INTRODUCTION
The reproductive efficiency of dairy and beef cows are important factors influencing the production of milk and meat. In cattle, it is well known that a negative energy balance (NEB) has detrimental effects on reproductive efficiency, such as delaying the estrus cycle [1], reducing the embryo quality [2], and reducing pregnancy rate after insemination [3]. Body condition score (BCS) is used to determine the amount of metabolized energy in the fat and muscle of a live animal [4]. Postpartum cows with NEB and low BCS had abnormal estrus cycles until a normal BCS was restored [5]. The in vivo embryo quality after multiple superovulations, artificial insemination (AI), and embryo recovery depends on the BCS of the donor cow [6]. The nutrient requirements (NR) affect nutrition intake, blood metabolites, ovarian function, the estrus cycle, and fertility [7]. Blood metabolites change with the feeding status, and their levels are used as criteria to evaluate cows’ nutritional status [8]. Glucose is mainly metabolized via the glycolytic pathway [9] and used as an energy source for the maturation of oocytes and expansion of the surrounding cumulus cells in vitro [10]. Total cholesterol plays an important role in steroidogenesis regulation in the ovary [11].
Triglycerides are a major lipid in bovine oocytes; for instance, lipolytic activity increases in bovine oocytes during maturation, and the oocytes utilize triglycerides to generate adenosine triphosphate (ATP) [9]. Dairy cows with NEB during ketosis and post parturition had low triglyceride levels. In cows with NEB, stored triglycerides in the adipose tissue are degraded to restore the energy balance [12]. Excess lipids in circulation accumulate as triglycerides in the liver. Alanine aminotransferase (ALT) and aspartate aminotransferase (AST) concentrations in the plasma can be used as liver injury indicators for stored triglycerides in the liver [13].
Elevated plasma urea nitrogen concentrations are caused by high dietary crude protein feeding, and it is associated with decreased fertility in dairy cows [14]. Fertilized oocytes in cows fed high and moderate levels of protein had similar morphological blastocyst developments. However, cows in the moderate feeding group showed a relatively high pregnancy rate after embryo transfer [14]. Different plasma urea nitrogen concentrations affect oocyte competence and embryo developmental rate in vitro [15]. A normal serum albumin concentration in donor cows can be used to predict the in vitro developmental competence of oocytes [16].
It is well known that non-esterified fatty acids (NEFA) is an indicator of cow NEB. Post parturition cows have been shown to have higher NEFA concentrations, produce less milk [17], and have lower fertility [18]. Elevated NEFA concentrations have negative effects on cow granulosa cell viability and cell growth [19], and induce apoptosis in human granulosa cells [20]. However, the nutritional status and effects of changes in blood metabolites, BCS, estrus cycle, and embryo quality have not been thoroughly investigated in Hanwoo cows.
To obtain high quality embryos from elite cows, multiple steps of ovulation, including hormone treatment, AI, and embryo recovery, should be adapted in fields [21]. Farm owners select elite cows to be used as donors based on their BCS, but this is the only factor they use when selecting donors. In farms, commercial concentrates and hay are the basic feeds used for Hanwoo cows, and there is no NR guideline for multiple superovulations or embryo recovery. Therefore, we examined the estrus cycle, blood metabolites, BCS, and embryo recovery rate after multiple superovulations and AI based on different NR.
MATERIALS AND METHODS
Nineteen Hanwoo cows (mean age = 32.3 ± 6.9 months, mean body weight = 436.7 ± 38.3 kg) were from the Hanwoo Research Institute, National Institute of Animal Science, Rural Development Administration, Korea, were used in the study. Animal study design were approved by the Institutional Animal Care and Use Committee of the National Institute of Animal Science, Korea (Approval number: NIAS 20191557). The cows were randomly divided into three groups: 80%, 100%, and 120% NR groups (containing 6, 8, and 5 cows, respectively). The mean body weight was described as shown follow: 80% NR group = 455.0 ± 28.3 kg, 100% NR group = 417.8 ± 26.9 kg, 120% NR group = 437.4 ± 52.3 kg. All the cows were raised in a pen using a group feeding procedure based on the Korean feeding standard for Hanwoo cows. Commercial formulated concentrates and hay (straw rice) were fed to Hanwoo cows according to the experimental design from December 26, 2018 to April 29, 2019. The 100% NR group (control) was fed 2.0 kg of formulated concentrate and 4.0 kg of hay per cow. The 80% NR group was fed 1.5 kg of formulated concentrate and 2.4 kg of hay per cow. The 120% NR group was fed 3.0 kg of formulated concentrate and 5.0 kg of hay per cow. The chemical compositions of the concentrates and hay are provided in Table 1.
