INTRODUCTION
Ruminant animals depend on a diverse microbial assembly, which consists of bacteria, archaea, protozoa, and fungi, in their rumen for their survival, growth, and production of animal proteins (beef, lamb, milk, and wool). Collectively, digestive and fermentative processes convert dietary carbohydrates, primarily starch and cellulose, and dietary nitrogen, primarily plant protein nitrogen, into carbon and nitrogen sources that ruminants can utilize. However, the utilization efficiency of dietary nitrogen in ruminants is low, which is only approximately 25% [1,2]. This low nitrogen utilization efficiency not only increases the production cost but also creates a major environmental problem. Indeed, about 70% of NH3 and 30% of N2O released into the environment by anthropogenic activities are estimated to stem from livestock husbandry [3]. The ruminal microbiome participates and greatly affects the nitrogen utilization efficiency in ruminants. Conceptually, two metabolic processes can lead to low nitrogen utilization efficiency: microbial protein proteolysis and amino acid deamination. In the rumen, approximately 70% of dietary nitrogen (primarily as protein, often referred to as rumen degradable protein (RDP) is hydrolyzed to oligopeptides and free amino acids, which can be fermented to short-chain fatty acid (SCFA) and ammonia. Some of these nitrogen forms are used as nitrogen sources by ruminal microbes, primarily bacteria, to synthesize cellular proteins, which are the main direct nitrogen source of host animals [4-6]. However, a large portion of microbial cells (about 24% of the total ruminal bacteria daily) are engulfed by ruminal protozoa [7]; furthermore, approximately 50% of the engulfed bacterial protein is hydrolyzed by protozoa and discharged as oligopeptides and free amino acids [8], thereby promoting protein nitrogen recycling in the rumen. A significant portion of oligopeptides and free amino acids are fermented by amino acid-fermenting bacteria (AAFB) to SCFA and ammonia. Thus, ruminal protozoa can decrease the ruminal outflow of microbial protein, which is the main protein source of host animals, to the small intestines. They also increase the availability of substrates for AAFB and the production of ammonia, which is absorbed and converted into urea in the liver and excreted. Ruminal protozoa and AAFB have been explored extensively to understand their roles in nitrogen utilization efficiency in ruminant animals, but their interactions remain poorly understood.
Ruminal protozoa have been considered a nonvital group of microbes for host animals although they contribute to organic matter digestion and homeostasis of the rumen environment [9]. Although their peptidase and deaminase activities remain to be determined and are probably variable among different protozoal species [10,11], all ruminal protozoa engulf and digest cells of ruminal microbes, even small ruminal protozoa; subsequently, they degrade the microbial protein into oligopeptides and amino acids, producing substrates for AAFB [12]. Although AAFB can utilize carbohydrates, they can use amino acids as their sole energy and carbon sources [13]. Unlike the predominant proteolytic bacteria that have limited deamination activity, AAFB, especially hyper-ammonia-producing bacteria (HAB), including Clostridium aminophilum, Clostridium sticklandii, and Peptostreptococcus anaerobius, have high deamination activities [13-15]. Numerous studies have been conducted to understand the roles of ruminal protozoa and AAFB in ruminal protein metabolism and decrease ruminal protozoa (primarily by defaunation) and AAFB by using plant extracts [15-18]. However, effective and practical approaches are yet to be developed to decrease intraruminal protein turnover and improve nitrogen utilization efficiency.
From an ecological perspective, ruminal protozoa and AAFB can form two relationships: prey-predator relationship and mutualism. In the former relationship, ruminal protozoa benefit from preying on AAFB, but in the latter relationship, ruminal protozoa provide substrates to AAFB. We hypothesized that a better understanding of the interactions between ruminal protozoa and AAFB could help unravel the roles of ruminal protozoa and AAFB in intraruminal protein recycling and improve nitrogen utilization efficiency in ruminants. This study aimed to evaluate the interaction between Entodinium caudatum, the most predominant rumen protozoal species, and AAFB in terms of feed degradation, ammoniagenesis, and dynamics of microbial populations.
