INTRODUCTION
Methionine (Met) is an essential amino acid (AA) that is crucial in retaining body protein and promoting feather growth. In animal diets, particularly in poultry, Met is often the first-limiting AA when using corn- and soybean meal (SBM)-based diets [1]. Furthermore, Met is involved in various metabolic functions, including protein synthesis, immune response, acting as a methyl donor during DNA replication, and aiding in cysteine (Cys) production through degradation. Dietary Met can lead to the de novo formation of Cys, rendering Cys an indispensable AA. This makes the total sulfur AA (TSAA; Met + Cys) a common representation for Met requirements. A dietary Cys deficiency may lead to overestimating the Met requirement in broilers. This is because dietary Met is utilized to meet the demand for Cys in case of insufficient dietary Cys. Consequently, converting Met into Cys to replenish insufficient levels may reduce the overall proportion of Met utilization. Commercial diets are typically formulated based on TSAA to ensure adequate Met and Cys levels [2]. Understanding the specific growth response to dietary digestible Met concentrations in broilers is crucial. This information is essential for accurately meeting the Met requirements of broilers to attain production goals such as optimal and maximum breast meat yields. By optimizing Met levels, productivity can be enhanced, and nutrient excretion into the environment reduced, benefiting both the industry and environment. Numerous studies have concentrated on establishing TSAA requirements [3-7], as well as the Met-to-Cys ratio [2]; however, only a few have specifically addressed Met requirements [8-11]. Furthermore, decades of intensive selective breeding have increased voluntary daily feed intake and enhanced digestive capacity, leading to rapid growth rates in modern broilers [12]. Therefore, continued research is essential to accurately determine Met requirements and appropriately adjust dietary Met concentrations to align with the genetic improvements in broilers. The Met requirements recommended by the National Research Council [13] and Rostagno et al. [14] differ from those estimated using empirical methods to determine Met or TSAA requirements. Estimating Met requirements for broilers is complex due to several interacting factors, including estimation methodology [15], dietary Met sources [16], and animal-related factors, such as age [6,7], intestinal growth [17], and onset of feathering [3]. Moreover, the individual AA needs for broilers may vary depending on dietary nutrient concentrations, body size, and growth stage [18]. Additionally, the critical role of feather development has been overlooked, despite its importance in protecting birds from environmental risks and conserving heat [19]. Feather protein synthesis demands a substantial amount of Cys [20,21] and constitutes a significant portion of the AA composition [3,22]. Therefore, the feather growth rate might impact the necessary Met intake for optimal growth performance in broilers. This study aims to determine Met requirements based on male broiler growth during the grower phase and variations in Met concentrations in feathers using the ideal AA concept with diets containing different dietary standardized ileal digestible (SID) Met concentrations.
MATERIALS AND METHODS
All the experimental procedures in this study were reviewed and approved by the Institutional Animal Care and Use Committee of Kyungpook National University (KNU2021-0217).
A total of 192 22-day-old male broiler chickens (Ross 308) were used in this study. The birds were allocated to six treatments in a randomized complete block design. To reduce the initial body weight (BW) impact, a set of experimental treatments was categorized into groups (blocks). The initial BW was regarded as a blocking factor. Each treatment having eight replicate cages containing four birds per cage. The experimental diets were provided in mash form, and both water and feeds were offered ad libitum. The environment was carefully controlled with continuous light and temperature. The birds were housed in wire-floored cages, measuring 60 cm × 60 cm × 60 cm, in an environmentally controlled room. Following the Ross 308 broiler recommendations [23], the temperature in the room was initially set at 33°C for the first three days and then gradually decreased by 3°C per week until it reached 24°C by day 29 and relative humidity was set at 65% for the first three days and gradually decreased to 45% at the end of the experiment. This temperature and relative humidity were monitored and maintained until the end of the experiment.
Six experimental diets were formulated based on the corn-SBM-based diet, with varying SID Met concentrations ranging from 0.40% to 0.65%. These diets were designed to contain graded levels of dietary SID Met while maintaining sufficient Cys at 0.47% (Table 1). The ideal digestible AA ratios relative to lysine concentrations, except for histidine and phenylalanine, for the grower phase (day 15 to 35 of age) were adopted from Hoehler et al. [24]. Relative histidine and phenylalanine ratios to lysine were obtained from Rostagno et al. [14]. The SID of AA in corn and SBM was determined using equations described by Kong and Adeola [25]. Moreover, the SID AA in corn and SBM for 25-day-old Ross 308 male broilers were derived from a previous study [26]. All indispensable AA, except for Met, were included at 110% of recommended levels to prevent deficiencies; other AA ratios; the recommended lysine level used in the present study was obtained from our previous study [27], and the value was determined based on the weight gain of 21 to 28 days old male broilers using a broken-line model. The dietary Met in each experimental diet served as the first-limiting AA. Analyzed AA concentrations in the experimental diets were close to the calculated values (Table 2).
