INTRODUCTION
Prenatal and postnatal skeletal muscle growths in animals are due to embryonic myoblasts and satellite cells, respectively. In particular, myosatellite cells found between the sarcolemma and the basal lamina participate in muscle development, recovery, and regeneration [1]. Although they are normally in a resting state, they can be activated by external stimuli such as muscle damage and exercise. Activated satellite cells can repeatedly increase myoblasts through proliferation according to demands of skeletal muscle. Through differentiation, myoblasts can attach to each other to form myotubes and myofibroblasts. By fusion to the skeletal muscle, myoblasts can regenerate and grow [2,3]. These myosatellite cells play an important role in muscle regeneration after injury. Among various cell types for cultured meat production, myosatellite cells have been shown to be the most effective and promising [1]. Among stem cells, except for satellite cells, there are embryonic stem cells, induced pluripotent stem cells, and adipose-derived adult stem cells. Although these cells have sufficient differentiation capacity [4], whether they can successfully form muscle tissues has not been reported yet.
In 2013, a company made the first cultured beef hamburger. Since then, dozens of companies have entered the cultured meat field, developing a variety of products including chicken, beef, pork, and seafood [5]. In addition, cell-based cultured meat development is being actively carried out in several countries such as the United States, Israel, the Netherlands, Canada, Japan, Singapore, Spain, China, the United Kingdom, and Turkey [6]. A large amount of muscle fibers for cultured meat can be obtained with only a very small number of cells [7]. Although various cells can be used for the production of cultured meat, C2C12 myoblast is popularly used for cell culture experiments including cultured meat because it rapidly proliferates and differentiates with the advantage of being well cultured in a relatively poor environment [8]. Various cattle breeds are being researched for cultured meat production. Various studies such as gene expression, effects of vitamins, and hypoxia of Hanwoo myosatellite cells have been reported [9]. However, there are very few research on cultured meat production using Hanwoo myosatellite cells. Hanwoo is an indigenous Taurine cattle in Korea. It is characterized by marbling, soft texture, juiciness, and unique flavor [10]. Domestic consumption of Hanwoo per capita in 2021 was 4.4 kg, an increase of 4.8% compared to 2020 when it was 4.2 kg, showing a continuous increase in Hanwoo consumption [11]. As such, we judge that it would be good to use Hanwoo, which accounts for a large portion of domestic beef consumption, as a key raw material for cultured meat research, like other breeds of cattle abroad where research is actively underway for cultured meat production.
Cultured meat shows a lot of potential as a solution to various problems, including environmental pollution, methane emission, land and water shortage, and the welfare of livestock caused by consumption of meat food [12]. Despite many advantages of cultured meat, the high production cost compared to meat produced by a general method is the reason for the lack of its popular consumption [13]. To lower the production cost of cultured meat, culture efficiency should be good above all else. Thus, it is necessary to study a method to increase the culture efficiency by shortening the time for proliferation and differentiation of cells to prepare cultured meat. Cell culture may vary depending on various external conditions such as cell type, compositions of culture medium, pH of culture, time of culture, air conditioning, growth factors, mechanical, electronic, gravitational, and so on [14,15]. Among which various external conditions, satellite cells are very sensitive to temperature changes, which can greatly affect their proliferation and differentiation [16]. For example, in the case of primary human skeletal muscle cultured cells, human skeletal muscle myoblasts, and C2C12 mouse myoblasts, diameters of myotubes cultured at 39°C are larger than those cultured at 37°C [17]. In addition, cells cultured at 43°C could not proliferate well due to heat shock [18]. When cells are exposed to a high temperature above a certain level, various cellular dysfunctions such as inhibition of protein synthesis, defects in protein structure and function, and changes in morphology due to cytoskeletal rearrangement can occur [19].
To provide data for using Hanwoo myosatellite cells as cultured meat, the proliferation and differentiation efficiency of Hanwoo myosatellite cells in comparison with C2C12 cells according to culture temperature of 37°C and 39°C.
MATERIALS AND METHODS
Before using Hanwoo myosatellite cells, preliminary experiments were performed using C2C12 cells. C2C12 cells (CRL-1772, ATCC, Manassas, VA, USA) are commonly used in cultured meat experiments. Hanwoo myosatellite cells used in the experiment were collected from the round top muscle of one 34-month-old Hanwoo at Farmstory Hannaeng located in Eumseong-gun, Chungcheongbuk-do, Korea. These collected muscles were moved to the laboratory using an ice box. Hanwoo myosatellite cells were collected with an isolation technique in the laboratory.
A flask coated with collagen for proliferation and a flask coated with Matrigel for differentiation were prepared. The collagen coating solution was prepared by diluting a collagen solution of 5 mg/mL with distilled water to a concentration of 0.5%. 1M acetic acid was added to the collagen coating solution to have a final concentration of 2% for collagen solubilization. After dispensing the prepared collagen solution into a flask, it was left in an incubator at 37°C for at least 16 hours. After removing the coating solution by suction before using in the experiment, the flask was washed twice using 1X phosphate buffered saline (PBS; HyCloneTM, Cytiva, Logan, Utah). It was then dried for use in the experiment. For Matrigel coating, a solution of Matrigel was prepared by diluting it with cold 1X PBS at a ratio of 1:200. After Matrigel coating, the flask was then left in an incubator at 37°C for at least 4 hours. After removing the coating solution using suction, the flask was washed once with 1X PBS. It was then dried before it was used in the experiment.