MATERIALS AND METHODS
An Ent. caudatum monoculture established from a single cell isolated from the rumen of gerenuk [19] was maintained by daily feeding of protozoal feed containing wheat grain, alfalfa, and grass and regular transfers into a fresh SP medium [20].
An AAFB consortium was established previously in our laboratory by using enrichment on casamino acids as the sole substrate [21]. Briefly, rumen fluid was collected from two rumen-fistulated Jersey dairy cows, mixed, and inoculated into a mineral medium [22] containing yeast extract (0.5 g/L) as growth factors and casamino acids (30 g/L) as the sole substrate. The culture was incubated anaerobically at 39°C and transferred every 24 h until the ammonia concentration in the culture stabilized. Proteus mirabilis, Bacillus spp., Fusobacterium ulcerans, C. aminophilum, and P. anaerobius with varying amino acid fermentation activities were identified in this AAFB consortium through 16S rRNA gene sequencing.
The monoculture ofEnt. caudatum and the AAFB enrichment culture were incubated individually or as a co-culture to explore the interaction between ruminal protozoa and AAFB (Table 1). The Ent. caudatum monoculture was washed using three filter membranes with decreasing pore sizes (50, 25, and 10 mm; Sefar Filtration, New York, USA) to remove most prokaryotes present in the Ent. caudatum monoculture. The washed Ent. caudatum cells retained on the 10 mm filter membrane were then collected into the simplex buffer (modified from Williams and Coleman, 1992 [12]) and used as the Ent. caudatum inoculum. The AAFB culture was centrifuged at 21,000×g for 7 min. The pelleted cells were washed with the simplex buffer thrice followed by centrifugation and resuspended in an SP medium [20] as the AAFB inoculum. The cell density of the AAFB suspension was estimated based on its optical density [23]. Approximately 8.8 × 107 AAFB cells/mL were inoculated into the AAFB culture and the Ec + AAFB co-culture. Both the medium and the buffer were made anaerobically by continuous sparging with O2-free CO2 gas. The medium conditions were similar among the three treatments except for the microbial inocula and a higher ammonia concentration in AAFB (data not shown).
Protozoal cells in the two Ent. caudatum-containing cultures (i.e., Ec and Ec + AAFB) were fixed and counted microscopically every 24 h of the incubation as described previously [20]. Briefly, 0.5 mL of each culture was fixed in 50% formalin solution and then mixed with 30% glycerol to dilute the culture and prevent the rapid settlement of protozoal cells during the procedures [24]. The protozoal cells were stained with brilliant green (10 mL of dye added to 1.5 mL of fixed protozoal cell suspension) to facilitate microscopic counting. A 1-mL aliquot of each stained protozoal sample was added to a Sedgewick Rafter counting chamber (no. 9851 C20, Thomas Scientific, Swedesboro, NJ, USA), and the cells within 50 different grids were counted twice and averaged.
After 3 days of incubation, 1.5 mL of culture was subsampled from each replicate culture every 24 h of the incubation. Then, 1 mL of each subsample was centrifuged at 16,000×g for 10 min at 4°C, and the pellet was used for microbial DNA extraction per the repeated bead beating (RBB) + C method [25]. The supernatant of each culture sample was used to measure pH with an Accumet AB15 pH meter (Fisher Scientific, Suwanee, GA, USA) and determine the concentrations of VFA using gas chromatography (HP 5890 series, Agilent Technologies, Santa Clara, CA, USA) and ammonia using a colorimetric assay [26]. The remaining 0.5 ml culture was used to count protozoal cells as described above. The remaining content of each culture replicate was poured into a filter bag (Ankom Technology, USA; 25 mm porosity), and the retained solid was dried in a hot-air oven at 105°C overnight [27]. The dry matter (DM) and neutral detergent fiber (NDF) content of the fresh protozoal feed and the residual feed after fermentation were determined following the method described by Van Soest et al. [28].