1) Supplies the following per kilogram of diet: vitamin A, 24,000 IU; vitamin D3, 8,000 IU; vitamin E, 160 mg/kg; vitamin K3, 8 mg/kg; vitamin B1, 8 mg/kg; vitamin B2, 20 mg/kg; vitamin B6, 12 mg/kg; pantothenic acid, 40 mg/kg; folic acid, 4 mg/kg; niacin, 12 mg/kg.
During the experimental period, daily mortality for each cage was recorded. Individual BW, feed supply, and leftovers per cage were also monitored to determine body weight gain (BWG), feed intake, and gain-to-feed ratio (G:F) at the beginning and end of the experiment. Four birds from each treatment group were selected based on their average BW for whole-body feather sampling. The AA compositions in the broilers’ feathers were determined using 29-day-old birds. Feathers were removed from each carcass by dry plucking.
Dry matter and AA compositions in the experimental diets were analyzed. The samples were ground using laboratory mill (CT293 Cyclotec, Foss, Hillerød, Denmark) for chemical analysis. Test feed ingredients, commercial diet, and experimental diet samples were dried at 135°C for 2 hours according to the Association of Official Analytical Chemists (AOAC) International method 930.15. Ccorn, SBM, commercial diet, and experimental diet samples were analyzed for AA using the AOAC International methods 994.12 and 988.15 [28]. Similarly, the collected feather samples were ground and analyzed for dry matter and AA composition using AOAC International methods 930.15, 994.12, and 988.15 [28].
Data for BWG and G:F were analyzed using the MIXED procedure in SAS (SAS Institute, Cary, NC, USA). The SID Lys requirements were estimated using one-slope broken-line and quadratic-line models employing nonlinear regression analysis in SAS, based on the study by Robbins et al. [29]. Each experimental diet was treated as a fixed effect, while the blocks with replicates based on initial BW per cage were considered a random effect. If the random effect was Final statistic model Each cage served as an experimental unit, and statistical significance was set at p = 0.05.
RESULTS
This experiment was conducted to determine the SID Met requirements of broilers in the grower phase (22 to 29 days of age). Only one bird was dead during the experimental period and feed intake of dead birds was calculated and eliminated from the final dataset.
Graded levels of dietary SID Met concentrations resulted in significant quadratic effects (p < 0.01) on final BW, BWG, and feed intake (Table 3). However, there was no significant differences in G:F in response to dietary SID Met concentrations. Moreover, feather weight and relative feather compositions (% of BW) were not influenced by dietary SID Met concentrations. The data in Table 4 indicate that dietary SID Met concentrations had no significant influence on weight and AA composition in feathers (wing + body). The SID Met requirements for BWG and feed intake, which were determined using a linear broken-line model, were 0.45% and 0.46%, respectively. The estimated SID Met requirement for both BWG and feed intake, using a quadratic-line model, was 0.55%. In addition, the calculated SID Met 95% of the upper asymptotic value of quadratic-line model for BWG and feed intake was 0.52% (Figs. 1 and 2).
