INTRODUCTION
Exogenous enzymes have been used in the poultry industry for a long time, and in recent years, interest in feed enzyme technology to maintain or improve growth performance as a result of increased feed costs and environmental concerns has emerged [1–3]. It is well documented that enzymes accelerate the digestion of ingredients and improve the assimilation of less digestible feed as a result of catalysts secreted by cells in the gastrointestinal tract of broilers [4,5].
Dietary proteases have been used to improve protein availability by breaking down protein-bound starch within feed ingredients [6,7]. The proteases improve digestion by separating the protein-starch bonds effectively and this facilitates the use of extra energy by the animal [8–10]. Adeola and Cowieson [11] demonstrated that broilers fed a diet supplemented with a dietary protease had a higher protein utilization compared to those fed a diet without a dietary protease. Moreover, multiple proteases with different pH optima and substrate specificity have been proposed to improve the digestion of soybean meal, and thus increasing protein intake and utilization, improving poultry physiology, and reducing environmental impact [12,13].
The combined action of different forms (i.e., alkaline and neutral) of proteases can be synergistically used in the animal’s digestive tract by increasing free amino nitrogen content compared with the supplementation of a single protease [14,15]. This suggests that supplementation with a multi-protease could be cost-effective by reducing crude protein (CP) and synthetic amino acids (AA) in the feed formulation due to the increased bioavailability of AA in supplied protein [16–18]. In this light, the present study was designed to determine the effects of a multi-protease on the growth performance, carcass traits, proximate analysis of breast meat, and ileal digestibility of broilers fed CP- and AA-deficient diets. The hypothesis conducted was that supplementation of multi-protease into AA-deficient diets would improve growth performance and nutrient digestibility in broilers for 35 days after hatching.
MATERIALS AND METHODS
The experiment was conducted by using a total of 448 one-day-old Ross 308 male broiler chickens for 35 days. Birds were allocated into eight dietary treatments. Each dietary treatment contained eight replicate pens. Seven birds were housed in each raised battery cage (76 × 61 × 46 cm3), with similar body weight (BW) (36.96 ± 0.37 g; p > 0.05). Birds were offered the experimental diets on an ad libitum basis and had free access to fresh and clean drinking water via nipple drinkers throughout the experimental period.
The experiment was conducted using a completely randomized design. The eight dietary treatments were: (1) conventional standard positive control (PC, PC_1–3 week: 21.5% CP, 3,060 kcal/kg metabolizable energy, and PC_4–5 week: 19.5% CP, 3,200 kcal/kg metabolizable energy), (2) PC plus multi-protease 150 g per ton (PC-150), (3) CP (minus 0.5% from PC) and AA (minus 2% of lysine, methionine, threonine and methionine plus cysteine) deficient-diet (negative control [NC, NC_1–3 week: 21.0% CP, 3,060 kcal/kg metabolizable energy, and NC_4–5 week: 19.0% CP, 3,200 kcal/kg metabolizable energy), (4) NC plus multi-protease 150 g per ton (NC-150), (5) NC plus a multi-protease 300 g per ton (NC-300), (6) CP (minus 1% from PC) and AA (minus 4% of lysine, methionine, threonine and methionine plus cysteine) deficient-diet (extreme negative control [ENC], ENC_1–3 week: 20.5%, 3,060 kcal/kg metabolizable energy, and ENC_4–5 week: 18.5%, 3,200 kcal/kg metabolizable energy), (7) ENC plus multi-protease 150 g per ton (ENC-150), (8) ENC plus multi-protease 300 g per ton (ENC-300). Corn and SBM control diets were formulated to meet the Ross 308 nutrition specification [19] (Tables 1 and 2).
The eight dietary treatments were: positive control (PC), negative control (NC: minus 0.5% from PC, and minus 2% of lysine, methionine, threonine and methionine plus cysteine), extreme negative control (ENC: minus 1% from PC, minus 4% of lysine, methionine, threonine and methionine plus cysteine), and plus multi-protease 150 or 300 g per ton (e. g., PC-150); PC, PC-150, NC, NC-150, NC-300, ENC, ENC-150, ENC-300.