For cell proliferation, Ham’s F-10 (11550-043, Gibco, Waltham, MA, USA) medium supplemented with 20% fetal bovine serum (FBS; 35-015-CV, Corning, Corning, NY, USA) and 1% penicillin-streptomycin-amphotericin B (PSA; 17-745E, Lonza, Basel, Swiss) was used. For effective cell proliferation and growth, basic fibroblast growth factor (bFGF; Gibco) at a final concentration of 0.05% was added to the flask. Medium used for differentiation was DMEM (11995-065, Gibco) supplemented with 2% FBS and 1% PSA. For cell proliferation during experiments, Hanwoo cells and C2C12 cells were seeded at a density of 1,800 cells/cm2 and cultured in an incubator at 37°C with 5% CO2 nor 39°C with 5% CO2 for 5 days and 4 days, respectively. In the case of differentiation, cells were cultured in growth medium (GM) until confluent in a 96-well plate or a T25 flask and then differentiated into muscle myotubes in differentiation medium (DM).
Cells were detached with trypsin. The number of cells was counted using a cell counter (Countess cell FL automated cell counter, Invitrogen, Waltham, MA, USA) after staining with trypan blue solution (T8154, Sigma-Aldrich, Burlington, MA, USA). A trypsin neutralization solution (2% FBS in PBS) was used to neutralize trypsin.
To measure proliferation capacity using an antibody, Hanwoo cells and C2C12 cells were seeded at a density of 1,800 cells/cm2 in a 96-well plate and cultured in an incubator at 37°C with 5% CO2 or 39°C with 5% CO2 for 3 days and 4 days, respectively. After removing the culture medium, cells were washed with 1X PBS and treated with 2% paraformaldehyde (GeneAll, Seoul, Korea) at 37°C for 45 minutes. After washing twice with 1X PBS, cells were treated with 0.1% Triton X (Sigma-Aldrich, St. Louis, MO, USA) at room temperature for 20 minutes. Thereafter, cells were incubated with 2% bovine serum albumin (BSA; Roche, Basel, Swiss) at room temperature for 30 minutes, washed twice with 1X PBS, and incubated with Pax7 (Paired box 7) antibody at 4°C overnight. After washing twice with 1X PBS, cells were incubated with secondary antibody (Goat anti-Rabbit IgG [H+L] Cross-Adsorbed Secondary Antibody, Alexa Fluor 488) at room temperature for 2 hours. Finally Hoechst reagent was added. Immunofluorescence-stained Hanwoo myosatellite cells and C2C12 cells were observed with an EVOS-5000 optical microscope. Images were obtained after dividing each well into five zones. Each treatment was repeated in five wells. The number of muscle cell nuclei number, myotube width, fusion index were counted by an experienced expert on imaging data using ImageJ program.
To measure the differentiation, Hanwoo myosatellite cells and C2C12 cells were seeded into 96-well plates coated with Matrigel at 1,800/cm2 each well and then cultured at 37°C or 39°C. After proliferation for 3 days and 4 days, respectively, DM was used to replace GM. Differentiation was performed for 4 days and 5 days, respectively. Differentiation ability was measured monoclonal anti-myosin antibody, was used as the antibody, and goat anti-mouse IgG1 cross-adsorbed secondary antibody, Alexa Fluor 488 was used as the secondary antibody.
Hanwoo myosatellite cells and C2C12 cells were dispensed into Matrigel pre-coated T25 flasks and cultured at 37°C and 39°C as described above. When these T25 flasks were more than 80% confluent, GM was replaced by DM. Differentiated Hanwoo muscle cells and C2C12 cells in T25 flasks were sampled for western blotting. These T25 flasks were kept cold and washed with cold 1X tris-buffered saline (TBS; Bio-Rad, Hercules, CA, USA). Then 1X radio-immunoprecipitation assay (RIPA) lysis buffer (Rockland, Gilbertsville, PA, USA) was dispensed and cells were separated from the flask using a cell scraper. Protein concentrations in samples were measured by Bradford assay. These quantitatively measured protein samples were separated by TGX Precast Gel (Bio-Rad) and transferred to an Immun-Blot PVDF membrane (Bio-Rad). The membrane was blocked with EveryBlot Blocking Buffer (Bio-Rad) at room temperature for 1 hour. Proteins of Hanwoo myosatellite cells were incubated with primary antibodies against β-actin, myosin levels for one day at 4°C. Proteins of C2C12 cells were incubated with primary antibodies against β-actin and myosin at 4°C for one day. Membranes were washed twice in TBS with 0.1% tween® 20 (Bio-Rad) at room temperature for 10 minutes and then incubated with Affinity Purified Goat Anti-Mouse IgG (H+L) horseradish peroxidase (HRP)-conjugated antibody at room temperature for 1 hour. After washing 4 times with TBST again (10 minute each wash), Clarity western ECL substrate (Bio-Rad) was used to detect proteins.