Quantitative real-time PCR assays were used to quantify the total bacteria and three selected AAFB species (i.e., Prevotella ruminicola, C. aminophilum, and P. anaerobius). The PCR primer sets used are listed in Table 2. One sample-derived real-time PCR standard was produced using PCR amplification for each target group of microbes with the respective specific primer set and a DNA sample pooled from all the replicates of the three treatments as the template as described previously [29]. Each PCR product was electrophoresed on agarose (1%) gel to check its expected size and purified using a PCR purification kit (Qiagen, Germantown, MD, USA). The copy number concentration of each standard was calculated based on its length (bp). A serial dilution (102–1011 copies/mL) of each standard was used to quantify the abundance of the target bacteria by using an Mx3000 real-time PCR system (Stratagene, La Jolla, CA, USA). The thermal cycling profile and detailed PCR conditions are the same as described previously [30], but the annealing temperatures shown in Table 2 were used.
Target | Primer | Sequence, 5ʹ to 3ʹ | Annealing T (°C) | Product size (bp) | References |
---|---|---|---|---|---|
Total bacteria | 27f | AGAGTTTGATCMTGGCTCAG | 55 | 1,535 | [46] |
1525r | AAGGAGGTGWTCCARCC | ||||
Total bacteria (qPCR) | Eub358f | TCCTACGGGAGGCAGCAGT | 60 | 448 | [47] |
Eub806r | GGACTACCAGGGTATCTAATCCTGTT | ||||
Prevotella ruminicola | P. rumi-F | GGTTATCTTGAGTGAGTT | 53 | 485 | [48] |
P. rumi-R | CTGATGGCAACTAAAGAA | ||||
Clostridium aminophilum | C. amin-57F | ACGGAAATTACAGAAGGAAG | 57 | 560 | [49] |
C. amin-616R | GTTTCCAAAGCAATTCCAC | ||||
Clostridium sticklandii | C. stick-185F | ATCAAAGAATTTCGGATAGG | 61 | 442 | [49] |
C. stick-626R | CAAGTTCACCAGTTTCAGAG | ||||
Peptostreptococcus anaerobius | P. anae-73F | TGCTTGCAYTRATGAAAGATG | 55 | 570 | This study |
P. anae-642R | TCTTCCAGTTTCGGAGGCTA |
Data from the three replicates were shown as the mean values of each measurement and subjected to the GLIMMIX procedure followed by Tukey’s honestly significant difference (HSD) test by using SAS 9.4 (SAS Institute, Cary, NC, USA). The model to analyze the data was as follows:
where Y is the dependent variable, m is the overall mean, Ti is the fixed effect of culture treatment, tj is the time effect, (T × t)ij is the interaction between culture treatment and time, and eij is residual error. A Pearson correlation matrix was calculated for the fermentation characteristics and bacterial abundance by using the CORR procedure in SAS and visualized in R 3.2.2 [31]. The effects of incubation time and culture treatment were considered significant at p ≤ 0.05.
RESULTS
Approximately 99% (about a decrease by 2 logs) of the bacteria present in the monoculture were removed when Ent. caudatum cells were filtered and washed, but the total bacterial population increased dramatically after 24 h of incubation (Fig. 1). Ent. caudatum grew significantly better in the co-culture after 48 h of incubation (Fig. 2). The DM digestibility was greater in the co-culture, especially at 48 h and thereafter, than in Ent. caudatum- or AAFB-alone cultures (Table 3). However, no overall difference in NDF digestibility was noted among the three cultures. The ammonia concentration was higher in AAFB and Ec + AAFB co-cultures than in Ec throughout incubation. The ammonia concentration was higher in Ec + AAFB than in AAFB at 48 h but higher in AAFB than in Ec + AAFB at 72 h.