Components | Concentrates (%) | Hay (%) |
---|---|---|
Crude protein | 13.5 | 2.7 |
Ether extract | 1.5 | 1.98 |
Ca | 0.7 | 0.13 |
P | 0.7 | 0.05 |
Crude fiber | 15.0 | 34.57 |
Crude ash | 10.0 | 11.46 |
TDN | 69.0 | 53.37 |
Heat detection was conducted every morning and afternoon for 30 min from February 21, 2019 to April 29, 2019. To define heat estrus, estrus detection patches (Estrotect™, Rockway, Spring Valley, WI, USA) were applied about mid-way between the hip and tail head as previously described [22]. To determine the estrus cycle in Hanwoo cows, the follicular wave, ovulation, and corpus luteum formation were examined twice each week (Monday and Thursday) by ultrasonography with 7.5 MHz probe (NL/MyLab, Esaote, Genova, Italy).
Blood collection was conducted after 2 h of feeding twice each week (Monday and Thursday) in the morning from February 21, 2019 to April 29, 2019. Ten milliliter of blood samples was collected from the veins of cows using a serum vacutainer (BD vacutainer serum REF 367820, Plymouth, UK) and transferred to the laboratory. Serum was collected by centrifuging the blood sample at 1,763×g at 4°C for 15 min. The collected serum was cryopreserved in a deep freezer until analysis. We evaluated the following blood metabolites using a blood metabolite analyzer (7020 Automatic Analyzer, Hitachi, Tokyo, Japan): glucose (mg/dL), total cholesterol (mg/dL), triglyceride (mg/dL), blood urea nitrogen (BUN, mg/dL), AST (IU/L), ALT (IU/L), NEFA (ЧEq/L), albumin (ALB, g/dL), and total protein (TP, g/dL).
Nineteen Hanwoo cows with a synchronized estrus cycle were inseminated, and their embryo was recovered between May 7, 2019 and August 1, 2019. Briefly, 1.9 g of a progesterone-releasing intravaginal device (EAZI-BREED CIDR 1900, Zoetis, Belgium) was introduced to the vagina of the cows for 7 days, irrespective of the point in the estrus cycle. Four days after CIDR introduction, 28 AU of follicle-stimulating hormones (FSH, Antrin, Japan) was administrated in decreasing dosages twice per day for 4 days (5, 4, 3, and 2 FSH per administration on days 1 to 4, respectively, at 7:30 AM and 5:30 PM). Six days after CIDR introduction, 25 mg of prostaglandin analog (dinoprost tromethamine; Lutalyse, Zoetis, Belgium) was injected intramuscularly in the morning, and then CIDR was removed. Two days after CIDR withdrawal, AI was accompanied by intramuscular injection of 2 mL of gonadotrophin-releasing hormone (Fertagyl, Inervet International GmbH, Unterschleissheim, Germany). A second AI was carried out after 2.5 days of CIDR withdrawal. AI was conducted by two well-trained technicians. Freeze–thawed semen from one bull was used for AI. After 7 days of the first AI, embryos were recovered nonsurgically into embryo recovery fluid (Agtech, Manhattan, KS, USA). The recovery fluid was filtered with an embryo collection filter (Agtech), and the embryos were recovered under a stereoscopic microscope. The embryos were classified according to the Manual of International Embryo Transfer Society [23]. As shown Fig. 1, the recovered embryos from the morulae to expanded blastocyst stages were evaluated, and those classified as excellent, good (code1), or fair (code2) quality were considered transferable embryos. Unfertilized oocytes and poor-quality embryos were considered as non-transferable embryos.