Hanwoo myosatellite cells and C2C12 cells were dispensed into Matrigel pre-coated T25 flasks and cultured at 37°C and 39°C in the same manner as described above. When the T25 flask was more than 80% confluent, GM was replaced by DM. Muscle stem cell samples were collected by scraping differentiated Hanwoo muscle cells into a T25 flask with a cell scraper. TRIzol reagent (Ambion, Carlsbad, CA, USA) was used for RNA extraction. In the case of Hanwoo myosatellite cells, expression levels of MYOG (myogenin), MyHC (myosin heavy chain), MYF6 (myogenic factor 6), and MB (myoglobin) were determined. cDNA was prepared using a Reverse Transcription Master Premix (ELPIS-BIOTECH, Korea). For RT-qPCR of Hanwoo myosatellite cells, a template RNA Primer Mixture was prepared using 1.0 μg of mRNA as a template according to the manufacturer’s manual, followed by incubation at 60°C for 60 minutes and 94°C for 5 minutes. Expression levels of MYOG, MyHC, MYF6, and MB were analyzed byqRT-PCR. Glyceraldehyde-3-phosphate dehydrogenase (GAPDH) gene was used as an internal control for expression level analysis. The qRT-PCR reaction had a volume of 20 μL consisting of 1 μL of cDNA as a template, 10 μL EzAmp™ FAST qPCR 2X Master Mix (ELPIS - BIOTECH, Daejeon, Korea), and 1 μL of each primer. Amplification was conducted at 95°C for 10 minutes followed by 40 cycles of 95°C for 10 seconds and 60°C for 20 seconds. Gene-specific primers were: MYOG, Forward – AGA AGG TGA ATG AAG CCT TCG A and Reverse – GCA GGC GCT CTA TGT ACT GGA T; MyHC, Forward - AGG AAG AGT TCC AGA AAA CC and Reverse - TGG AGC TGT AGG TCA TTT TT; MYF6, Forward - GGT GGA CCC CTT CAG CTA CAG and Reverse - TGC TTG TCC CTC CTT CCT TGG; MB, Forward - AAG AGG TGG ATG GGT TAG GG and Reverse - GGC ATT GAG GTG AAA GGA AA; and GAPDH, Forward - CAC CCT CAA GAT TGT CAG C and Reverse - TAA GTC CCT CCA CGA TGC.
In RT-qPCR of C2C12, MYOG, MyHC, and MB expression levels were measured. Amplification was conducted at 95°C for 10 minutes followed by 40 cycles of 95°C for 10 seconds and 53°C for 20 seconds. Gene-specific primers were: MYOG, Forward – GCC CAG TGA ATG CAA CTC CCA CA and Reverse – CAG CCG CGA GCA AAT GAT CTC CT; MyHC, Forward - AGC AGC GAC ACT GAA ATG GA and Reverse - GTT GTC GTT CCT CAC GGT CT; MB, Forward - GGA AGT CCT CAT CGG TCT GT and Reverse - GCC CTT CAT ATC TTC CTC TGA; and GAPDH, Forward - GTG GCA AAG TGG AGA TTG TTG CC and Reverse - GAT GAT GAC CCG TTT GGC TCC.