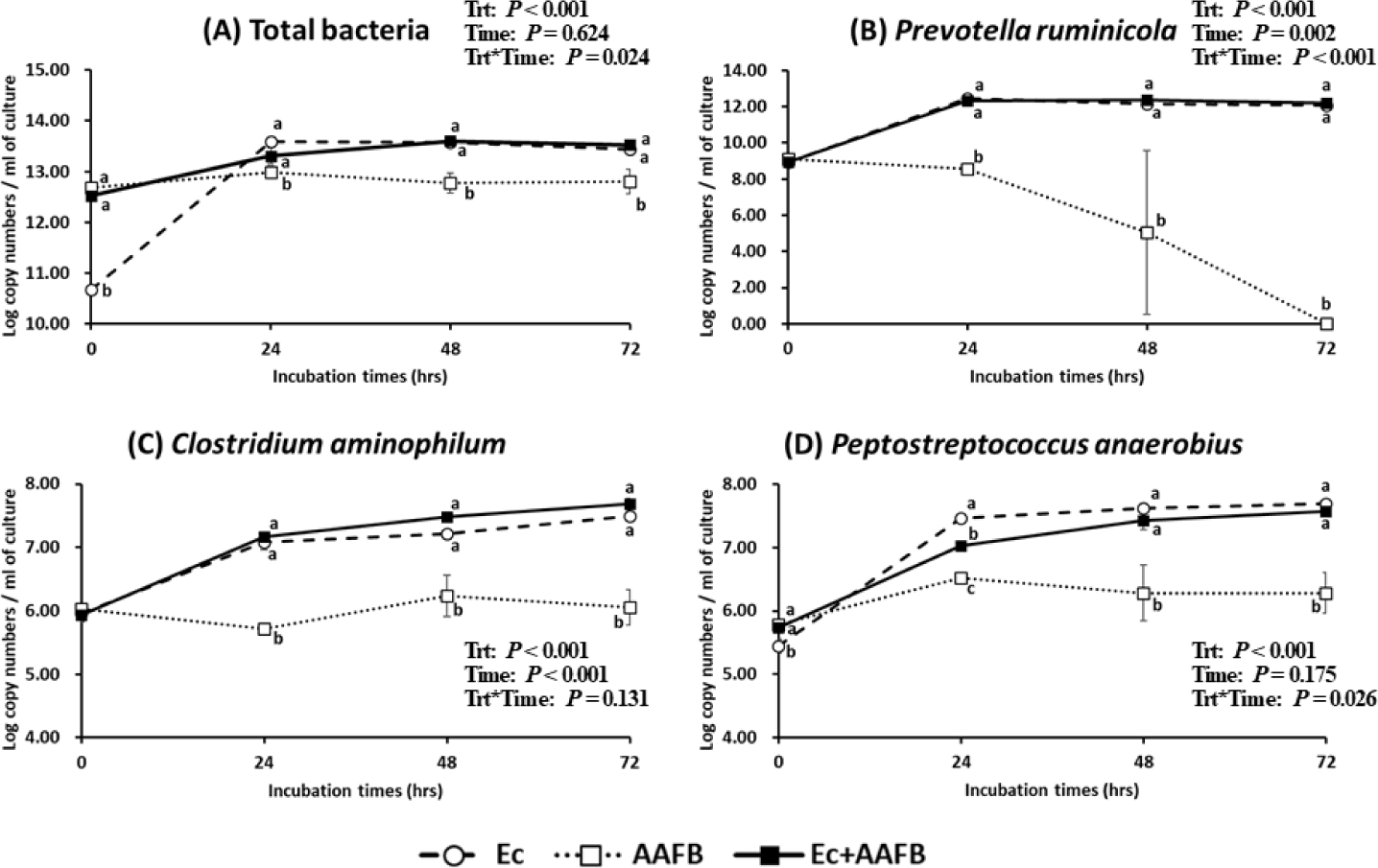
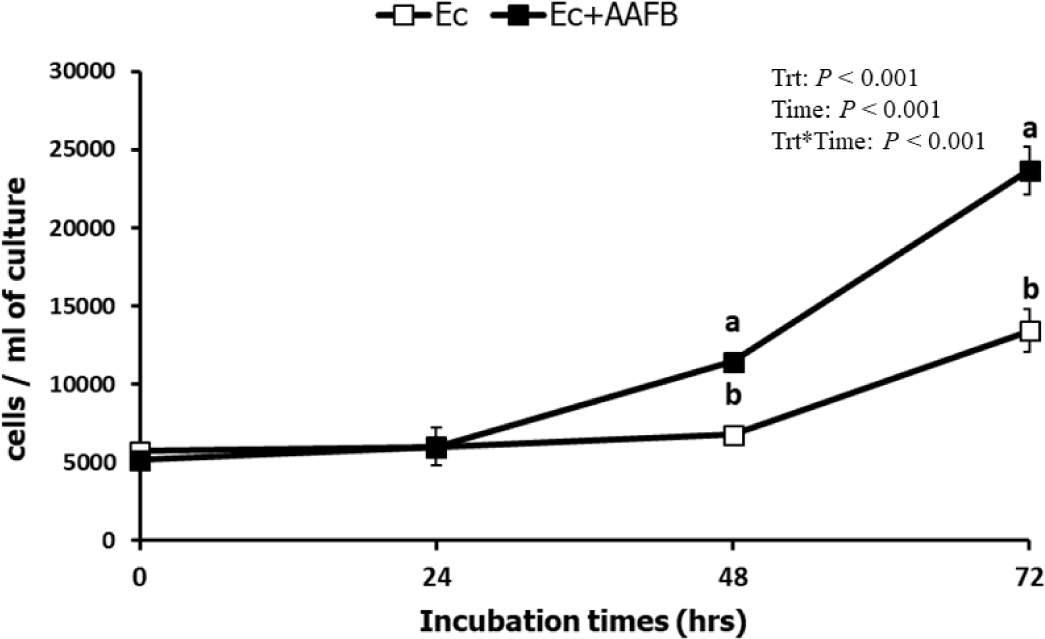
The total VFA production was higher in the Ent. caudatum-containing cultures, especially in the Ec + AAFB co-culture, than in AAFB (Table 4). The molar proportions of all VFAs differed (p < 0.01) in these two cultures and at the two incubation times. More propionate but less acetate was produced in the Ent. caudatum-containing cultures, especially in the co-culture; as a result, the acetate:propionate ratio in the two Ent. caudatum-containing cultures was significantly lower than that in the AAFB culture. The proportion of branched-chain VFAs (BCVFA) was higher in the AAFB-containing cultures than in the two other cultures at 24 h of incubation but not at 72 h of incubation.
After analyzing the fermentation characteristics and protozoal counts, we quantified the total bacteria and P. ruminicola, C. aminophilum, and P. anaerobius in the cultures. At the beginning of incubation, the abundance of the three bacterial species was similar in the three cultures except in the Ec culture which contained a smaller population of P. anaerobius (Fig. 1). Even though the Ec culture had the lowest abundance of total bacteria (at least 70-fold lower than that in the AAFB-containing cultures), it had a total bacterial abundance similar to that of Ec + AAFB after 24 h of incubation; conversely, AAFB alone had the smallest total bacterial population. At 24 h of incubation, the populations of the three selected bacterial species were similar and much larger in the Ent. caudatum-containing cultures than in the AAFB culture. After 72 h of incubation in the AAFB culture, P. ruminicola became undetectable. The initial population of C. aminophilum in all three cultures was not different, but it was significantly higher in Ent. caudatum-containing cultures than in the AAFB culture after 24 h of incubation and thereafter. Before co-culturing was performed, P. anaerobius was higher in AAFB-containing cultures, but this HAB species rapidly grew in the Ec culture during the first 24 h of incubation. This rapid growth was maintained until 72 h of incubation, and this finding was comparable with that in the Ec-AAFB culture. Because C. sticklandii, which was previously known as a culturable HAB [16], was not detected using its specific primer set in the AAFB enrichment culture (AAFB inoculum), it was not quantified in the cultures. The relative abundances of the three AAFB species are also shown in Table 5.