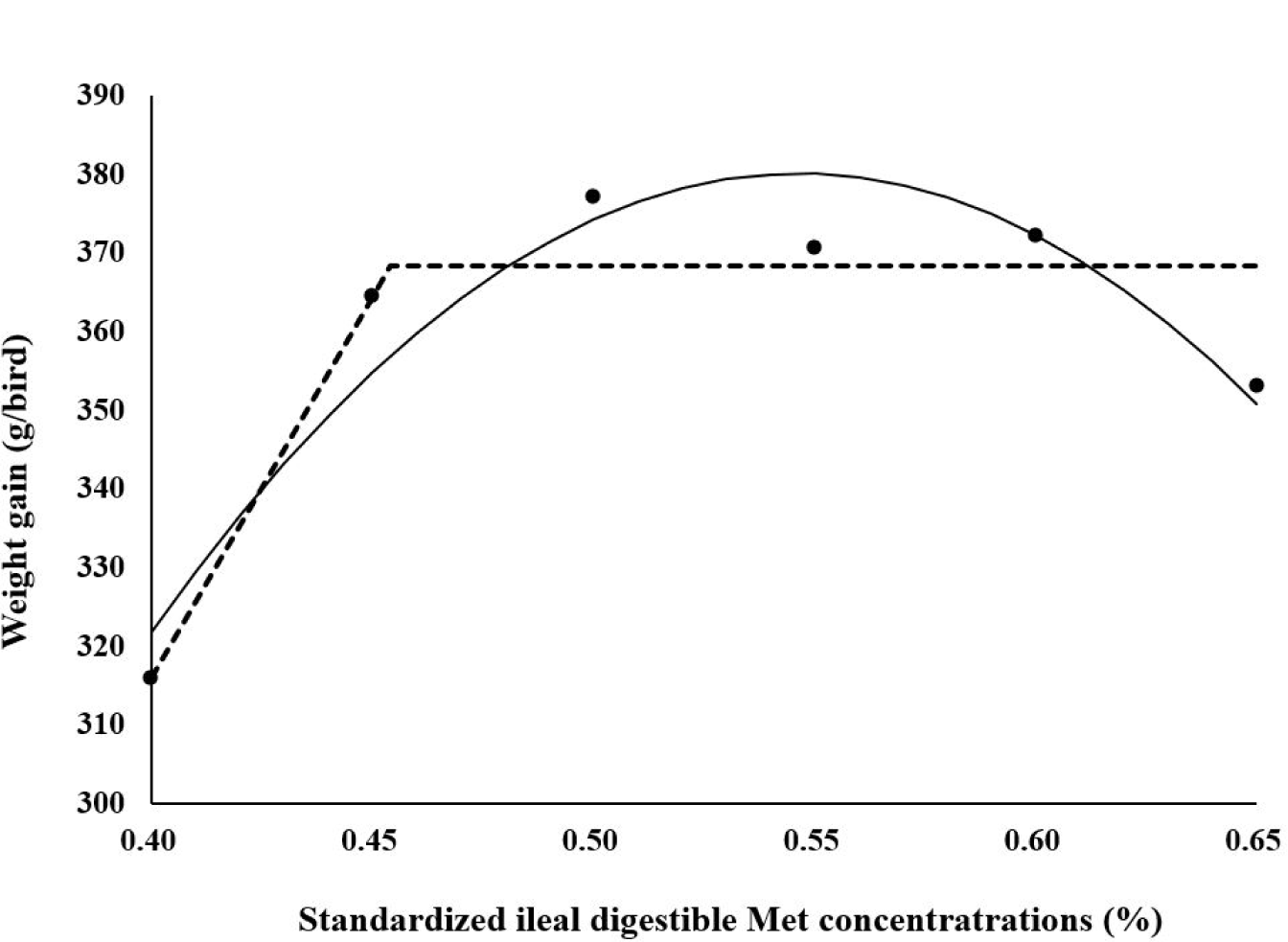
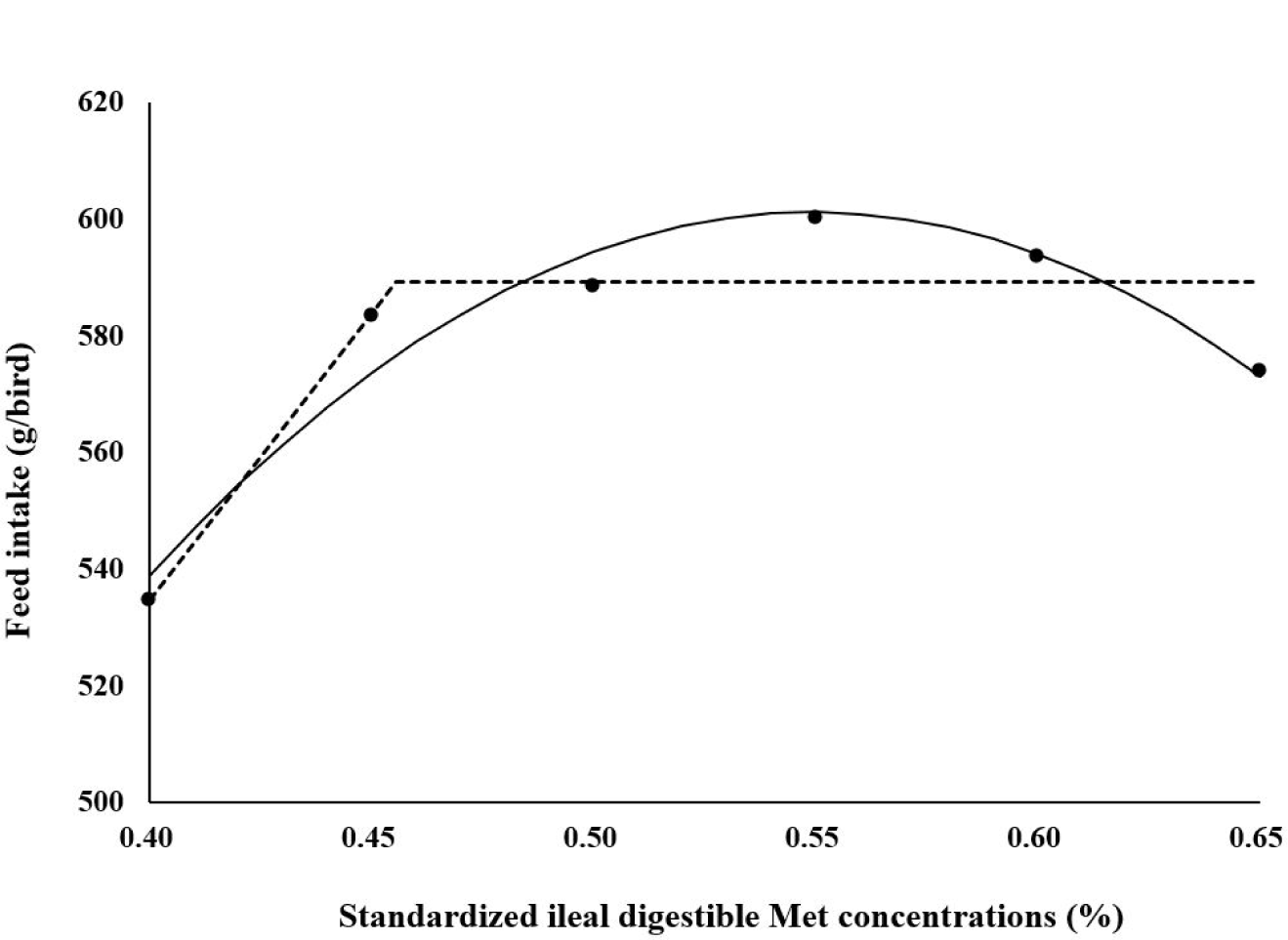
DISCUSSION
Various regression models can be used to determine the AA requirement. The selection of a regression model is based on plotting the response of birds against dietary AA concentrations. A linear broken-line model is appropriate if there is a plateau response after a certain point. On the other hand, if there is an increase or decrease in responses after the breakpoint, a quadratic-line model is suitable to determine the AA requirement or AA toxicity. Baker [30] suggested that estimated AA requirements from a linear broken-line model represent the maximum response with a minimum amount of dietary AA, regardless of economic considerations and safety margins. Moreover, the fitted broken-line model provides an objective value rather than a subjective one. However, if there is an increase or decrease in responses after the breakpoint, a quadratic-line model is suitable to determine the AA requirement or AA toxicity. The estimated AA requirement does not yield objective breakpoints. Baker [30] proposed that one must subjectively select, for example, 90% of the upper asymptote value of the quadratic line. Therefore, this study provided the SID Met requirement based on the quadratic-line model using the 95% upper asymptote value.
The birds in this study had inadequate growth compared to the recommended growth rate specified in the Ross 308 performance objectives [23]. Various factors, such as animal and environmental conditions, can influence bird growth rate. It is challenging to explain why the birds did not reach their target growth potential. However, one potential contributing factor could be the feed forms used. Numerous studies have shown that different feed forms impact broiler chickens’ growth performance and feed efficiency. It is widely recognized that feeding pellets, rather than mash, enhance broiler growth rate and increase feed intake [31,32]. In this study, the birds were fed crumble commercial feed for 21 days, then