phenylalanine [15]. This present result suggests that the catalytic mechanism of LPS hydrolysis by wheat phytase may vary from that of alkaline phosphatases.
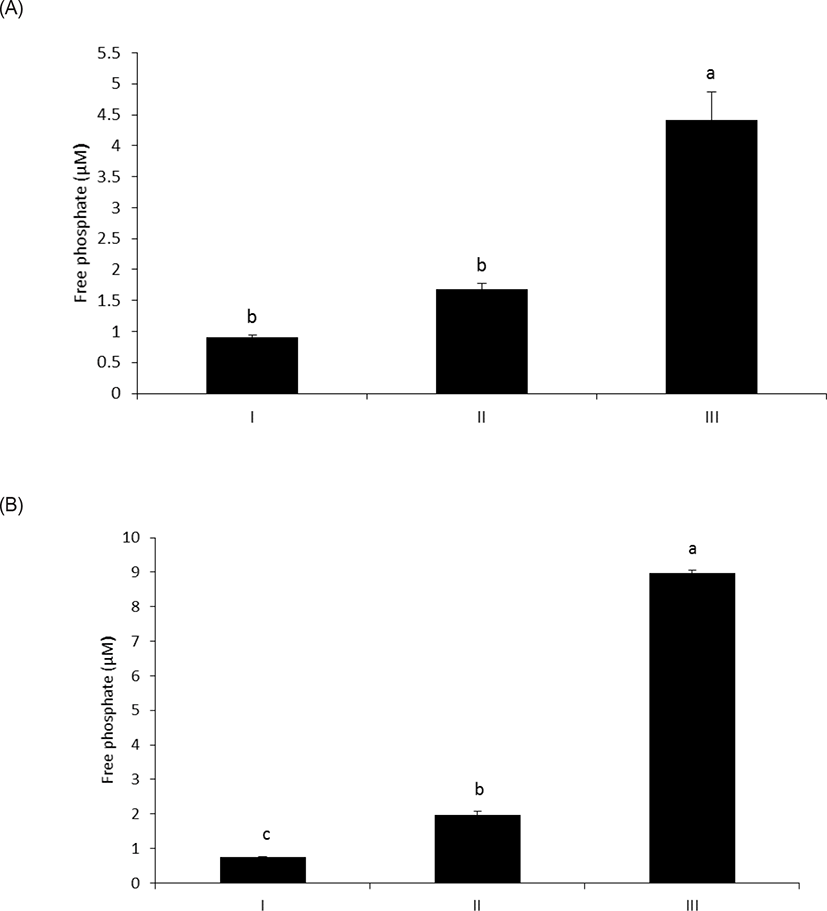
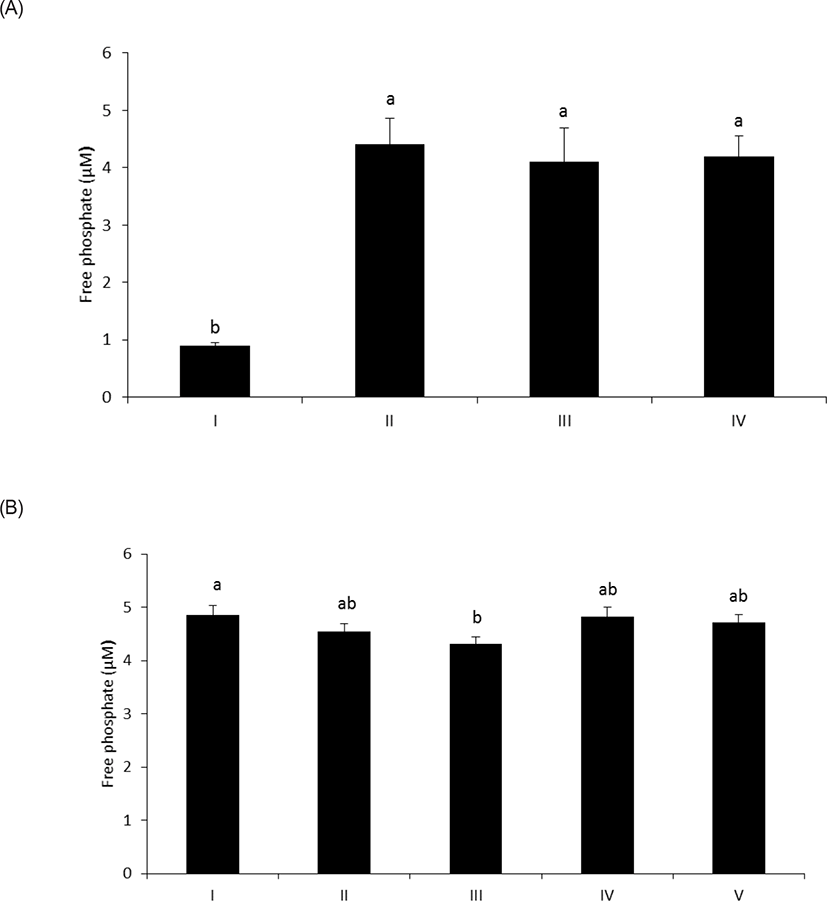
As shown in Figs. 3A and 3B, wheat phytase effectively reduced the in vitro toxicity of LPS, and 63% and 54% of its initial toxicity was retained after 1 h and 3 h of the enzyme reaction, respectively (p < 0.05) (Fig. 3A). In this regard, Koyama et al. [10] demonstrated that the structural destruction of LPS by enzymatic dephosphorylation was closely associated with LPS detoxification, due to defective binding to the effector cells.