Correlations between the fermentation characteristics and the abundance of select bacteria are shown in Fig. 3. Ammonia concentration was weakly correlated positively with the abundance of C. aminophilum and P. anaerobius (r = 0.33 and r = 0.38, respectively) but negatively correlated with the abundance of P. ruminicola (r = −0.34). C. aminophilum and P. anaerobius were strongly correlated positively with the total VFA concentrations (r > 0.8, p < 0.001) but negatively correlated with the valerate concentration (r < −0.8, p < 0.001). Moreover, the two HAB species were positively correlated with each other (p < 0.001). The cell counts of Ent. caudatum were positively correlated (r ≥ 0.57) with the abundance of the three quantified bacterial species.
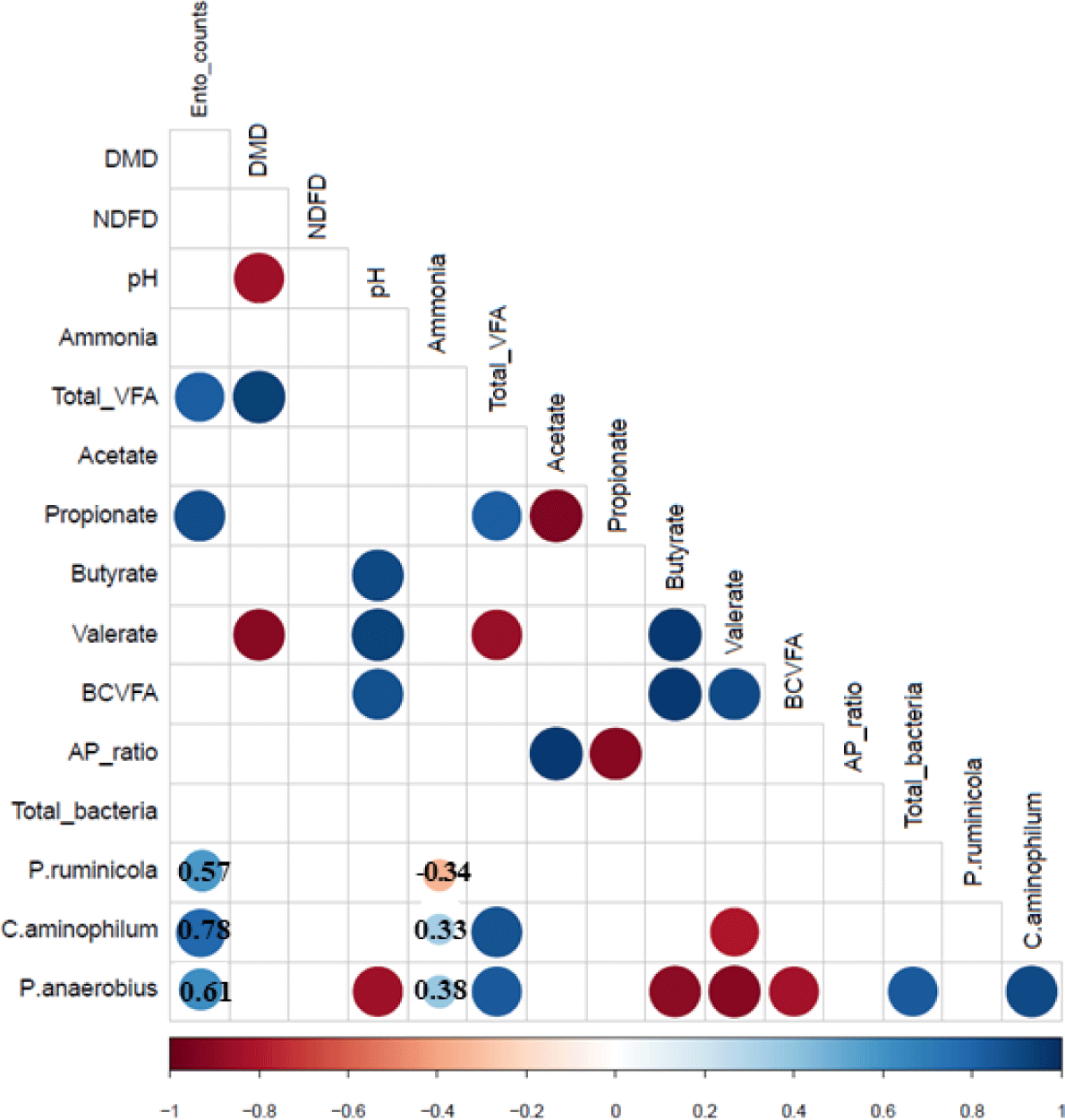
DISCUSSION
Sitting at the top of the food chain in the rumen ecosystem, protozoa form a predator–prey relationship with all members of the ruminal microbiome. Because of their proteolytic activity, including the ability to degrade microbial proteins and produce oligopeptides and amino acids, ruminal protozoa also establish other relationships with other ruminal microbes, particularly AAFB. Conceptually, ruminal protozoa can engulf and provide substrates (i.e., oligopeptides and free amino acids) to AAFB, forging a commensalistic relationship beneficial to AAFB. However, these relationships have not been deterministically investigated because of the lack of and difficulties in obtaining axenic cultures of ruminal protozoa [20]. This study was the first to explore the interactions between these two important groups/guilds of ruminal microbes by using carefully washed Ent. caudatum and an AAFB consortium.
