INTRODUCTION
Many factors, such as infection and stress, can induce immunosuppression, resulting in retardation of growth performance, susceptibility to disease, and mortality [1,2]. Immunosuppression in livestock is thus directly related to economic loss. Alleviating adverse immune reactions is necessary for successful livestock production [1,2] Cyclophosphamide (CTX), an alkylating agent, interferes with DNA replication and RNA transcription and is used in cancer chemotherapy [3]. The CTX directly activates immune responses in hematopoietic stem cells and dendritic cells [4,5]. It affects the proliferation of lymphocytes and is extensively used for the treatment of certain tumors, cancers, and autoimmune disorders. Dose-dependent administration of CTX also enhances immune responses. Despite its chemotherapeutic activity, many studies report that CTX treatment induces several adverse effects, including hemorrhagic cystitis in bone marrow transplantation, genotoxicity in human lymphocytes, cardiac toxicity, and pulmonary fibrosis [6–9]. Nevertheless, CTX is widely used as an anticancer agent because it suppresses the immune system [10].
N-acetylcysteine (NAC) is an antioxidant that exerts its effects by directly scavenging reactive oxygen species to protect cells from oxidative damage and by stimulating glutathione synthesis [11,12]. Furthermore, NAC modulates oxidative stress, liver injury, and inflammation of the small intestine [13,14].
The NAC alleviates CTX-induced gonadotoxicity and genotoxicity in experimental animals such as mice and rats [15,16]. However, the effects of dietary supplementation with NAC on CTX-induced immune reactions, liver injury, and oxidative stress have not been investigated in miniature pigs. Miniature pigs are a valuable animal model to investigate the effects of therapeutic agents because of their similarity to humans in terms of anatomical and physiological features and sensitivity to drugs compared with other non-rodent species [17]. The present study thus used miniature pigs to evaluate the effects of dietary supplementation with NAC on CTX-induced immune reactions, including cytokine production and expression of immune-related genes; liver injury; and oxidative stress.
MATERIALS AND METHODS
Miniature pigs (n = 15) [MK strain; (Duroc × Yorkshire) × (Pot Valley × Berkshire) × Yucatan] with an average initial body weight of 22.3 ± 0.32 kg were used to evaluate the effects of dietary supplementation with NAC on effects caused by CTX injection in a 4-week trial. Each animal pen was equipped with a one-sided, stainless steel self-feeder and a nipple drinker that allowed access to feed and water ad libitum. All diets were formulated to meet or exceed NRC nutrition requirements [18]. The corn-soybean meal based dietary treatments were as follows: T1, control diet + saline injection; T2, control diet + CTX injection; and T3, control diet + 0.5% NAC and CTX injection. For CTX treatment, pigs were injected intraperitoneally with 0.01% CTX (50 mg/kg) or saline solution after a 2-week feeding trial.
Blood samples were collected into non-heparinized and K3-EDTA vacuum tubes (Becton Dickinson Vacutainer Systems) to determine numbers of immune cells, including leukocytes, lymphocytes, and monocytes, after 3 and 4 weeks. Immune cell counts were determined using an automatic blood analyzer (ADVIA120; Bayer, Leverkusen, Germany). ELISA was used to quantify serum concentrations of tumor necrosis factor (TNF)-α (R&D Systems, Minneapolis, MN, USA), alanine transaminase (ALT; Sigma-Aldrich, St. Louis, MO, USA), aspartate aminotransferase (AST; Sigma-Aldrich, St. Louis, MO, USA), superoxide dismutase (SOD; Cohesion Biosciences, USA), malondialdehyde (MDA; Abcam, Cambridge, UK), and glutathione peroxidase (GPx; Cayman Chemical, Ann Arbor, MI, USA).
Blood samples were collected 6 h after injection, and PBMCs were prepared as previously described [19]. Briefly, blood samples were diluted with an equal volume of balanced salt solution and were mixed with Histopaque solution. After centrifugation at 400×g for 35 min at room temperature, PBMCs were collected from the Histopaque solution-plasma interface.