Provided per kilogram of diet: vitamin A, 12,000 IU; vitamin D3, 2,500 IU; vitamin E, 30 IU; vitamin K3, 3 mg; D-pantothenic acid, 15 mg; nicotinic acid, 40 mg; choline, 400 mg; and vitamin B12, 12 μg; Fe, 90 mg from iron sulfate; Cu, 8.8 mg from copper sulfate; Zn, 100 mg from zinc oxide; Mn, 54 mg from manganese oxide; I, 0.35 mg from potassium iodide; Se, 0.30 mg from sodium selenite.
The values were calculated according to the values of feedstuffs [20].
The eight dietary treatments were: positive control (PC), negative control (NC: minus 0.5% from PC, and minus 2% of lysine, methionine, threonine and methionine plus cysteine), extreme negative control (ENC: minus 1% from PC, minus 4% of lysine, methionine, threonine and methionine plus cysteine), and plus multi-protease 150 or 300 g per ton (e. g., PC-150); PC, PC-150, NC, NC-150, NC-300, ENC, ENC-150, ENC-300.
In addition, Cr2O3 (Chromium oxide powder, > 99.9% purity, Sigma-Aldrich, St. Louis, MO, USA) was added as an internal indigestible marker for digestibility analysis in a proportion of 0.3% to all experimental eight diets. This study has employed Kemzyme® protease (Kemin Industries Pte Limited., Singapore) as a multi-protease.
BW was recorded at the start and on every week of the experimental periods, and feed consumption was recorded on the same date of BW recording to calculate average daily gain, average daily feed intake, and feed conversion ratio.
Eight birds per treatment (one bird per cage) were selected randomly and euthanized by cervical dislocation for sample collection on day 21 and 35. After evisceration, empty bodies were weighed. Drumsticks (skinless) and breast meat were removed from carcasses and weighed. The empty BW, drumstick, and breast meat weight were expressed as proportions relative to slaughter live BW.
The digesta samples were pre-dried at 55°C for 24 h, ground through a 0.75 mm sieve (ZM 200 Ultra-Centrifugal Mill, Retsch GmbH & Co. KG, Haan, Germany), and analyzed for levels of dry matter, CP (Macro-Kjeldahl, N × 6.25 [1/0.16 = 6.25] to convert nitrogen content into protein content), ether extract, and gross energy according to the methodologies of AOAC [21]. Chromium oxide concentration of the feed and digesta samples were also analyzed [22]. The digestibility coefficient of nutrients was calculated as described by [23] using the following equation:
Where ID is the concentration of an indigestible marker in the diet; IF is the indigestible-marker concentration in ileal digesta; AF is the nutrient concentration in ileal digesta and AD is the nutrient concentration in the diet.
The AA contents in the diets were measured according to a method described by Cohen [24]. Samples were freeze-dried and grounded through a laboratory hammermill (1 mm screen; Cyclotec 1093, Tecator AB, Höganäx, Sweeden). A 200–300 mg sample was hydrolyzed with acid (6 M hydrochloric acid [HCl]) to convert protein-bound AA into free AA. The AA in the hydrolysate then underwent pre-column derivatization with 6-aminoquinolyl-N-hydroxysuccinimidyl carbamate. For tryptophan analysis, a separate 50–100 mg sample was hydrolyzed in an alkaline solution (5 M sodium hydroxide [NaOH]) and neutralized before pre-column derivatization. The AA derivatives were then separated and quantified by reversed-phase high-performance liquid chromatography (HPLC; ACQUITY UPLC system with ultraviolet [UV] detector, Waters Corporation, Milford, MA, USA). For all analyses, a Waters AccQ-Tag Ultra column (BEH C18, 2.1 × 100 mm2; 1.7 μm) was used with column temperature at 55°C, detection at 260 nm, and the flow rate 0.7 mL/min.
The intestinal samples (n = 6 per treatment group) were collected from broiler chickens at day-21 and day-35, respectively. Total RNA was extracted from using the HiGeneTM total RNA prep kit (BIOFACT, Daejeon, Korea). Their concentration and quality of RNAs were checked by NanoDrop®ND-1000 (NanoDrop Technologies, Wilmington, DE, USA). cDNAs were synthesized by using Quantitect® Reverse Transcription Kit (Qiagen, GmbH, Hilden, Germany). Quantitative real-time polymerase chain reaction (qRT-PCR) was carried out utilizing a StepOnePlus Real-Time PCR system (Applied Biosystems, Foster City, CA, USA), SFCgreen®I (BIOFACT) and the following gene-specific primers and beta-actin (Bioneer, Daejeon, Korea); ACC (forward) 5’-AATGGCAGCTTTGGAGGTGT-3’, (reverse) 5’-TCTGTTTGGGTGGGAGGTG-3’, PEPT1(forward) 5’-CCCCTGAGGAGGATCACTGTT-,3’ (reverse) 5’-CCTTGCAGTGGGCAGGAA-3’, GLUT2 (forward) 5’-CACACTATGGGCGCATGCT-3’, (reverse) 5’-ATTGTCCCTGGAGGTGTTGGTG-3’, beta-actin (forward) 5’-CCACCGCAAATGCTTCTAAAC-3’, (reverse) 5’-AAGACTGCTGCTGACACCTTC-3’. The relative quantification of gene expression was determined by the 2−ΔΔCt method and beta-actin was used as an internal control for quantification of target genes.