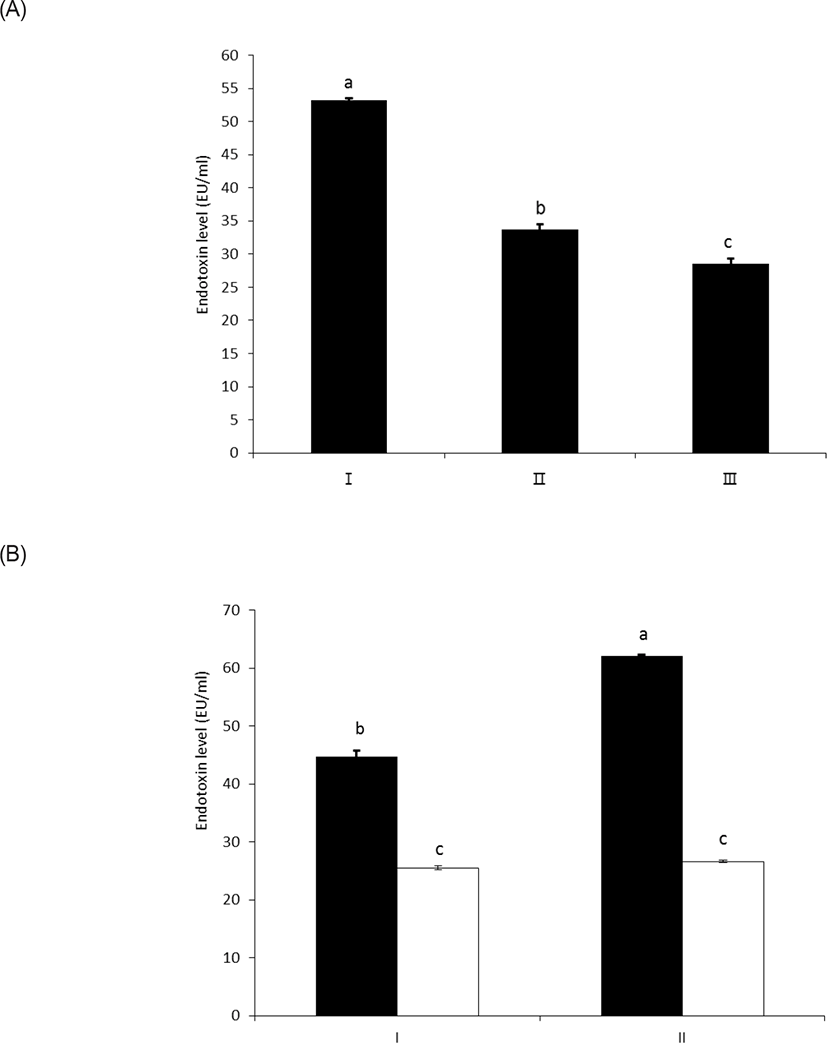
As shown in Figs. 4A and 4B, intact LPS clearly decreased the cell viability of HAE cells with unusual sensitivity [17]. However, the LPS dephosphorylated by wheat phytase counteracted the inhibitory effect on cell viability. Meanwhile, it was reported that the cell viability of HAE cells was enhanced following exposure to LPS and was attributed to the presence of alkaline phosphatase induced by IL-6 [10,18].