All measurements were repeated at least three times. A statistical processing program SAS (9.4 for Windows, Cary, NC, USA) was used to test the significance of results. To compare significant differences between measured values, Duncan multiple range test was performed at a significance level of p < 0.05.
RESULTS
C2C12 cells in flasks were cultured at 37°C and 39°C for 4 days (Fig. 1A). Live cell counting and viability of C2C12 cells cultured at 37°C and 39°C for 4 days were measured (Fig. 1B). Doubling time was calculated to compare differences by culture temperature (Fig. 1B). For C2C12 cells, more live cells were obtained at 37°C than at 39°C based on live cell count (p < 0.05). Cell viability was also found to be higher at 37°C than at 39°C but showed no significant difference (p > 0.05). C2C12 cells confluent in flask were differentiated with DM. C2C12 cells cultured at 37°C showed differentiated after culturing for 3 days. The myotube was highly differentiated after culturing for 5 days (Fig. 1A). It tore off after culturing for 7 days. C2C12 cells cultured at 39°C showed differentiation after culturing for 2 days. The myotube was highly differentiated after culturing for 4 days (Fig. 1A). It tore off after culturing for 7 days.
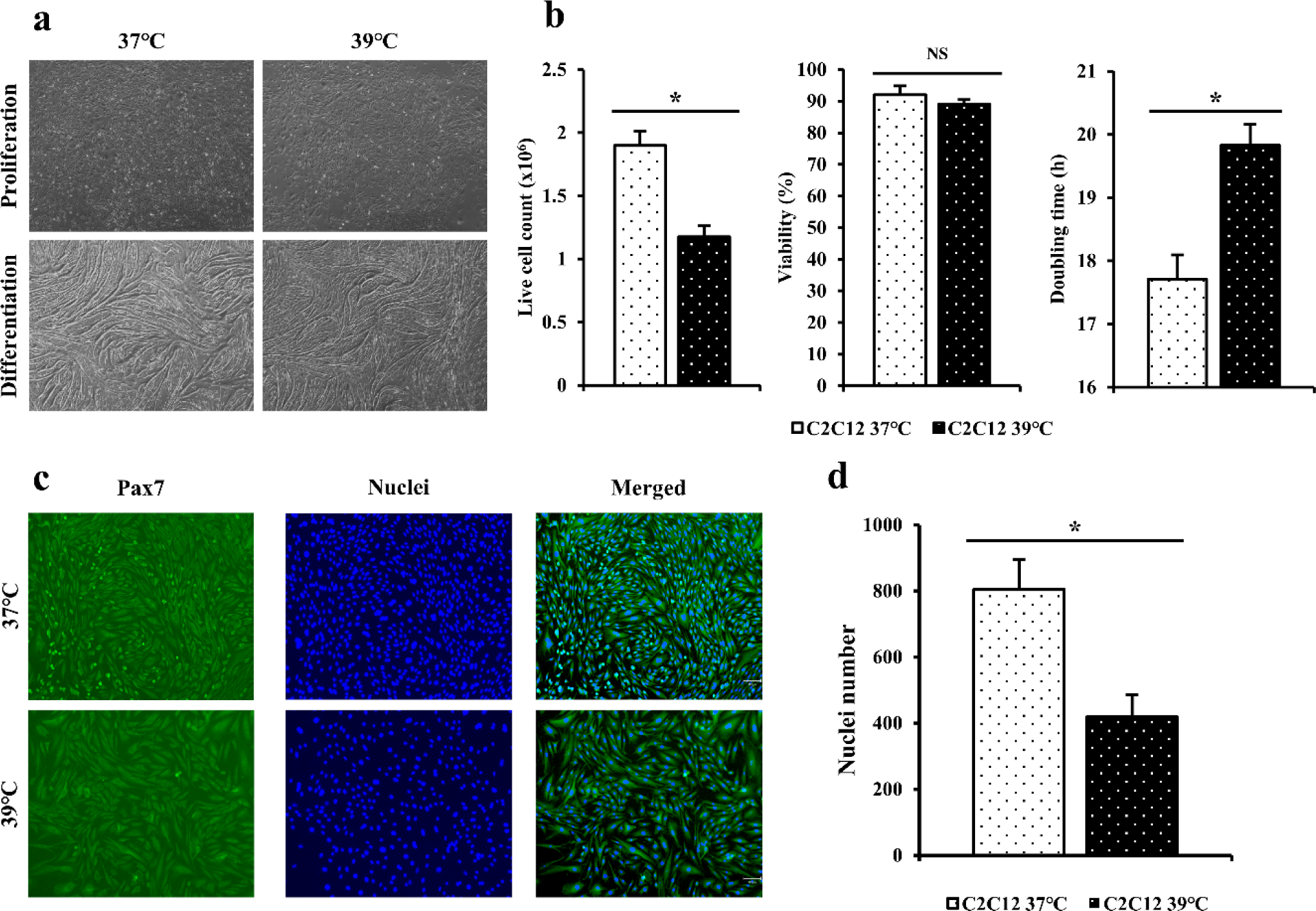
Immunofluorescence staining was performed for C2C12 cells cultured for 4 days using Pax7 antibodies and Hoechst (Fig. 1C). When dyed area was observed under a microscope, blue color indicated nuclei and the green color indicated Pax7. When the number of nuclei was calculated based on stained cells and nuclei (Fig. 1D), the distribution of C2C12 cultured at 37°C appeared to be significantly higher (p < 0.05) that that cultured at 39°C.
Immunofluorescence staining was performed using myosin antibodies and Hoechst to indirectly compare differentiation power of C2C12 cells differentiated at 37°C and 39°C for 4 days (Fig. 2A). When dyed area was observed under a microscope, the blue color indicated the nuclei and the green color indicated myosin. When immunofluorescence staining was performed to myotube width, number of nuclei, and fusion index (Fig. 2B), it was found that C2C12 cells differentiated at 39°C and 37°C showed no significant difference in myotube thickness and fusion index of cells (p > 0.05). However, cells differentiated at 37°C had significantly higher number of nuclei (p < 0.05).
