INTRODUCTION
The processing operations of fish and aquatic products generate a considerable amount of rest raw materials called by-products. Fish by-products are regularly converted into fish meals and consumed as a protein source in aquatic farming, poultry, and livestock nutrition. Mincing, cooking, pressing, drying, and milling, which are applied in traditional fish-meal production, are overpriced and complex procedures, and the high temperature utilized for processing fish meal could impair the digestibility of the final product [1,2]. Therefore, during the past decades, a large quantity of scientific documents have been published regarding the properties and potential applications of fish proteins recovered from fish by-products for animal nutrition [1,3,4].
Fish protein hydrolysate (FPH) is a functional product developed from the whole fish or fish by-products using the protein hydrolysation method in which fish proteins are broken into smaller parts, namely, peptides and amino acids [5]. FPH is available in liquid, paste, and dried forms in the market. Liquid FPH contains up to 90% of moisture and is highly unstable for long-time storage; therefore, its transportation is not economic. Thus, the dried form of this product is preferred because of less-challenging storage, longer shelf-life, and easier transport. On the other hand, one of the problems of dried-form FPH production is the separation of a considerable amount of water from liquid FPH which is a tough and expensive task [6,7].
Drying of hydrolyzed proteins is a serious challenge in the operation of these products [2]. The co-drying of hydrolyzed fish protein with dry agricultural by-products is an innovation in the use of agricultural and fishery by-products for animal feed. Several researchers have used this method on a laboratory scale to co-dry liquid hydrolyzed protein and use the product in animal feed. This method can be economical if produced on a semi-industrial or industrial scale [8-11]. Thus, this offers a profitable and novel method for converting the by-products of fish into useful protein-hydrolysate materials in order to feed birds, aquaculture, and other livestock [12].
Meanwhile, dependence on soybean imports from major producer countries for animal farming including broilers husbandry, has led many livestock producers to replace local protein products with soy protein. The use of local protein products, including fish protein ingredients from fishery by-products, is an alternative to partial substitution of soybean meal in livestock diet [13]. Co-drying of FPH to develop new sources of value-added animal protein as a feed supplement is a new approach for feeding animals with local agricultural and fisheries by-products [2].
Although some studies have reported the positive influence of FPH on the growth performance of broiler chickens [14-16], but limited applied studies have addressed the application and evaluation of co-dried fish protein hydrolysate (CFPH), produced in a feasible method, in the animal nutrition. Further, more data are required to be published about the immunological and microbiological effects of these products on commercial poultry species. Accordingly, this research focused on the production of a novel protein supplement based on FPH and its effects on the performance, intestinal microbiology, and cellular immunity of broiler chickens. The results of this study may be employed to valorize the application of a new generation of FPH in the animal feeding industry and may help to reduce the amount of soy bean meal in diets.
MATERIALS AND METHODS
Experimental procedures relating to chicken rearing and care in the current study were reviewed and approved by the Animal Ethics Board of the Animal Science Research Institute of Iran (Certificate No. 47-13-13-083-990566- 22 September 2020).
The CFPH was developed from whole Kilka fish (Clupeonella spp.) using an enzymatic process on a pilot scale. The alcalase, a proteolytic enzyme, was used to produce FPH [6]. The whole fish protein solution (without phase separation) was stabilized by formic acid (CH2O) to obtain a pH value of 4.5. After stabilizing the pH, a 50% fish protein solution, 20% rice bran, 29% defatted sesame seeds (Sesamum indicum L.), and 1% feed grade calcium bentonite clay were applied to prepare a semidry material. The semidry FPH was co-dried in a vacuum oven-dryer at 60°C for 4 h. The dried samples were milled to pass through a 0.4-mm sieve and stored at ambient temperature (25°C) until included in the diets.
The dry matter, ash, crude fiber, ether extract, crude protein, calcium, available phosphorus, pH, theAA compositions and total carbohydrate of CFPH were determined according to AOAC [17]. The total volatile basic nitrogen (TVB-N) content was estimated via the method described by Goulas and Kontominas [18]. The fatty acid (FA) compositions of CFPH were analyzed by gas chromatography (GC, 6890 series, Agilent Technologies, Wilmington, DE, USA) following the procedure described in previous research [19]. The chemical compositions of the CFPH are provided in Table 1.