Data were analyzed according to a completely randomized design, using the general linear model (GLM) procedure of one-way ANOVA of SPSS software (Version 21; IBM SPSS 2012). The pen was used as the experimental unit for all growth performance measurements. Selected individual birds were considered as the experimental unit for the proportion of carcass trait weights, respectively. Mean differences were considered significant at p < 0.05. When treatment effects were significant (p < 0.05), means were separated using Turkey’s multiple range test procedures of SPSS software. To determine the optimum multi-protease level in diets, linear-plateau and quadratic-plateau regression analysis were conducted using a Nutritional Response Model (Version1.1; 16) as described previously by Wickramasuriya et al. [25].
RESULTS
Table 3 shows the effect of a multi-protease feeding of a crude protein and AA-deficient diet on growth performance for 35 days after hatching. Overall, the multi-protease-supplied groups had a higher BW than the non-multi-protease groups (PC, NC, and ENC); in particular, a higher BW was observed on days 7, 28, and 35 (p < 0.05). Average daily gain (ADG) on days 7 and 28 was higher with multi-protease (p < 0.05). There was no difference in average daily feed intake (ADFI) among treatments during the 35 days (p > 0.05). Feed conversion ratio (FCR) was lower (p < 0.05) in the groups fed with multi-protease compared to groups without multi-protease on day 28. However, linear-plateau and quadratic-plateau regression analyses did not reflect any improvement in growth performance among treatments (p > 0.05). Feeding a diet with multi-proteases improves the growth performance of broilers.
The eight dietary treatments were: positive control (PC), negative control (NC: minus 0.5% from PC, and minus 2% of lysine, methionine, threonine and methionine plus cysteine), extreme negative control (ENC: minus 1% from PC, minus 4% of lysine, methionine, threonine and methionine plus cysteine), and plus multi-protease 150 or 300 g per ton (e. g., PC-150); PC, PC-150, NC, NC-150, NC-300, ENC, ENC-150, ENC-300.
There are no significance linear-plateau and quadratic-plateau regression obtain on growth performance for 5 weeks after hatching.
The effects of a multi-protease on carcass traits of 21- and 35-day-old broilers are shown in Table 4. The empty BW and weight of drumsticks were not different among feed treatments in the absence or presence of a multi-protease by days 21 and 35 (p > 0.05). On the other hand, the percentage of breast meat was increased in groups fed a multi-protease compared to each control group (PC, NC, and ENC; p < 0.05). Therefore, when comparing the results of NC and ENC in the absence or presence of a multi-protease, protein accumulation could have improved the deposition of breast meat in the broilers.
The eight dietary treatments were: positive control (PC), negative control (NC: minus 0.5% from PC, and minus 2% of lysine, methionine, threonine and methionine plus cysteine), extreme negative control (ENC: minus 1% from PC, minus 4% of lysine, methionine, threonine and methionine plus cysteine), and plus multi-protease 150 or 300 g per ton (e. g., PC-150); PC, PC-150, NC, NC-150, NC-300, ENC, ENC-150, ENC-300.
In the standard and protein and AA-deficient diets, the quality of breast meat was compared in broilers at days 21 and 35 with or without a multi-protease (Table 5). Overall, no effects of dietary treatment were found on the moisture, CP, crude fat, and crude ash (p > 0.05). However, a numerical tendency towards an increased percentage of CP in the breast meat was observed in broilers fed a multi-protease under ENC on day 35 (ENC, 20.78%; ENC-150, 20.94%; ENC-300, 21.21%; p > 0.10).