TRIzol reagent (Invitrogen) was used for RNA extraction from PBMCs. For complementary DNA synthesis from total RNA (100 μg), a Maxima First Strand cDNA Synthesis Kit (Life Technologies, Carlsbad, CA, USA) was used. Primer sets were designed using Primer3 software (http://frodo.wi.mit.edu/), and the primer sequences are shown in Table 1. Quantitative real-time PCR was performed using a 7500 Fast Real-Time PCR System (Applied Biosystems, Waltham, MA, USA) with the following conditions: 94°C for 3 min, followed by 40 cycles at 94°C for 30 s, 59°C–61°C for 30 s, and 72°C for 30 s. Glyceraldehyde 3-phosphate dehydrogenase (GAPDH) was used as an endogenous control, and quantitative real-time PCR data were calculated using the 2−ΔΔCt method, where ΔΔCt = [threshold cycles (Ct) of the target gene − Ct of GAPDH] treatment − [Ct of the target gene − Ct of GAPDH] [20].
Data were statistically analyzed using analysis of variance and the general linear model (GLM) procedure of statistical analysis software (SAS) program (SAS Institute, Cary, NC, USA), with a completely randomized design. Data are presented as means and standard error of the means. Statistical significance of differences between treatments for immune cells, TNF-α production, inflammatory cytokine-related gene expression, liver function parameters, and oxidative stress markers was analyzed using the GLM in SAS. Duncan’s multiple range test was used as a post hoc test to analyze differences between means, and a p-value of < 0.05 was considered statistically significant.
RESULTS
Treatment with CTX decreased immune cell numbers at 3 and 4 weeks compared with that in the control group [T1 group; Fig. 1]. Compared with CTX alone, dietary supplementation with NAC increased the immune cell numbers at 3 and 4 weeks. The effect of dietary supplementation with NAC on TNF-α production after CTX treatment is shown in Fig. 2. CTX treatment increased the levels of TNF-α at 3 and 4 weeks compared with that in the T1 group. Compared with CTX alone (T2 group), dietary supplementation with NAC decreased the levels of TNF-α at 3 weeks.
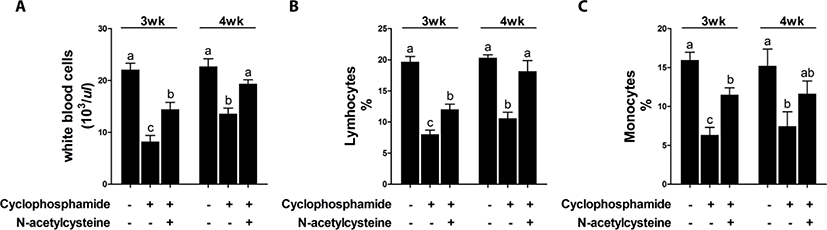
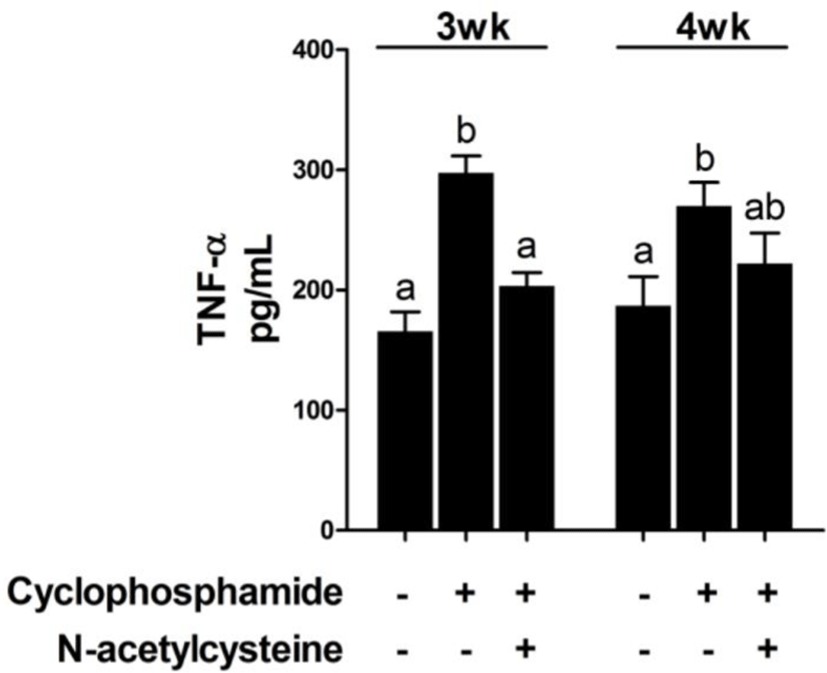
CTX increased NF-κB, IFN-γ, TNF-α, IL-8, and IL-1beta1 expression and decreased IL-10 and IL-4 expression compared with the results in the control group (Fig. 3). Dietary supplementation with NAC decreased NF-κB, IFN-γ, TNF-α, and IL-8 expression and increased IL-10 expression.