The project was conducted at the Research Poultry House affiliated with the Agricultural Research, Education, and Extension Organization (AREEO) of Iran (Karaj, Alborz, Iran). Five hundred one-day-old male broiler chickens (Ross 308) were acquired from an industrial hatchery (with an initial body weight [BW] of 40 ± 0.5 g) and randomly distributed in 20 floor pens. The initial temperature of the farm was kept at 33 ± 2°C and regularly declined (2.4°C weekly) to reach a persistent temperature of 21–23°C at the age of 28 days. Throughout the experiment, the lighting regime and relative humidity were maintained in 23:1 h of light/darkness and 50%–60%, respectively. The access of chickens to water and feed was unlimited during the experiment.
Before formulating diets, the chemical composition of the main feed ingredients, including maize and soybean meal, was analyzed according to the AOAC procedures [17], and the data were applied to formulate the experimental diets. Four levels of CFPH (0% as the control, 2.5%, 5%, and 7.5%) were included in the dietary treatments (Table 2). All diets contained a similar amount of protein and energy and were formulated based on Ross 308 recommendations [20].
1) To provide vitamins and minerals per kilogram of diet: vitamin A, 17500 IU; vitamin E, 35 mg; vitamin D3,3900 IU; vitamin K3, 4.8 mg; riboflavin, 7.4 mg; vitamin B12, 1.7 mg; niacin, 56 mg; thiamine, 2.96 mg; biotin, 0.17 mg; pyridoxine, 4.55 mg; folic acid, 1.8 mg; ethoxyquin, 0.124 mg; pantothenic acid, 17.7 mg; choline chloride, 486.5 mg; cyanocobalamin, 0.025 mg; Zn-sulfate, 83 mg; Fe-sulfate, 39.5 mg; iodine (calcium iodate), 1.25 mg; Cu-sulfate, 19 mg; Mn-sulfate, 158 mg; selenium (sodium selenite), 0.30 mg.
Performance variables, including feed intake (FI) and BW, were recorded periodically. The difference between given and residual feed was considered as FI. Mortality was daily recorded, and data related to average daily gain (ADG), average daily feed intake (ADFI), and feed conversion ratio (FCR) were corrected based on this parameter. At the end of the experiment (42 days of age), 30 birds from each treatment (six birds/pen which had close weight to the average of the pen) were chosen, and then weighed and slaughtered after 4 h of fasting. Chickens were slaughtered based on the European Union legislation on the protection of animals used for scientific purposes (Directive 2010/63/EU). Next, the slaughtered birds were plucked, and visceral organs were excised to determine the relative weight of the carcass and organs. At 42 days of age, the European broiler index (EBI) of broiler chicks (including the sacrificed birds) was calculated by the following formula [21]:
Different parts of the small intestine, including jejunum, duodenum, and ileum, were sectioned, intestinal digesta was removed, and intestinal segments were washed in neutral-buffered saline and blotted dry on paper towels. Then, the relative weight and length of each segment were calculated by dividing the weight and length of each section by live BW, respectively [22].
At 30 days of age, the cutaneous basophilic hypersensitivity (CBH) reaction to phytohemagglutinin P (PHA-P, Sigma Chemical, St. Louis, MO, USA) was assessed by intra-dermally injection of 100 μg of PHA-P (dissolved in a 0.1 mL of sterile saline) into the toe web of the left foot of three birds from each pen based on the method described by previous researchers [23]. The difference between skin thickness before and 12 or 24 h after injection was considered as the CBH reaction to PHA-P.
Moreover, at 30 days of age, the DNCB reaction was determined by calculating the difference between skin thickness on the right side of the lateral abdomen in three birds per pen (other than those treated with PHA-P) before and 24 or 48 h after the challenge with 0.1 mL of the prepared DNCB (2,4-dinitrochlorobenzene, Merck, Darmstadt, Germany) solution [24]. The average of the three repeat measurements for each bird was used for the analysis.