The eight dietary treatments were: positive control (PC), negative control (NC: minus 0.5% from PC, and minus 2% of lysine, methionine, threonine and methionine plus cysteine), extreme negative control (ENC: minus 1% from PC, minus 4% of lysine, methionine, threonine and methionine plus cysteine), and plus multi-protease 150 or 300 g per ton (e. g., PC-150); PC, PC-150, NC, NC-150, NC-300, ENC, ENC-150, ENC-300.
The effect of a multi-protease in protein and AA-deficient diets on ileal digestibility in broilers on days 21 and 35 are presented in Table 6. Feeding a diet with a multi-protease (i.e., up to 150 g/ton) improved (p < 0.05) ileal digestibility of dry matter and CP on days 21 and 35 compared to those fed without a multi-protease (i.e., PC, NC, and ENC).
The eight dietary treatments were: positive control (PC), negative control (NC: minus 0.5% from PC, and minus 2% of lysine, methionine, threonine and methionine plus cysteine), extreme negative control (ENC: minus 1% from PC, minus 4% of lysine, methionine, threonine and methionine plus cysteine), and plus multi-protease 150 or 300 g per ton (e. g., PC-150); PC, PC-150, NC, NC-150, NC-300, ENC, ENC-150, ENC-300.
The transcription levels of genes involved in the intestinal nutrient transporter genes, such as ACC (acetyl-CoA carboxylase), PEPT1 (peptide transporter 1), and GLUT2 (glucose transporter 2) were examined. There was no dramatic increase in expression over 2-fold, but in the multi-protease, treated groups, particularly NC-150 and ENC-150, the genes were upregulated (Fig. 1; p > 0.10).
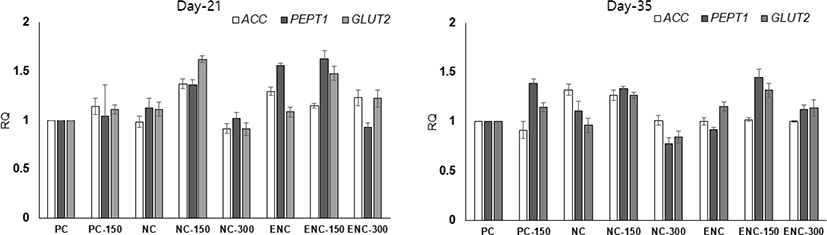
DISCUSSION
A study related to enzyme ability in corn-soybean meal-based diets demonstrated that specific enzyme activities could enhance the absorption of nutrients in broiler intestines [26]. Thus, research on exogenous enzymes (i.e., carbohydrase, phytase, and protease) has been conducted to improve the digestibility of nutrients in animals [27,28]. The addition of exogenous proteases to poultry and swine diets has been attempted over the past decades to improve the status of deficient endogenous peptidases in the neonate.
There are inconsistent results from protease incorporation into the lower protein diets in previous studies because the accessibility of exogenous enzymes highly depends on the protein matrices and cell wall structure of the protein sources. Therefore, it is important to consider the protein structure and cell wall components present in the protein sources in low protein diets to obtain better broiler performance by adding protease [29].
Production of digestive enzymes that remove intestinal endotoxins is insufficient for 21 days after hatching because pancreatic proteases predominate in the initial post-hatch period [30]. In addition, poultry less than 14 days of age has less developed viscera and there is a shortage of endogenous digestive enzyme secretions, so supplementation of an exogenous enzyme is more effective at this stage [31,32]. Lower protein diets with exogenous proteases can improve negative environmental effects by reducing nitrogen excretion (i.e., fecal and urinary nitrogen) [18,33,34]. Incorporating exogenous protein enzymes into the feed to ameliorate the anti-nutritive effects of a protein source is a common strategy for poultry [35]. Numerous dietary enzymes (i.e., protein digestive enzymes) have long been used to maximize the production of broilers by improving the digestibility of nutrients [36,37]. There are reports of positive results in the supplementation of exogenous proteases in diet [36,38,39]. In the current study, the supplementation of multi-proteases in CP and AA deficient-diets has improved growth performance and nutrient digestibility in broilers.