after eight days, feeding mash-form the experimental diet. In terms of BW, male broilers in this study at three weeks of age yielded results similar to previous studies that aimed to determine the metabolic requirement of broilers [10,20]. The lower BW observed in the birds used in the present study after three weeks of age, compared to the performance potential of the Ross 308 breed, could be attributed to the transition from a crumble commercial to a mash-form experimental diet.
When assessing the AA requirements for broilers, growth response indicators such as BWG and feed efficiency are traditionally employed [33]. In this study, the impact of dietary SID Met concentrations on feed efficiency was not significant. However, both BWG and feed intake showed significant results. Feed intake is the main factor driving the growth rate of broilers. Therefore, the estimated SID Met requirement was determined based on both the BWG and feed intake of birds. The estimated Met requireme nts for 22 to 29-day-old male broilers in the present study were within the reported range of values, ranging from 0.46% for 21 to 42-day-old [20] to 0.59% for 21 to 42-day-old [10]. The optimal dietary Met requirement (using linear-broken line model) based on weight gain (0.45%) and feed intake (0.46%), as estimated in this study, was similar to the estimation for weight gain and G:F (0.46%) provided Moran [34]. Although growth response and feed intake of birds increased quadratically, but the G:F is not influenced by dietary SID Met concentration from 0.40% to 0.65%. Therefore, in the present study, SID Met requirements for 22 to 29-day old male broilers were determined based on the weight gain and feed intake; and linear-broken and quadratic-line models were employed to determine the SID Met requirements. The estimated Met requirement was lower than the value recommended by the NRC [13], which was 0.38% for three to six-week-old broilers, but similar to the value of 0.46% for 22 to 33-day-old broilers reported by Rostagno et al. [14].