Ileal samples were subjected to microbial analyses based on the previously published method [25]. Concisely, each sample of the ileal content (approximately 1 g) was homogenized, followed by serial dilutions. A 10 μL sample of each serial dilution was inoculated using bacteria-specific agars, including MacConkey agar (MC agar, Neogen, Lansing, MI, USA) for the enumeration of total coliforms, total enterobacters [25,26], total yeast and molds and total gram-negative bacteria [27,28]. Furthermore, Rogosa agar (Becton, Dickinson, Franklin Lakes NJ, USA) was employed for total lactobacilli [24]. At the end of incubation, the number of colonies was counted as units per gram of the sample.
The GLM procedures of SAS 9.1 software [29] were utilized to analyze data in a completely randomized design. Values in percentage were transformed to arcsine. The pen of birds functioned as the experimental unit. Duncan’s multiple range test was applied for comparing the means [30]. Orthogonal contrasts were performed to determine linear and quadratic relationships among treatments. Differences were considered to be statistically significant at p < 0.05.
RESULTS
As shown in Table 3, the dietary inclusion of CFPH linearly and quadratically affected BW and ADG at 10 and 24 days of age (p < 0.05). At the age of 10 days, the BW and ADG of the control group were higher than those of the groups consuming CFPH. However, at 24 days of age, only the BW of 5% CFPH-received birds was negatively affected compared to the control (p < 0.05). At 42 days of age, no significant difference was observed between experimental treatments regarding BW and ADG (p > 0.05).
In the period of 11–24 days of age, the ADFI was linearly decreased by the dietary supplementation of CFPH (p < 0.05); however, no significant effect was detected in this regard during 1–10, 24–25, and 1–42 days of age (p > 0.05). The dietary addition of CFPH in the period of 1-10 days had a linear and quadratic negative effect on the FCR (p < 0.05), but this effect was not significant in the period of 11–24, 25–42, and 1–42 days of age (p > 0.05). Moreover, liveability and EBI were not influenced by adding different levels of CFPH into the diet.