The utilization of multi-proteases has been proven by sodium dodecyl sulfate-polyacrylamide gel electrophoresis (via the separation of phosphoproteins) to involve trypsin alone, improving the protein sequence coverage due to the increasing diversity of the peptide sequences, and this can facilitate the identification of protein sequences lacking tryptic cleavage sites by application of different cleavage site specificities [40,41]. Non-starch polysaccharides (NSP) in cereals and soybeans provide physical barriers to prevent the action of exogenous enzymes on starch and protein [42]. However, the relationship between the starch granules and protein structures in broilers may disrupt the absorption of starch. In the absence of exogenous enzymes, starch granules are closely associated with the protein matrix, which impedes the accessibility of the endotoxin enzymes, which may reduce starch digestion in the small intestine [43]. Amylolytic enzymes can act upon the starch granules when the protein matrix has been digested by exogenous proteases [9]. This means that feeding a diet with exogenous proteases can help to reduce the requirement of energy and AA by assisting peptidase production [8].
In the results of growth performance, NC-300 (BW, day 7, 28, and 35; ADG, days 7, 28, 29–35, and 1–35) showed similar data to those of the PC diet. Low protein diets with supplementation of proteases (i.e., up to 200 mg/kg) showed similar results to the growth performance of standard CP diets [36]. Several studies have reported improvements in weight gain and FCR under low CP diets with exogenous proteases compared to standard protein diets [39]. The main effect of multi-protease on the final BW explains a maximum of 5.03% of the variance (ENC vs. ENC-300). The results demonstrated that the cumulative supplementation of protein enzymes had a significant increase in the BW by improving the digestibility of CP and energy.
The study was designed to compare CP- and AA-deficient diets with a standard (control) diet. Supplementation of exogenous proteases could complement the activity of endogenous proteases, thus showing significant effects in the case of this study. It is the supplementation of proteases that directly affect the digestibility of AA.
Environment and other management factors influence the use of nutrients indirectly. These indirect external factors include disease status, nutrient balance, feed processing conditions, age, and animal species. There have been reports of changes in apparent ileal AA digestibility in a range of standard diets depending on the age of the animals [44]. AA digestibility may theoretically increase with the age of animals. However, AA digestibility on day 42 decreased on wheat and canola meal diets (day 42: 75.8% vs. day 14: 78.7%) compared to that on day 14 [44]. The above description may be beyond the scope of the present study. The changes in AA digestibility with animal age are explained in the literature [44–46]. In addition to the growth stage, various other factors modulate the digestibility of AA in the diets of pigs and broilers, and so will invariably influence protease efficacy. Other than animal age, there are also factors (e.g., dietary fat concentration, xylanase, phytase, dietary calcium or limestone inclusion, supplementary AA and AA density, ingredient type, and quality, anti-nutrients, and hydrothermal conditioning) that alter AA digestibility in poultry, which may be closely related to the efficacy of proteases [27,47,48,50]. Nonetheless, poultry fed a diet supplemented exogenous proteases are able to improve protein digestibility regardless of those factors.
Supplying proteases to maximize the utilization of protein is an opportunity to compensate for the lack of AA [50]. Although there was no significant difference in empty BW and drumstick weight, the breast meat yield was higher in diets with multi-proteases in the present study (i.e., up to 150 g/ton). The factors affecting the breast meat of broilers in this study might be attributed to the availability of AA. Supplementation of lysine, methionine, and valine in diets may have a direct effect on breast meat of broilers [51,52]. To increase breast meat in broilers, a study on the improvement of AA digestibility through exogenous protease supplementation is needed.
The imporovement of nutrient digestibility due to multi-protease could influence and/or upregulate the expression of genes related to protein digestible transportersin the intestine [53,54]. In this light, we have expected that those transporters and/or relatedgenes would be altered by the multi-protease However, multi-protease supplementation improved the ileum digestibility of dry matter, crude protein, and energy but did not increase the expression of ACC, PEPT1, and GLUT2 in the present study. Although the unexpected results could be due to unknown responses of gene expression or a limited number of samples or higher variation, which is an important part of data for future investigations intending to conduct advanced poultry productivity.
In conclusion, multi-proteases added (up to 150 g/ton) to CP- and AA-deficient diets for 35 days improve both ADG (PC vs. PC-150, 1.60%; NC vs. NC-150, 1.22%; NC vs. NC-300, 2.73%; ENC vs. ENC-150, 1.00%; ENC vs. ENC-300, 5.14%) and FCR (PC vs. PC-150, 5.15%; NC vs. NC-150, 2.78%; NC vs. NC-300, 4.96%; ENC vs. ENC-300, 5.59% without ENC vs. ENC-150) in broilers compared to standard diets.