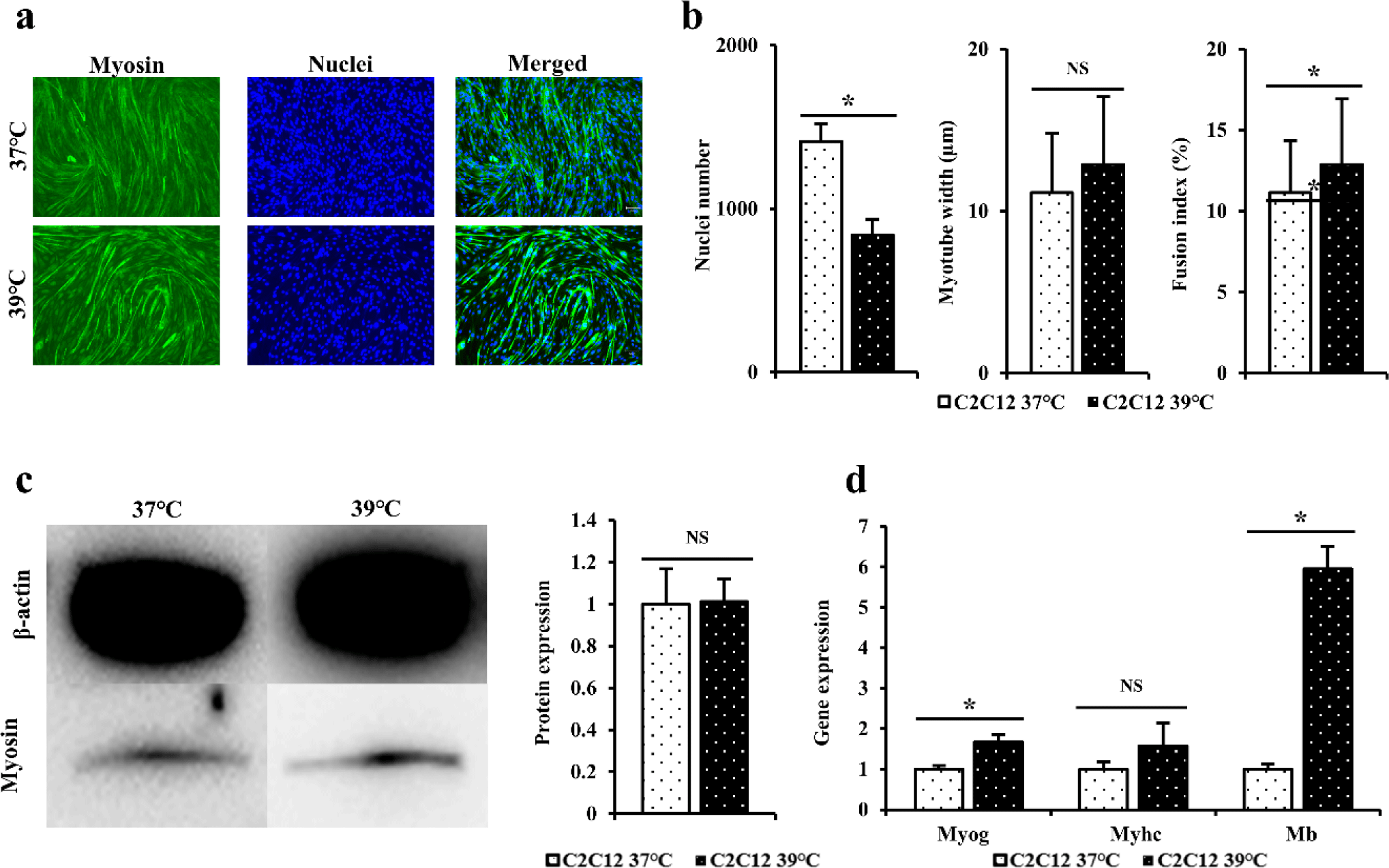
Western blot was performed to determine myosin levels in C2C12 differentiated for 3 days at 37°C or 39°C (Fig. 2C). When western blot results of C2C12 cells differentiated at 37°C and 39°C were compared, level of myosin protein in C2C12 differentiated at 39°C was measured to be relatively higher than those at 37°C, although such differences were not statistically significant (p > 0.05). A similar pattern was found for myotubes based on immunofluorescence staining.
RT-qPCR was performed to confirm quantitative gene amounts of Myog, Myhc, and Mb in C2C12cells differentiated at 37°C and 39°C for 3 days (Fig. 2D). When expression levels of the above gene were measured to determine the degree of formation of differentiated myotubes, gene levels of Myhc in cells differentiated at 39°C were higher than those in cells differentiated at 37°C, although these differences were not statistically significant (p > 0.05). Gene levels of Myog and Mb were significantly higher in cells cultured at 39°C than in cells cultured at 37°C (p < 0.05).
After completing the preliminary experiment using C2C12 cells, the same experiment was performed on Hanwoo myosatellite cells. Hanwoo satellite cells in flasks were cultured at 37°C and 39°C for 5 days (Fig. 3A). Live cell counting and viability of Hanwoo myosatellite cells cultured at 37°C and 39°C for 5 days were measured (Fig. 3B). Doubling time was calculated to compare differences by culture temperature (Fig. 3B). For Hanwoo myosatellite cells, more live cells were obtained at 37°C than at 39°C based on live cell count (p < 0.05). Cell viability was also found to be higher at 37°C than at 39°C but showed no significant difference (p > 0.05). Hanwoo myosatellite cells confluent in flask were differentiated with DM. It was confirmed that Hanwoo myosatellite cells differentiated at 37°C had myotube formation after culturing for 2 days. They were highly differentiated after culturing for 4 days (Fig. 3A). The myotube tore off after culturing for 6 days. On the other hand, Hanwoo myosatellite cells cultured at 39°C were differentiated after 1 day of culture. They were highly differentiated after culturing for 3 days (Fig. 3A). The myotube quickly tore off after culturing for 4 days.