The carcass yield and relative weight of the breast, back and neck, heart, liver, pancreas, bursa of Fabricius, spleen, and abdominal fat were not affected by dietary treatments (Table 4). However, the addition of 5% CFPH to the diet linearly and quadratically increased the relative weight of the thigh compared to the control group (p < 0.05), which was not significantly different from the other levels of this product (p > 0.05). Based on the findings, the relative weight of gizzard in the group receiving 7.5% CFPH demonstrated a significant linear decrease when compared to the control (p < 0.05).
The relative weight and length of the duodenum and ileum were not affected (p > 0.05) by the dietary inclusion of CFPH (Fig. 1). Meanwhile, the relative weight and length of the jejunum were linearly influenced by the dietary supplementation of CFPH (p < 0.05) so that the highest relative length was observed in the birds that received 2.5 and 5% CFPH, and the highest relative weight belonged to the group fed with 5% CFPH, which was significantly different from the control (p < 0.05).
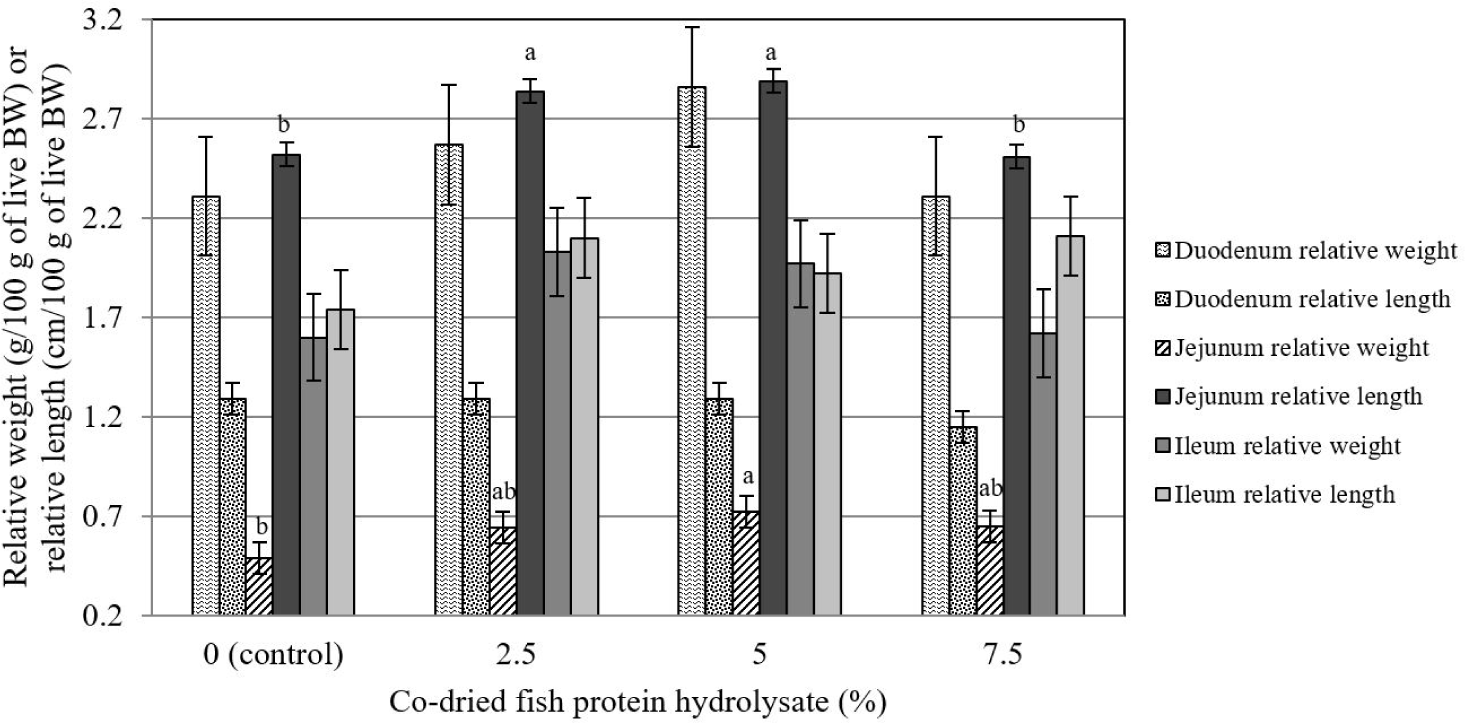
The addition of CFPH to the broiler diet had no significant effect on the hypersensitivity reaction induced by the injection of DNCB and PHA-P at 30 days of age (Fig. 2).
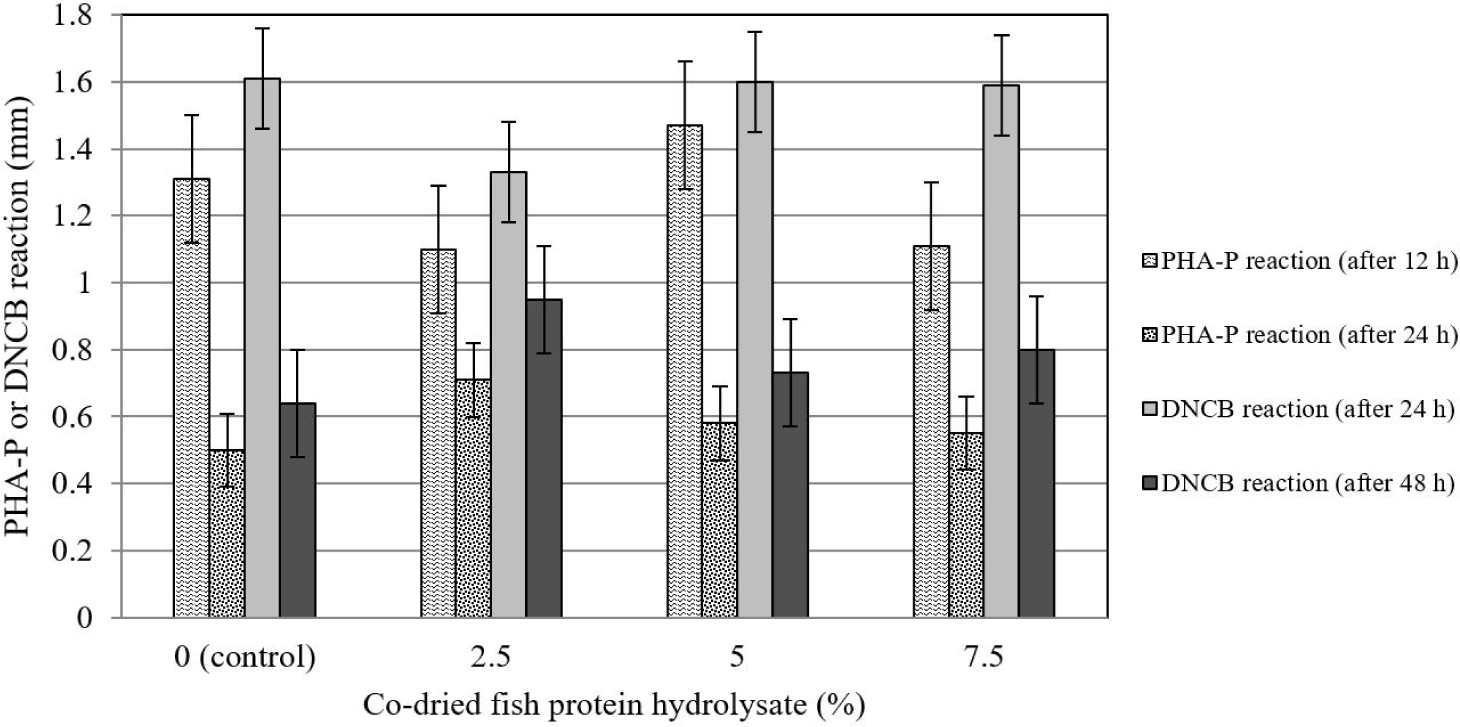
The results (Fig. 3) indicated that the total number of lactobacilli and yeasts and molds was not affected by experimental treatments (p > 0.05). Meanwhile, the effects of increasing the level of CFPH on coliforms, enterobacters, and the total gram-negative bacteria population were linearly significant (p≤0.05) so that the highest number of these three bacterial species was observed in the control group, and their number decreased by an increase in the CFPH level.