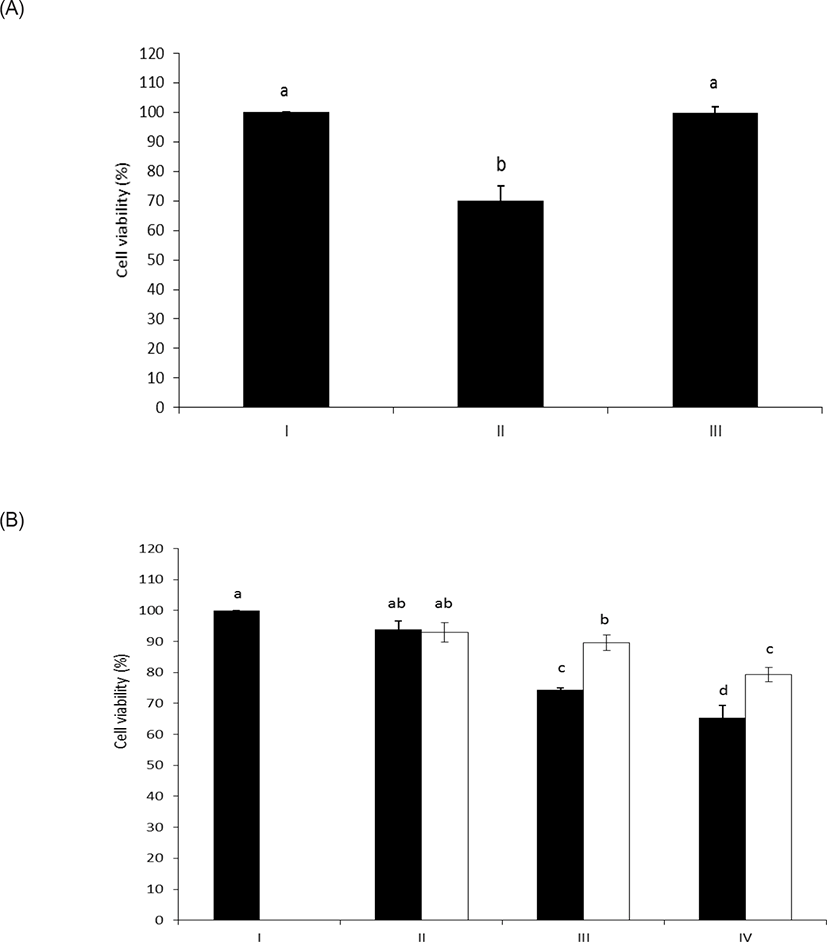
LPS treated with wheat phytase decreased IL-8 secretion from intestinal epithelial cell line, HT-29 cells to 14% (p < 0.05) when compared with intact LPS (Fig. 5A). In particular, the decrease of IL-8 secretion from the cells was effective at 20 μg/mL of LPS treated with the enzyme (Fig. 5B). It was known that LPS specifically exerts its inflammatory effects via IL-8 in HT-29 cell [19], via appropriate interaction of phosphorylated lipid A moiety of LPS with toll-like receptor 4 (TLR4)-MD2 complex on the cell surface induced by interferon gamma (IFN γ) [20,21]. Thus, the dephosphorylation of LPS by wheat phytase may suppress the side-effects of excessive inflammation induced by IL-8 over-expression [22].