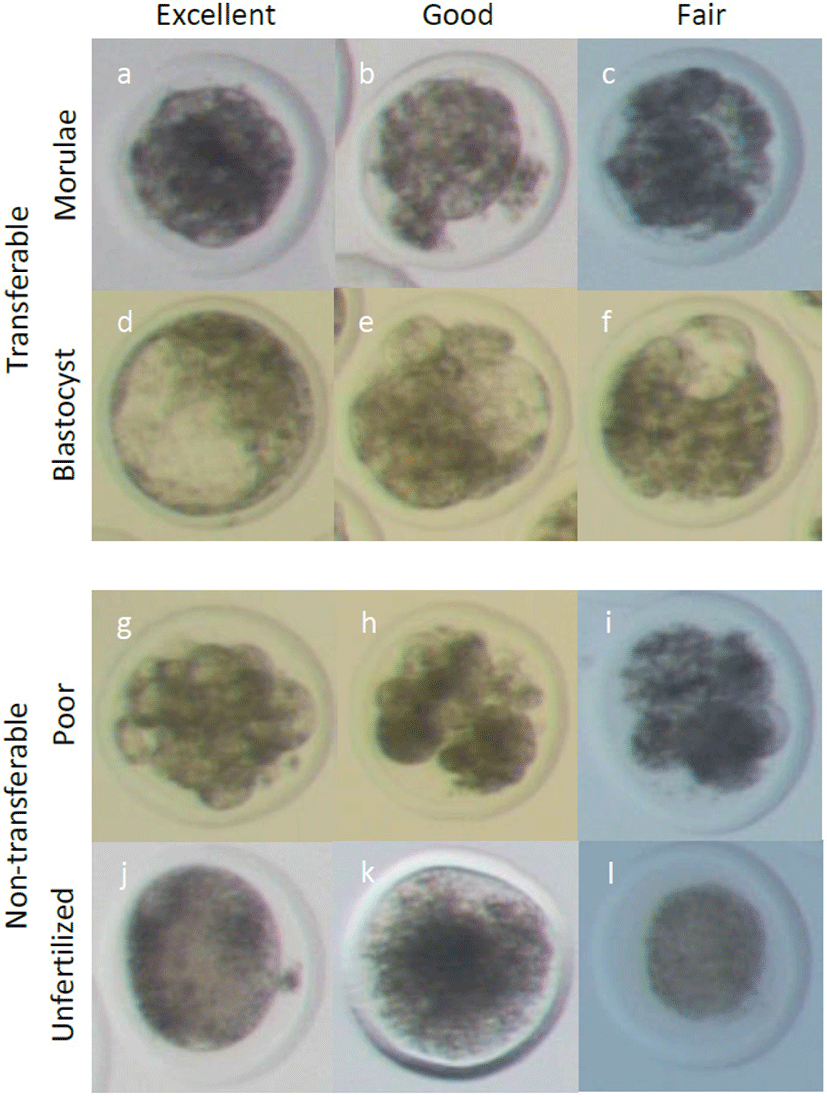
The blood metabolite levels and body weight among the different NR groups were compared using the one-way ANOVA, followed by Tukey–Kramer’s HSD post hoc test. All analyses were performed using SAS (Statistical Analysis System software, version 9.4). The number of transferable embryos among the NR groups was analyzed using the two-tailed Fisher’s exact test.
RESULTS
In the present study, we evaluated blood metabolites and the embryo recovery rate after multiple superovulation and AI in the different NR groups. As shown in Table 2, blood metabolites were changed by different NR. The glucose and ALB levels were not significantly different among the 80%, 100%, and 120% NR groups. Total cholesterol level among the 80%, 100%, and 120% NR groups was significantly different (126.5 ± 3.3, 152.6 ± 2.4, and 177.4 ± 1.8 mg/dL; p < 0.05). The level of ALT among the 80%, 100%, and 120% NR groups was significantly different (21.6 ± 0.4, 27.2 ± 0.5, and 30.6 ± 0.5 IU/LЧEq/L, respectively; p < 0.05). The triglyceride and BUN levels in the 120% NR group were significantly higher than that of the 80% and 100% NR groups (p < 0.05). The level of NEFA among the 80%, 100%, and 120% NR groups was significantly different (440.5 ± 18.2, 318.5 ± 23.1, and 195.1 ± 8.5 ЧEq/L, respectively; p < 0.05). The levels of AST and TP in the 80% NR group were significantly low compared that in the 100% and 120% NR groups.
The embryo recovery rate from cows in the different NR groups is shown in Table 3. The 120% NR group presented a significantly high percentage of transferable embryos compared with the 80% and 100% NR groups (55.9%, 50.0%, and 90.6% in the 80%, 100%, and 120% NR groups, respectively; p < 0.01). The body weight, BCS, and estrus cycle duration among the different NR groups are shown in Table 4. The mean body weight of the 80%, 100%, and 120% NR groups was significantly different (80%, 100%, and 120% vs. 364.6 ± 10.0, 431.0 ± 17.6 and 482.4 ± 20.3 kg, respectively, p < 0.05). The mean BCS among the 80%, 100%, and 120% NR groups was significantly different (p < 0.05). The mean estrus cycle duration among the 80%, 100%, and 120% NR groups was not significantly different. However, the 80% NR group showed extended estrus cycle (24.6 ± 2.8 days) compared with the 100% and 120% NR groups (20.8 ± 0.2 and 21.2 ± 0.5 days).