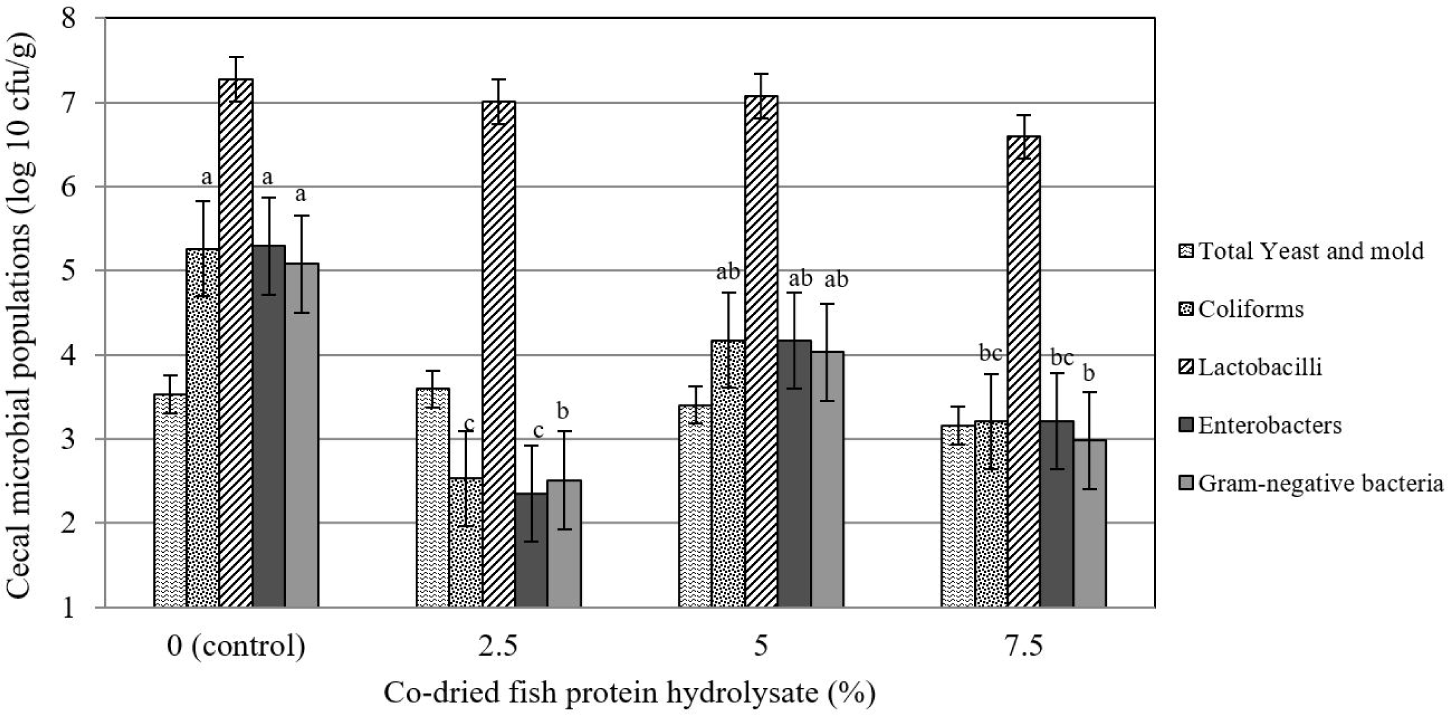
DISCUSSION
The dietary inclusion of CFPH up to the level of 7.5% exerted no significant impact on the productive traits of broilers, including BW, FI, ADG, FCR, EBI, live-ability, and carcass percentage at 42 days of age. This finding is consistent with the results of some other researchers. For instance, Al-Marzooqi et al. [31] reported that fish-derived recycled protein could be substituted for part of soybean meal in broiler diets without adversely affecting performance. Similarly, Ramírez et al. [32] concluded that the hydrolyzed protein obtained from a mixture of several fish waste in the broiler diets had no negative effect on the growth performance and meat quality of broilers, and thus it can be replaced with part of the plant proteins in the poultry diet as a reliable feed source. On the other hand, Ramírez et al. [33] found that the hydrolyzed protein of fish in a quail diet has a positive effect on the meat quality and growth performance of birds and can be replaced with some of the common plant proteins in the diet. In another study, the addition of 5% hydrolyzed salmon protein to the diet improved the performance of broilers compared to controls [14].
Meanwhile, in the present study, the addition of FPH to the diet caused a decrease in the BW at the age of 10 days. As a possible reason for this phenomenon, it can be supposed that since the FPH contains fish waste (including undigested contents of the fish digestive tract, intestinal tissue, and the like), the low digestibility of this product at an early age resulted in decreasing broiler performance in the first ten days of life. It is noteworthy that these negative effects diminished concurrent with growing and improving the digestive capacity of the bird [34] so that no significant difference in BW was observed between experimental groups at 42 days of age. Another