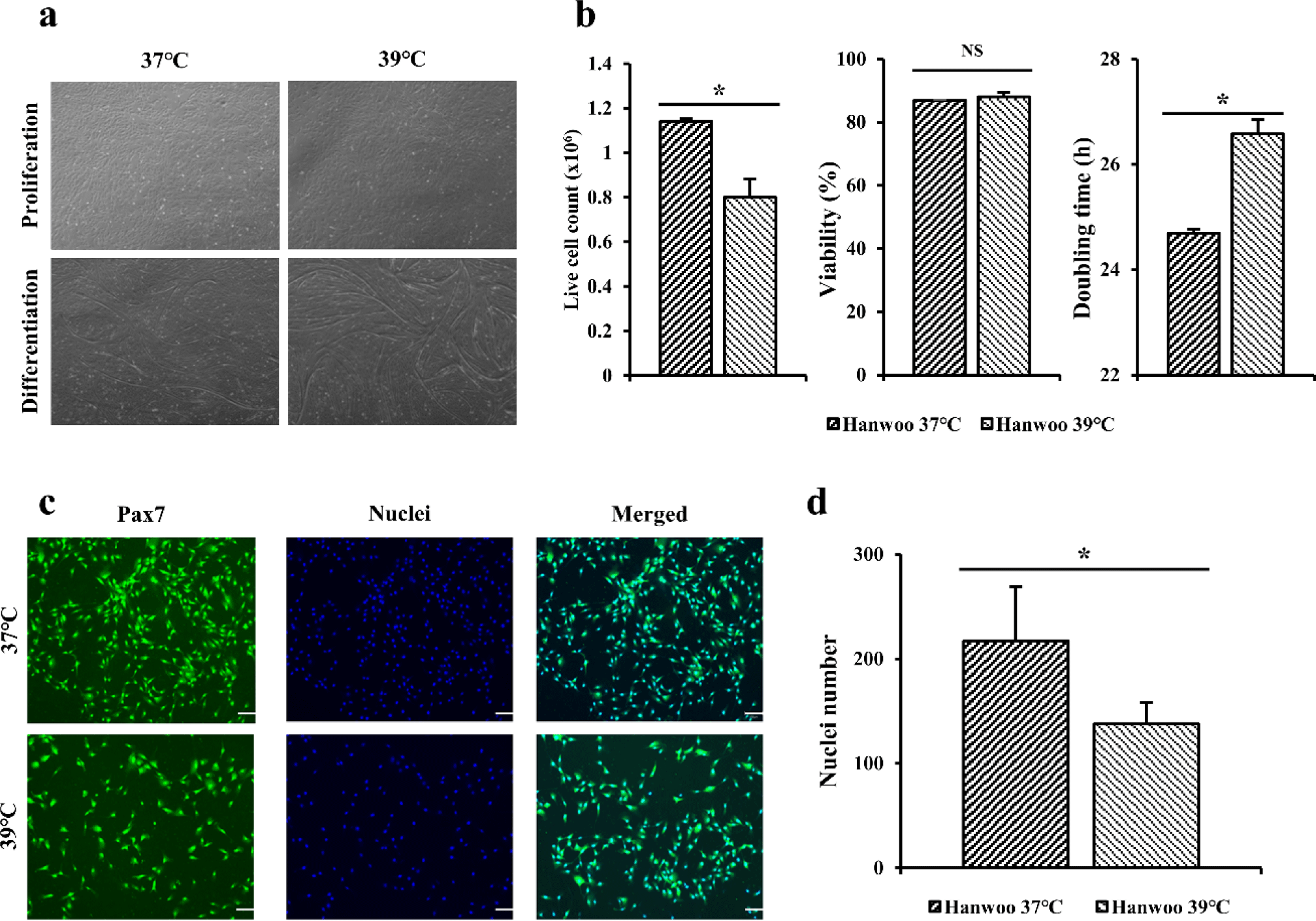
Immunofluorescence staining was performed for Hanwoo myosatellite cells cultured for 3 days using Pax7 antibodies and Hoechst (Fig. 3C). When dyed area was observed under a microscope, blue color indicated nuclei and the green color indicated Pax7. When the number of nuclei was calculated based on stained cells and nuclei (Fig. 3D), the distribution of Hanwoo myosatellite cells cultured at 37°C appeared to be significantly higher (p < 0.05) that that cultured at 39°C.
Immunofluorescence staining was performed using myosin antibodies and Hoechst to indirectly compare differentiation power of Hanwoo myosatellite cells differentiated at 37°C and 39°C for 3 days (Fig. 4A). When dyed area was observed under a microscope, the blue color indicated the nuclei and the green color indicated myosin. When immunofluorescence staining was performed to myotube width, number of nuclei, and fusion index (Fig. 4B), it was found that Hanwoo myosatellite cells differentiated at 39°C and 37°C showed no significant difference in myotube thickness and fusion index of cells (p > 0.05). However, cells differentiated at 37°C had significantly higher number of nuclei (p < 0.05).
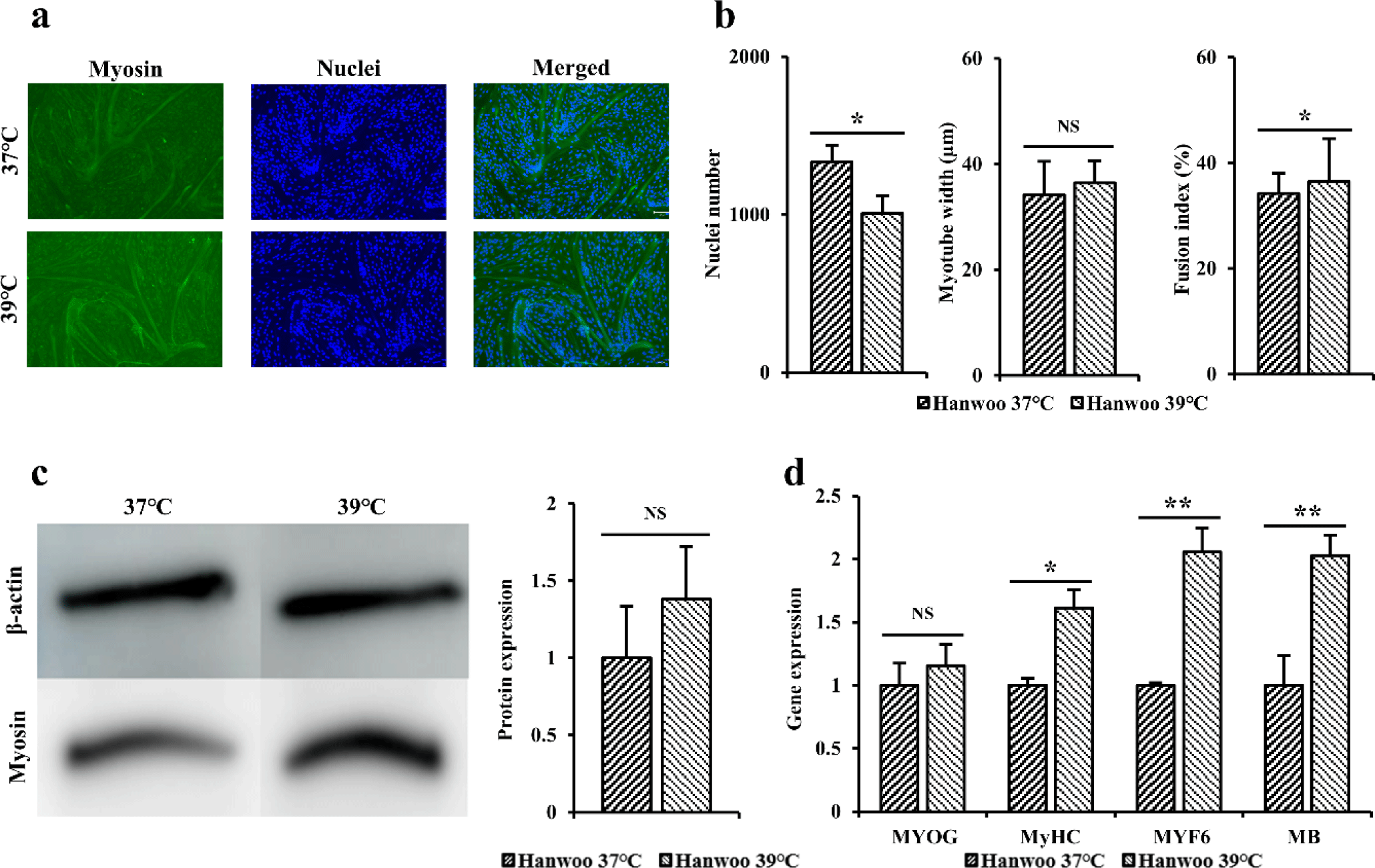
Western blot was performed to determine myosin levels in Hanwoo myosatellite cells differentiated at 37°C and 39°C for 3 days (Fig. 4C). When western blot results of Hanwoo myosatellite cells differentiated at 37°C and 39°C were compared, level of myosin protein in Hanwoo myosatellite cells differentiated at 39°C was measured to be relatively higher than those at 37°C, although such differences were not statistically significant (p > 0.05). A similar pattern was found for myotubes based on immunofluorescence staining.
RT-qPCR was performed to confirm quantitative gene amounts of MYOG, MyHC, MYF6, and MB in Hanwoo myosatellite cells differentiated at 37°C and 39°C for 3 days (Fig. 4D). When expression levels of the above gene were measured to determine the degree of formation of differentiated myotubes, gene levels of MYOG in cells differentiated at 39°C were higher than those in cells differentiated at 37°C, although these differences were not statistically significant (p > 0.05). Gene levels of MyHC (p < 0.05), MYF6, and MB (p < 0.01) were significantly higher in cells cultured at 39°C than in cells cultured at 37°C.