Recovered embryos were evaluated and divided into transferable and non-transferable embryo groups. Transferable embryo group selected from morulae to expanded blastocyst and divided into code 1 (excellent and good) and code 2 (fair). Non-transferable embryo group include the others; unfertilized oocytes, degenerated embryos, poor quality embryos having blastomeres of less than 16.
NR (%) | No. cow | Body weight (kg) | BCS | Estrus cycle (day) |
---|---|---|---|---|
80 | 6 | 364.6 ± 10.0c | 2.6 ± 0.1c | 24.6 ± 2.8 |
100 | 8 | 431.0 ± 17.6b | 3.2 ± 0.1b | 20.8 ± 0.2 |
120 | 5 | 482.4 ± 20.3a | 3.7 ± 0.1a | 21.2 ± 0.5 |
DISCUSSION
In the present study, we showed that different NR affects blood metabolites, number of transferable embryos after multiple superovulation with AI, BCS, and cow estrus cycle in Hanwoo cows. To the best of our knowledge, this study is the first to determine the productivity of transferable embryos from Hanwoo cows by different NR ratio. It has been demonstrated that the nutritional status of donor cow influences oocyte quality, embryo quality, and transferable embryo productivity in vivo [24]. Previous studies reported that feeding beef heifers a high-energy diet resulted in a low number of transferable in vivo embryos after multiple superovulation with AI [25] and a low number of in vitro embryos derived from the ovum pick up method in Gir cows [26]. In addition, the number of recovered embryos in the 180% maintenance energy or ad libitum of high-feed intake group after superovulations with AI was lower than that in the 70% or 100% maintenance energy of low-feed intake groups [27,28]. In the present study, we compared small differences in feed-intake level among 80, 100, and 120% NR groups. The 120% NR of feed intake group produced a higher number of transferable embryos compared with the 80% and 100% NR of feed intake group, as shown in Table 3. The 120% NR of feed intake was low feed intake level compared with previous studies. Previous studies examined the effect of large differences in the feed-intake level on transferable embryos after superovulation with AI. In a previous study, group fed approximately 3 kg of concentrate and that fed ad libitum produced 4.8 and 2.8 transferable embryos, respectively (p < 0.05) [25]. Approximately 70% of maintenance energy of the low-feed intake and 180% of high-feed intake group showed differences in the number of recovered embryos (10.5 vs. 6.7, p = 0.01) and transferable embryos (5.7 vs. 3.8, p = 0.17) [27]. The 100% and 180% maintenance feeding groups showed differences in the total number of embryos (14.1 vs. 9.5, p < 0.05) and number of viable embryos (10.1 vs. 6.7, p < 0.05) [28]. The 70% and 170% maintenance feeding groups showed differences in the number of viable (5.3 vs. 3.8, p = 0.028) and total number of embryos (9.9 vs. 6.7, p = 0.001) [29]. Previous studies demonstrated that low-diet feeding to cows is suitable for producing high quality and transferable embryos. We assumed that excessive feeding of cows might have increased the blood glucose level, which may disturb glucose transportation to the embryos and subsequently decrease the production of high quality embryos [24]. In addition, high-energy diet feeding can increase urea concentration in the blood and rumen, and this is associated with a low pregnancy rate in cows [25]. The results of a study comparing the embryo developmental rate between beef heifers fed high- and low-energy diets showed that the low-energy diet fed cows had a similar embryo quality to that observed in the present study. In beef heifers, the 125% NRC feeding level resulted in a higher embryo quality and more live cells in the embryo compared with the 80% NRC feeding level [2].
The effects of different NR on blood metabolites in Hanwoo cows are shown Table 2. The glucose and albumin levels were similar among the 80%, 100%, and 120% NR groups. We could not elucidate the effects of glucose and albumin levels on the embryo recovery rate after multiple superovulations with AI, but we proved that glucose level affects embryonic development in vitro. A high glucose concentration in the embryo culture media deleteriously affected the blastocyst developmental rate compared with a low glucose concentration in bovine [30] and mouse [31]. Bovine serum albumin contains numerous amino acids, and the addition of albumin to a culture medium has a positive effect on embryonic development in vitro [32]. Here, the triglyceride level in the 120% NR group was significantly higher than that in the 80% and 100% NR groups (Table 2). The optimal ranges of triglyceride level, embryo quality, and embryo recovery rate were not directly investigated in cows. There was no difference in the number of transferrable embryos between the non-lactating and lactating cows [33]. The triglyceride level in the blood was higher in non-lactating Holstein cows (23.8 ± 0.9 mg/dL) than in lactating cows (17.2 ± 0.6 mg/dL). In the present study, cows in the 120% NR group had a high triglyceride level (34.8 ± 1.2 mg/dL) and produced a large number of transferable embryos. Therefore, we conclude that cows with a high triglyceride level in the blood can produce large number of transferable embryos after multiple superovulations with AI. In addition, triglycerides act as an energy source for bovine oocyte maturation in vitro. It has been suggested that the triglyceride levels are lower in mature oocytes than in immature ones [34].