reason is that according to the nature of FPH, part of energy supply in diets containing this product is of fish oil origin. Specifically, the amount of fish oil in the aforementioned test diets was 0%, 0.525%, 1.05% and 1.57% respectively. In the early days of age, the secretion of bile FAs is insufficient [35], which can cause a decrease in the ability to dietary fat absorption and a decrease in performance. Along with increasing age and the improvement of the bird’s absorption ability, the weight loss was compensated; but probably due to the lower weight at the age of 10 days, the expected improvement in performance traits such as weight, feed consumption and FCR was not observed. Other reasons for the difference in the results of the present study in terms of performance with former findings are probably variations in fish species, the type of processing applied for hydrolysis, and the other existing components in the final product such as fillers or moisture-absorbing compounds. During 1–24 days, the FI of the groups receiving 5 and 7.5% CFPH was lower than that of the birds fed with 2.5% CFPH and the control; this may be related to the lower weight of birds in both latter groups.
In the current study, carcass components (e.g., carcass yield, breast, back, and neck) and most internal organs were not affected by CFPH supplementation. The ineffectiveness of dietary sources containing fish oil (e.g., CFPH containing a 21% ether extract) on the relative weight of internal organs has also been reported by other researchers [36]. At the same time, relative weight of thigh tended to improve by increasing the dietary level of CFPH and the highest relative weight of thighs were observed in the birds received 5% of CFPH. Thigh muscles are of the places for fat storage in poultry [37] and the CFPH oil is more absorbable at the end of the rearing period, therefore, it may result in increase of fat storage in the thigh and its relative weight.