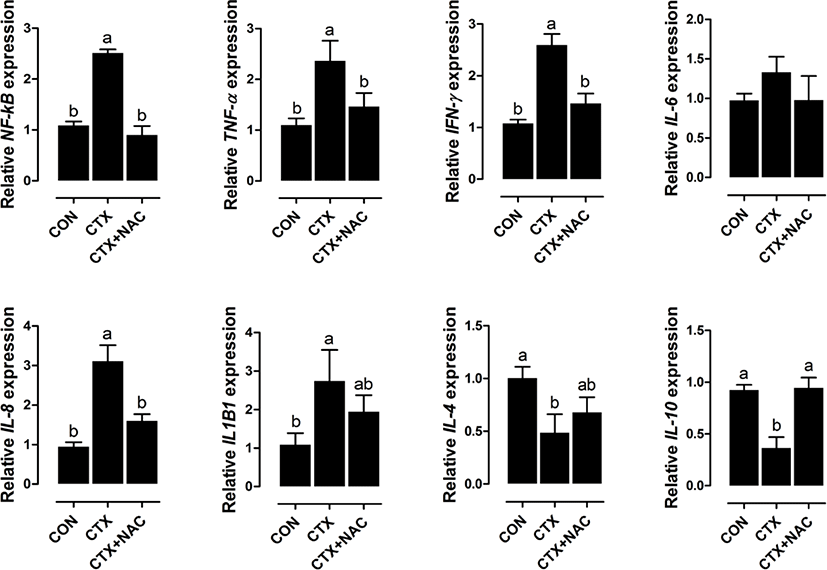
CTX increased the serum AST and ALT levels at 3 and 4 weeks compared with the results in the control group (Fig. 4). Dietary supplementation of NAC after CTX challenge decreased the serum AST and ALT levels.
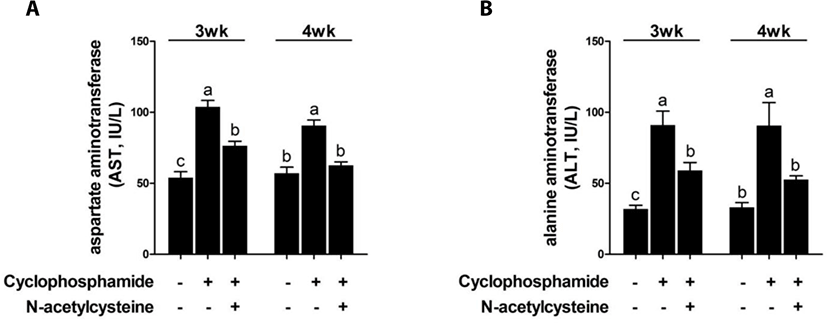
CTX challenge decreased the serum SOD and GPx levels and increased the serum MDA levels at 3 and 4 weeks compared with the results in the control group (Fig. 5). Dietary supplementation with NAC following treatment with CTX increased the serum SOD and GPx levels and decreased the serum MDA levels at 3 and 4 weeks.