Feather AA composition may change depending on dietary Met concentrations. A deficiency of dietary Cys can induce the conversion of Met into Cys; therefore, dietary L-Cys supplement replaces Cys and increases the proportion of digestible Met concentrations used for protein synthesis to increase body mass [2]. Moreover, the contribution of TSAA in the integument is greater than that in muscle tissue, and TSAA concentration is a major factor influencing the synthesis of feather keratin [3]. Consequently, an insufficiency in TSAA can lead to abnormal feathering, potentially impacting the nutritional requirement for growth due to its effects on the AA pool. Kalinowski et al. [20] evaluated the Met requirement for 0-to-3-week-old broilers by increasing DL-Met concentrations from 0.35% to 0.50% while maintaining a constant Cys level (0.50%) but failed to elicit a weight gain response. Nevertheless, they observed improved FCR and N retention, irrespective of the birds’ feathering rate. As a result, they concluded that the Met requirement is not influenced by the feathering rate, establishing it at 0.46% based on N retention using the quadratic model. However, they confirmed variations in Cys to TSAA) requirement depending on strains with genetically distinct feathering rates, such as 44% and 47% in slow- and fast-feathering birds, respectively. Therefore, the feathering degree may confound results, as mature broilers require higher N retention and AA for feathering than younger counterparts [20]. Fisher et al. [35] highlighted how dietary specifications could impact feather growth and AA composition. In contrast, Conde-Aguilera et al. [36] observed no differences in feather AA composition and retained Met and Cys contents in response to dietary TSAA concentrations, which conform to our results. In addition, the AA composition in feathers in the present study was similar to the previous studies [37-39]. In this context, birds may be more sensitive to dietary AA depend on the contribution on feather synthesis compared to the dietary Met, given its relatively higher proportion (7.33%) deposited in feather compared to Met (0.57%) [40].
Feather mass in birds corresponds to the excreted N within the consumed AA. Vargas et al. [41] reported that feather weight increased from 1.4 g to 151.3 g as BW increased from 44 g to 4,188 g while maintaining a constant relative weight (3.43%) from 1 to 56 days. Feather weight and deposited AA were measured to verify whether dietary Met contributes to feather AA composition. Similar outcomes were observed in the present study, where dietary SID Met concentrations did not impact feather weight (g), and relative feather weight (% of BW) averaged 1.91% at an average BW of 1,103 g at 29 days old. This result suggests that the lowest dietary SID Met levels (0.40%) are not limiting for feather protein synthesis. However, the relative feather weight to BW (1.91% vs. 3.43%) from the present study was lower than in Vargas et al. [41] with a similar BW. Differences between the studies could be attributed to the shorter feeding duration in this study (seven days) and different genetic backgrounds.
Numerous factors influence feather growth and AA composition, such as dietary specifications [3,35], limiting AA [42], and bird age [34,43,44]. The absence of differences in feather AA composition based on dietary SID Met concentrations in the current study may be attributed to adequate dietary AA, except for Met, which was supplied to meet broiler requirements according to the ideal AA ratio. This is because the experimental diets were formulated to increase Met concentrations from deficient to excess levels. The limitation in AA utilization is determined by the amount of dietary second-limiting AA as the dietary first-limiting AA is increased [45]. Therefore, the results of the present study suggest that balanced dietary AA supplementation is crucial in terms of AA concentrations and supporting protein accretion for growth and feather conditions.
In conclusion, the dietary SID Met concentration ranged from 0.40% to 0.65%, and the constant dietary protein ratio did not affect the feather AA composition of 29-days old male broilers. The estiamted SID Met requirements for 22–29 days old broilers vary depending on the statistical methods. The different SID Met requirements for broilers can be used for various target groups of animals. The optimum (brokne-line model) SID Met requirements based on the weight gain and feed intake were estimated to be 0.45% and 0.46%, respectively. The estimated maximum (quadratic-line model) SID Met requirements for BWG and feed intake was 0.55%. In addition, the calculated SID Met requirements for BWG and feed intake, which is 95% asymptotic value of quadratic-line model was 0.52%.