The dietary inclusion of 7.5% CFPH caused a significant reduction in the relative weight of the gizzard compared to the control (p < 0.05), which is in conformity with the results of Kiflay et al. [38]. Researchers have previously found that aquatic-based products (including fish meal) have the potential to erode the gizzard [39]. The lesions can range from small scratches on the gizzard to severe erosion and bleeding. While processing aquatic products, histidine or histamine in the body parts of fish can react with lysine to produce a compound called gizzerosine. Gizzerosine is not a biogenic amine, but the ability to stimulate acid production by this compound is 10 times stronger than histamine, which can lead to gizzard erosion [40]. In the present experiment, although no obvious lesions were observed in the gizzards of the CFPH-fed chickens, it can be assumed that the occurrence of this phenomenon in a mild and subclinical manner is the reason for the relative weight loss of this organ in birds receiving the highest level of CFPH.
The effect of experimental treatments on the relative weight and length of the jejunum was significant (p < 0.05) so that the highest length was observed in chickens that received 2.5 and 5% CFPH, and the highest weight was found in 5% CFPH-fed birds, which represents a significant difference from the control group. Few reports have evaluated the effects of a supplementing diet with hydrolyzed fish protein on the gastrointestinal characteristics of broilers. A recent study reported an increase in the ratio of villus length to crypt depth in the jejunum of chickens receiving hydrolyzed fish protein [41]. It might indirectly have a positive impact on the relative weight and length of this segment of the intestine. In contrast, Saki et al. [42] demonstrated that increasing the level of fish-based products from 2.3% to 8.7% in the diet exerted no significant effect on the relative weight and length of different parts of the intestine at 42 days of age. Discrepancies in the results can be related to modifications in processing methods, as well as the level of product consumption in the diet.
The density and composition of the intestinal microbial population are among the main characteristics of the intestinal ecosystem that help maintain its health and regulate host function. The reduction in the number of harmful bacteria (specific coliforms) as a result of the use of hydrolyzed fish protein in the present study is in line with the findings of Some other studies [1,41]. Organic acids in processed foods can eradicate undesirable bacterial species either directly by preventing penetration into the cell membrane or indirectly by acidifying the gastrointestinal tract environment [43]. Reduction in the number of coliforms in our experiment can be related to the preventing penetration into the cell membrane, as, the number of lactobacilli was not significantly affected by experimental treatments in the current study. Similar to our results, Al-Khalaifah and Al-Nasser [44] reported the ineffectiveness of consuming a food source containing fish oil on the population of lactobacilli in the ileal contents of broiler chickens. In this regard, Seidavi and Simões [45] found that the dietary inclusion of a source containing fish oil up to 2% did not have a negative effect on the population of beneficial gastrointestinal bacteria and helped maintain the health of birds.
A hypersensitivity reaction is a test in which the proliferation of a set of cells related to the cellular immune system, especially T lymphocytes, is stimulated and indirectly evaluated via the intradermal injection of compounds such as PHA-P and DNCB [46]. In the present experiment, cellular immune responses were not affected by CFPH supplementation, which contradicts the findings of Al-Khalaifah and Al-Nasser [44]. These researchers concluded that the dietary addition of food sources containing omega-3 and omega-6 FAs (e.g. fish oil) intensified the hypersensitivity reaction to the subcutaneous injection of PHA-P. The difference in the results is probably due to the dissimilarity in the processing method and the filler materials used in the experimental fish by-product.
CONCLUSION
In general, it is possible to feed broilers with CFPH up to the level of 7.5% without adverse effects on performance and immunity. Efficient utilization of fish by-products and agricultural residues is obtained by co-drying of such rest raw materials for animal nutrition. The application of CFPH in broiler nutrition can decrease the level of soybean meal in feed formulation and it can be considered as a new protein feed supplement in animal production especially in developing countries where the animal production is dependent on importation of plant protein sources. It is also suggested to incorporate this new supplement in other livestock’s diets.