DISCUSSION
Hanwoo myosatellite cells and C2C12 cells were cultured in an incubator at 37°C and 39°C to observe proliferation through cell counting and immunofluorescence staining. Pax7, a transcription factor that controls the proliferation and differentiation of satellite cells during muscle formation, is an essential factor for muscle formation and differentiation of satellite cells into myoblasts [20]. Hoechst dye is the most popular dye that can distinguish cell nuclei due to their high affinity and specificity for DNA. According to Park et al. [21], mild heat stress and severe heat stress have different standards for each cells. Mild stress and severe heat stress were classified according to denaturation of pre-existing proteins, heat shock protein (HSP) synthesis, cell proliferation, differentiation, and signaling pathways. Briefly, it was defined that mild heat stress occurs at a temperature adapted to growth conditions, and severe heat stress appears at a temperature at which cell death occurs. The culturing time is as important as the culturing temperature. If the incubation temperature is increased by 1°C, the time required for the same extent of heat shock response is reduced twofold [22,23]. The effects of heat stress on the cell cycle also depend on the strength and the duration of applied heat stress [24–26]. Park et al. [21] has shown that mild heat stress plays a beneficial role in organisms through facilitating growth factor-mediated cell survival and proliferation. Mild heat stress may act as one physicochemical signal to regulate activities of membrane proteins by affecting membrane fluidity. Heat stress is presumed to positively regulate cell proliferation and differentiation, through various signal pathways such as multiple Ras signal pathways involving the Raf-extracellular-regulated kinase 1/2 (ERK1/2) pathway, phosphatidylinositol-3 kinase (PI3K)-Akt/PKB-glycogen synthase kinase (GSK)-3b pathway, and Rho-Rac1-nicotinamide adenine dinucleotide phosphate (NADPH) oxidase pathway [21,27–30].