At the beginning of incubation, the total bacterial population was about 2 logs smaller in the Ec culture than in the two other cultures. The comparable abundance of total bacteria between the Ec culture and the Ec + AAFB co-culture at 24 h of incubation clearly showed that the residual bacteria remained after the Ent. caudatum inoculum was washed and grew rapidly, reaching an abundance similar to that in the Ec culture. In previous in vitro studies, antibiotics are used to remove prokaryotes associated with protozoa [32-34]. However, antibiotics inhibit, directly and indirectly, the viability of Ent. caudatum by killing its prey [20]. Although bacteria recovered after 24 h of incubation, they were protozoan-associated populations rather than free-living populations, and they probably function with ruminal protozoa. Therefore, the interactions between Ent. caudatum and AAFB could be inferred by comparing feed digestion, fermentation characteristics, or population dynamics of bacteria between Ec and Ec + AAFB.
The Ec + AAFB co-culture had the highest DM digestibility at 48 and 72 h of incubation possibly because of the greater abundance of Ent. caudatum, which degrades starch and hemicellulose [12,35]. Although Ec and Ec + AAFB had a similar total bacterial abundance, the latter had a larger Ent. caudatum population after 48 h of incubation. The NDF digestibility did not differ among the three cultures probably because Ent. caudatum cannot degrade cellulose [36], and neither the Ent. caudatum monoculture nor the AAFB consortium contained cellulolytic microbes. The AAFB culture had the lowest total VFA concentration, but it had a higher molar proportion of acetate, butyrate, valerate, and BCVFA than the two Ent. caudatum-containing cultures. Amino acid fermenters, including HAB, produce these VFAs as their common fermentation products [14,37]. Indeed, C. aminophilum and P. anaerobius can ferment a broad range of amino acids or casamino acids to ammonia, acetate, and butyrate [38, 39].