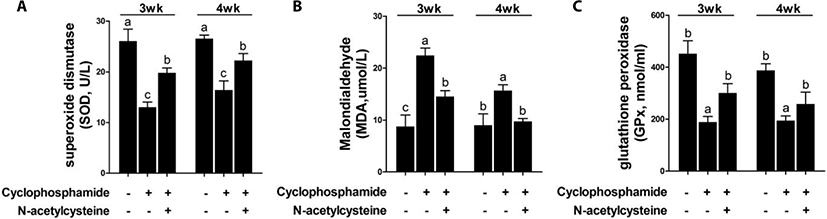
DISCUSSION
CTX is a commercially available agent used to treat immune disorders after organ transplantation [1]. It is widely accepted that CTX-induced immunosuppression reduces cellular immunity, oxidative stress, as well as daily weight gain and feed intake in livestock [1,21]. NAC, traditionally used as an antioxidant, modulates oxidative stress-mediated liver injury and inflammation of the small intestine [13,14]. Miniature pigs as an experimental model for humans have advantages of similar sensitivity to drugs compared with other non-rodent species and similar anatomical and physiological features [17,22,23]. Dietary supplementation with NAC increased immune cell numbers and decreased the level of TNF-α production compared with CTX treatment alone. Consistent with our findings, previous studies reported that other dietary agents also modulate lymphocytes, IL-2, and IFN-γ in CTX-induced immunosuppressed pigs [21]. The present study used PBMCs, which comprise lymphocytes, monocytes, and macrophages, as representative immune cells. In molecular nutrition and nutrigenomics, these cells are good targets because they directly reflect gene expression levels following chemical treatments [24].
The immune system is divided into an innate immune response that consists of cellular defenses against invading pathogens and an adaptive immune response that consists of memory cells for the elimination of pathogens [25]. In both innate and adaptive immune responses, NF-κB is activated by toll-like receptors and directly binds to upstream sequences of inflammatory cytokines, including TNF-α, a marker of immune system activation [26]. The present study examined the effects of dietary supplementation with NAC on serum cytokines in CTX-induced immunosuppressed pigs. Dietary supplementation with NAC decreased TNF-α production, decreased NF-κB, IFN-γ, TNF-α, and IL-8 expression and increased IL-10 expression in PBMCs from CTX-induced pigs. Thus, NAC may influence immune-related gene expression via the inactivation of NF-κB after CTX-induced immunosuppression in miniature pigs.
The present study revealed that dietary NAC decreased levels of ALT and AST as markers of liver function and modulated levels of SOD, MDA, and GPx as markers of oxidative stress after CTX-induced immunosuppression. NAC is well known to alleviate oxidative stress, liver toxicity, and heart disorders [27]. In previous reports, dietary supplementation with NAC moderated liver injury, anti-oxidative capacity, and energy metabolism [14,28]. The present study used CTX as an inducer of liver injury and oxidative stress. CTX is widely used to induce hepatotoxicity and oxidative stress in the liver, with a concomitant increase in serum levels of liver markers, including ALT and AST [29,30]. Abnormal increases in ALT and AST levels indicate CTX-induced cellular damage to hepatic cells [29,30]. NAC might thus be used as a therapeutic agent for modulation of hepatic injury and oxidative stress after CTX-induced immunosuppression in miniature pigs.
In conclusion, dietary supplementation with NAC modulates immune cell populations and TNF-α levels in serum after CTX-induced immunosuppression in miniature pigs. The present study also confirms that dietary NAC decreases the expression of NF-κB, IFN-γ, TNF-α, and IL-8 and increases IL-10 expression in PBMCs. Moreover, dietary NAC decreases serum levels of the liver function markers ALT and AST and modulates levels of the oxidative stress markers SOD, MDA, and GPx. These results suggests that NAC can be used as a therapeutic agent to modulate immune reaction, liver injury, and oxidative stress in livestock.