But heat stress is known to negatively affect skeletal muscle growth by converting energy and nutrients to body temperature maintenance. Acute heat stress has been found to affect cell function by increasing proteolysis and reducing protein synthesis [31]. Sajjanar et al. [32] have shown that when C2C12 cells are cultured at 37°C or 39°C, total protein content of C2C12 cells cultured at 39°C is lower than that of cells cultured at 37°C. Mitochondria generate most of the energy required for cell function through oxidative phosphorylation (OXPHOS) [32]. Heat stress on cells can cause mitochondrial dysfunction and reduce respiratory capacity. In addition, HSP, like HSP70, induced by heat stress was found to inhibit OXPHOS and at the same time enhance glycolysis to compensate for adenosine triphosphate (ATP) imbalance [33]. HSP, a defense mechanism to protect itself from external stress [34], is an important cellular temperature resistance protein to prevent denaturation of polypeptides under heat stress. When cells face stress, intracellular material transport and protein misfolding will occur. HSP can prevent these phenomena, increasing cell viability and helping cells overcome heat stress [35]. HSP72 is known to play an important role in maintaining protein homeostasis among HSPs produced when cells are cultured at 39°C, and HSP72 expression in muscle has been reported to play a role in preserving muscle function [36]. Heat stress is known to negatively affect skeletal muscle growth by converting energy and nutrients to body temperature maintenance. Acute heat stress has been found to affect cell function by increasing proteolysis and reducing protein synthesis [31]. Kamanga-Sollo et al. [37] have shown that pig myosatellite cells with increased amounts of various HSPs due to heat stress showed proliferation rates were 35% less than other pig myosatellite cells not subjected to heat stress. Consequently, HSP-inducing culture at 39°C inhibited the proliferation of Hanwoo myosatellite cells and lowered their efficiency. Therefore, the effect of heat stress on cell cycle progression or proliferation is highly ambiguous and the cellular response to mild heat stress depends on the type of cell line analyzed [21].
Hanwoo myosatellite cells and C2C12 cells were cultured in an incubator at 37°C and 39°C to observe differentiation through immunofluorescence staining, western blot, and RT-qPCR. Myosin is a representative structural protein of muscle cells and functional protein that enables muscle contraction and relaxation as the main component of the myosin filament of myotube. It is judged as an appropriate marker for the degree of cell differentiation. MYOG, one of myogenic regulatory factors, regulates proliferation and differentiation of satellite cells, precursor cells of myofibers [38]. That is, it is expressed in the nuclei of activated satellite cells for the regeneration of myofibers. It affects the process of myofiber growth by regulating the formation of myoblasts and myotubes. MYF6 is a transcription factor that regulates myogenic and amnion cells involved in skeletal muscle formation [36]. It is known to be abundant in adult muscle cells. MyHC is a late-stage marker of muscle cell differentiation. Its expression level increases as muscle cell differentiation proceeds. Its expression level increases as muscle cell differentiation proceeds. MB, as an oxygen storage protein in muscle, serves to buffer the concentration of intracellular oxygen and promote intracellular oxygen diffusion when muscle activity increases [36]. As an oxygen storage protein in muscle, it serves to buffer the concentration of intracellular oxygen and promote intracellular oxygen diffusion when muscle activity increases [36]. Looking at the flow of differentiation, when Pax7 and Pax3 are expressed in satellite cells, they express Myf5 and MyoD and differentiate into dividing myoblasts. These myoblasts are differentiated into myocytes while expressing MYOG and MYF6. Then myocytes stop dividing and form multinucleated myotubes [39]. There are many ways to promote cell differentiation. According to Kanatous and Mammen [40], high levels of actin and myosin are induced by electrical stimulation of C2C12 cells during differentiation. Controlling incubation temperature can also be one way to control differentiation. Previous experimental results have shown that differentiation of C2C12 cells cultured at a high temperature for a long time into myotubes can be inhibited by heat shock [18]. In C2C12 cells, heat stress promotes myofibrogenesis during myogenesis, but excessive heat stress can affect muscle size as it activates the muscle atrophy signaling pathway [41]. According to study of Ikeda et al. [42], HSP-expressing C2C12 cells by heat stress did not improve myotube width and differentiation rate during differentiation culture. However, it is also known that continuous heat stress increases intracellular calcium [43], and it is reported that intracellular calcium induces hypertrophy of muscled myotubes and changes the myofiber phenotype [44]. In the case of a mild heat stress at a low temperature, cell proliferation, differentiation, and immunity can respond positively [21]. Heat stress can regulate cell survival by triggering signals including Ras, Rac1, mitogen-activated protein kinase (MAPK), and other pro-survival molecules that are independent of HSF1-HSP induction [27,28]. In the case of myocytes of quail, differentiation at 39°C for a long period of time can increase the length and diameter of myotubes than incubation at 37°C [45]. Protein contents of slow MyHC isoform and cytochrome C oxidase subunit IV are higher than others [45]. In addition, there are experimental results showing that the amount of peroxisome proliferator-activated receptor gamma coactivator (PGC)-1α protein varies according to the duration of heat stress and the temperature [17]. A febrile rise in core body temperature positively regulates cell growth and differentiation, enhancing innate and adaptive immunity [46–48]. It means that the effects of heat stress on cellular functions are pleiotropic, and that HSP alone is not sufficient to induce muscle hypertrophy. Therefore, applying heat stress by raising the culture temperature may not be sufficient for cell growth and differentiation, but it can facilitate growth factor-mediated cell proliferation and differentiation [21].
As a result of the experiment, it was confirmed that the genes involved in muscle differentiation were significantly increased in cells cultured at 39°C, but it is difficult to say that they have a direct effect on differentiation, such as the differentiation rate or the width of myotube. In conclusion, the proliferation of Hanwoo myosatellite cells and C2C12 myoblasts at 37°C could be more efficient than incubation at 39°C. Differentiation at 39°C may be more efficient than differentiation at 37°C, but there was no significant effect. For efficient differentiation, it is considered that additional treatment as well as heat stimulation is required alone. These results can serve as basic data for cultured meat using Hanwoo myosatellite cells.