In the present study, a high BUN level in the 120% NR group (11.6 ± 0.3 mg/dL) resulted in a large number of transferrable embryos compared low BUN levels in the 80% and 100% NR groups (9.5 ± 0.5 and 9.7 ± 0.4 mg/dL). In our previous study, the 120% NR group showed higher total cholesterol and BUN levels than the 80% NR group [35]. This result suggested that 120% NR of feed intake increase the total cholesterol and BUN levels in the blood compared with 80% NR of feed intake. We assumed that 120% NR of feed intake induced high production of proteins in liver and releasing of BUN compared with 80% NR group. The findings of a previous study support our result that high BUN levels (11–18 mg/dL) resulted in a higher number of transferable embryos (5.4 ± 1.9 embryos) than low BUN levels (<10 mg/dL) (3.2 ± 1.2 embryos) [36]. Thus, we believe that the 120% NR group cows secrete appropriate BUN levels for producing transferable embryos after multiple superovulations with AI. The NEFA level has been used as a predictor of NEB and fertility in cows. In the present study, the low NEFA level (195.1 ± 8.5 ЧEq/L) in the 120% NR group resulted in a higher number of transferrable embryos than the high NEFA levels in the 80% and 100% groups (318.5 ± 23.1 and 440.5 ± 18 ЧEq/L, respectively). Dairy cows with high levels of NEFA in the blood had lower fertility than those with low levels of NEFA [37]. In Hanwoo cattle, the pregnant cow group showed significantly lower NEFA levels in the blood than the non-pregnant cow group after AI (326.7 and 419.2 ЧEq/L in pregnant and non-pregnant cows, respectively; p < 0.05) [35]. Cell number and embryo quality decreased when oocytes were exposed to high levels of NEFA in vitro [38]. The AST and ALT levels have been used as an indicator of ketosis by oxidative stress and hepatic damage in dairy cows. However, the effects of normal AST and ALT levels in the donor cow on embryo recovery after multiple superovulations have not been clearly determined. In a previous study, cows with ketosis (hepatic damage) had higher AST and ALT levels than healthy cows (hepatic damage and healthy groups: 160.0 and 85.0 AST (U/L), 35.8 and 22.0 ALT (UL), respectively; p < 0.0001) [39]. In another study, the AST and ALT levels of healthy dairy cows was 43.0 and 37.0 UL, respectively [40]. The AST and ALT levels in the present study were lower than those reported [40]. Hence, different NR in Hanwoo cows did not affect hepatic conditions at any of the NR levels, irrespective of the AST and ALT levels.
In contrast to our result, a previous study showed that Holstein heifers with 2.75 BCS produced more excellent grade embryos than those with 3.0–3.25 BCS [41]. Zebu cattle with a mean BCS of 2.5–3.0 produced more transferable embryos (2.0 embryos) than those with a mean BCS of 4.0–4.5 (0.0) after multiple superovulations with AI [42]. In the present study, cows with a mean BCS of 3.7 produced more transferrable embryos than those with a BCS of 2.6 or 3.2 (Table 4). We believe that the feeding system and circumference affect the number of transferable embryos depending on the cow species. There was no significant difference in the estrus cycle between the 80%, 100%, and 120% NR groups (Table 4). However, the estrus cycle in the 80% NR group was marginally longer (24.6 ± 2.8 days) than that in the 100% and 120% NR groups (20.8 ± 0.2 and 21.2 ± 0.5 days, respectively). This indicates the cows in the 80% NR group have a higher NEB. We postulated that the NEB in the 80% NR group can be elucidated using the highest NEFA level, unlike that in the 100% and 120% NR groups. Therefore, the NEB status in the 80% NR group might have extend the estrus cycles [43].
Our study provides the appropriate feeding level for Hanwoo cows for embryo recovery with multiple superovulations and AI. Blood metabolic tests revealed that Hanwoo cows with 120% NR had maintained the NEB and produced more transferable embryos. We suggest that 120% NR and BCS 3.7 are suitable for embryo recovery after multiple superovulations with AI. Therefore, 120% NR is recommended to produce a large number of transferable, high-quality embryos in Hanwoo cows by multiple superovulations with AI when fed a commercial concentrate and hay (rice straw).