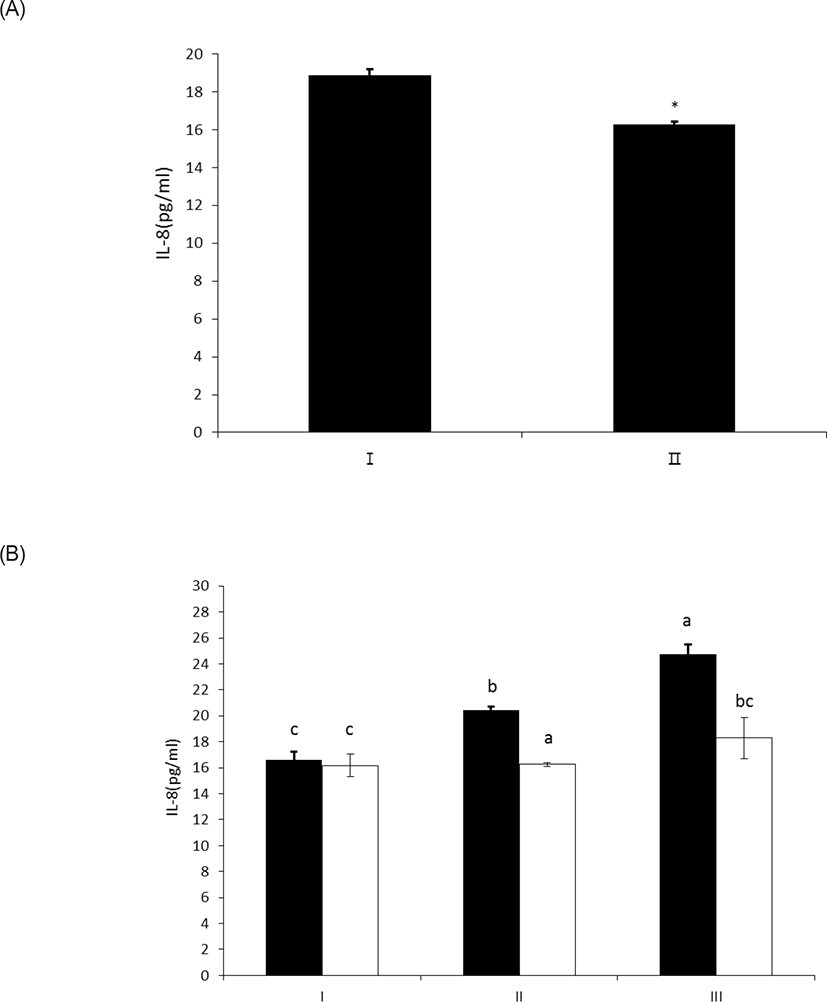
DISCUSSION
In animal husbandry, control of infection by pathogenic Gram-negative bacteria and related LPS-mediated inflammatory diseases can be addressed by the use of antibiotics and specific medications, or via application of immunostimulatory supplements such as probiotics and prebiotics. Indeed, antibiotics and certain medications such as the so-called chemical agents are associated with the increased resistance and abuse, harm and public dislike [23–25]. The aforementioned immunostimulatory supplements exhibit indirect efficacy against the targets by boosting specific immune cells in the body [26], which may be mild, variable and even unclear, despite the wide range of products that are commercially available [27,28].
Previously, the occurrence of necrotizing enterocolitis known as a severe intestinal inflammatory disease in premature infants and rat pups was closely associated with the exposure of LPS and the lack of endogenous intestinal alkaline phosphatase activity [29]. The recovery of exogenous alkaline phosphatase activity by breast milk feeding in them helped to weaken LPS-induced inflammation and toxification [29]. Therefore, wheat phytase may act as an efficient reservoir of the phosphatase activity for LPS hydrolysis and detoxification in the intestine.
In our study, the mechanism of wheat phytase involved in the reduction of IL-8 secretion in HT-29 cells under LPS stimulus was still unclear at a cellular level. In this regard, the supplementation of exogenous intestinal alkaline phosphatase leaded to the activation of endogenous intestinal alkaline phosphatase for LPS hydrolysis in porcine intestine extracts through the enhancement of a canonical inflammatory regulator, nuclear factor kappa-light-chain-enhancer of activated B cells (NF-kB) [30], which may down-regulate IL-8 signaling.
Some studies suggested that the supplementation of phytase could have a direct effect on enhancing the immune performance of farm animals. For example, inorganic phosphate released from phytate by phytase can modulate the virulent phenotypes of pathogens in the intestine of weaned pigs [31], and the parameters such as the percentage of lymphocytes and the amount of mucosal immunoglobulin (Ig) A and serum Ig M were improved with phytase in broilers [32,33]. Therefore, inorganic phosphate hydrolized from LPS by wheat phytase may positively work on diminishing the intensity of inflammatory response in HT-29 cells.
Wheat phytase is a potential therapeutic candidate and prophylactic agent for control of infections induced by pathogenic Gram-negative bacteria and associated LPS-mediated inflammatory diseases. Additionally, it is a potential feed additive beyond its conventional role in improving phosphate availability and preventing phosphate pollution. The activity of wheat phytase is mediated via relatively direct, safe and enzymatic mechanisms. Future studies exploring the possibility of mass production of wheat phytase are warranted.