Ent. caudatum grew faster in the Ec + AAFB co-culture than in the Ec culture during incubation. This finding verified the stimulatory effects on Ent. caudatum from the AAFB consortium. Although the Ec culture and the Ec + AAFB co-culture had similar total bacterial abundance, the latter had a greater Ent. caudatum population than the former. Thus, the Ec + AAFB co-culture could have higher bacterial recycling than the other culture. These findings were supported by higher concentrations of VFA and ammonia. The higher ammonia concentration in the Ec + AAFB co-culture than in the Ec or AAFB cultures also suggests that metabolic commensalism occurred through which Ent. caudatum provides substrates to AAFB and that AAFB are essential for ammonia production even though this bacterial consortium represents a small guild [40]. However, the Ec + AAFB co-culture did not increase the ammonia concentration after 72 h of incubation; this observation was inconsistent with a previous study that showed a two-fold higher ammonia concentration in Entodinium-faunated rumen than in fauna-free rumen of sheep [41]. In the present study, the protein added to the cultures might have been a limiting factor; therefore, ammoniagenesis in the Ec + AAFB co-culture could have been limited.
C. aminophilum and P. anaerobius are two of the three known HAB species [14]. Before incubation, their relative abundance was quite low (less than 0.002%). Their relative abundance linearly increased (p < 0.001) during incubation in the Ec culture and the Ec + AAFB co-culture but not in the AAFB culture. These results suggest that Ent. caudatum provided the substrates for these two species and stimulated their growth. The three quantified bacterial species had similar abundance before incubation, but their populations increased in the Ec culture and the Ec + AAFB co-culture but not in the AAFB culture (except for P. anaerobius) at 24 h. P. ruminicola gradually decreased and became undetectable at 72 h. These results suggest that Ent. caudatum provided the substrates for the fermentation and growth of C. aminophilum and P. anaerobius but not P. ruminicola. Previous studies demonstrated the exopeptidase activity in rumen protozoal samples [42, 43], and Entodinium species have a greater peptidase activity than large entodiniomorphs and holotrichs [44]. However, studies have yet to determine if the stimulatory effect of Ent. caudatum on the growth of C. aminophilum and P. anaerobius observed in the present study could be attributed to the exopeptidase activity of Ent. caudatum.
P. ruminicola can degrade dietary proteins but cannot use amino acids as its energy N source unless peptides are provided [11]. This ability might explain the decrease in P. ruminicola abundance in the AAFB culture and the more than 400-fold increase in P. ruminicola abundance in the Ec culture and the Ec + AAFB co-cultures during the first 24 h of incubation. Ent. caudatum counts were also positively correlated (r ≥ 0.57) with the abundance of the three quantified bacterial species. The Ec + AAFB co-culture had a higher ammonia concentration than the Ec culture, but both cultures had a similar abundance of the three quantified bacterial species. Therefore, uncultured or other AAFB populations may also contribute to the deamination activity observed in this study, as shown in another study [45].
CONCLUSION
Nitrogen utilization efficiency in ruminants is important for the economic viability of ruminant producers and the environment. The co-habitation and interactions of ruminal protozoa and AAFB contribute to the production of ammonia in the rumen. Our results verify that commensalism occurs between Entodinium caudatum and AAFB which benefits both microbial groups. The presence of Ent. caudatum in the cultures is also beneficial to HAB Prevotella ruminicola, Clostridium aminophilum, and Peptostreptococcus anaerobius. The abundance of C. aminophilum and P. anaerobius is correlated positively with ammonia concentration, verifying their role in ammoniagenesis. However, the prokaryotes that remained after washing the Ent. caudatum cells made it difficult to interpret some of the results. Understanding the interactions among the microbes involved in ruminal nitrogen metabolism remains challenging. Omics technologies, including genome-centric metagenomics, metatranscriptomics, and metabolomics, combined with stable isotope probing (SIP), should be used in future studies to help address this challenge.