INTRODUCTION
The number and variety of vibration applications in the medical treatment of blood flow have increased over the last decades. However, robust evidence on the significance of vibration effects (especially treatment effectiveness) is still lacking [1–5]. The treatment capabilities of the low power acoustic pulse therapy (APT) have been widely reported and is known to produce various responses in biological tissues, such as angiogenesis and anti-inflammatory effects [1], endorses the healing process in musculoskeletal diseases in humans [2], race horses [3], dogs [4] and partial-thickness wounds in piglets [5]. The low power acoustic pulse therapy pressure (10 ± 15 megapascal [MPa]) was shown to produce new blood vessels and improve tissue function with long term effects [1]. In race horses, low power APT improved lameness disorders such as insertional desmopathies, tendinopathy, osteoarthritis myopathies, arthrosis and podotrochlosis syndrome [2]. In dogs, APT was used for shoulder lameness, degenerative joint disease, Legg Calve Perthes disease (degeneration of the head of the femur bone in the hind leg) osteoarthritis and spondylosis [4]. The influence of shock waves on the reepithelialization of partial-thickness wounds was studied in Yorkshire piglets. The stimulating effect of low-energy shock waves coincides with significantly increased vascularization of the upper dermis and thicker layer of the newly formed epithelial cells covering the wound [5].
In 1996, Nakamura et al. [6] were the first researchers to claim that vibration used for research affects blood flow differently from vibration excited in the professional environment. The research showed that whole-body vibration influenced the blood flow in arteries. Most studies evaluated and analyzed the influence of high-frequency vibration on the human body, whereas only a few studies investigated low-frequency vibration effects [6–8]. Other researchers studied whole-body vibration effects on the blood flow in legs and found that the body was more sensitive to 5 Hz vibration than 50 Hz vibration. The influence of vibration intensity on the heart and blood vessel activity during physical workload was also proven [7,8]. Osawa and Oguma [9] found that whole-body vibration had a positive effect on arterial blood flow and other parameters of the circulatory system.
Many studies are investigating the effects of vibration on the whole body [7–9] however, there is a lack of studies on vibration effects on certain/specific body parts. The uniqueness of this study is that we have determined the influence of locally-generated low-frequency (15–60 Hz) mechanical oscillations on cow udder.
The known devices developed by foreign scientists for exciting the udder by the acoustic or electromagnetic waves of a particular frequency cause harmonic and directional oscillations of the frequencies directed to a target body area, e.g., to the udder, to be excited [10,11]. The device produces low power pulses with capability of deep tissue penetration that allows the pressure wave to be distributed over a large treatment area of the cow‘s udder capable of producing therapeutic effect [10–12].
Carefully selected physiologically resonant frequencies can both suppress disharmonious fluctuations that occur in affected cells during inflammation, and normalize metabolic processes, accelerating the transport of inflammatory products due to the known effect of increasing vascular permeability [13], while recording weak currents caused by potentials of the udder cells themselves [14,15].
The use of non-invasive methods ensures animal welfare and health, and the effects of acoustic low-frequency vibrations on the mammary glands are likely to stimulate blood circulation in cow udder and reduce the incidence of subclinical mastitis in dairy cows.
This is a novel pilot study, and of some interest to veterinary professionals working in the dairy sector. The objective of this study was to validate the operating principle, optimize the dimensions of the vibrating system, and investigate the dynamics of the 3D model of the cow udder, to investigate the impact of low-frequency oscillations on the udder and teats’ blood circulation by creating a mathematical model of mammary glands, using milkers and vibrators to analyze the theoretical dynamics of oscillations, to investigate the impact of low-frequency oscillations on the milk yield and quality parameters, and animal welfare.
MATERIALS AND METHODS
The experimental studies were implemented in three stages:
In the first stage, the analytical investigation and calculations were performed with the 3D dynamic model of the cow udder excited with an unbalanced rotating body during the automatic milking process (Fig. 1). The experiment aimed to validate the operating principle, optimize the dimensions of the vibrating system, and investigate the dynamics of the model.
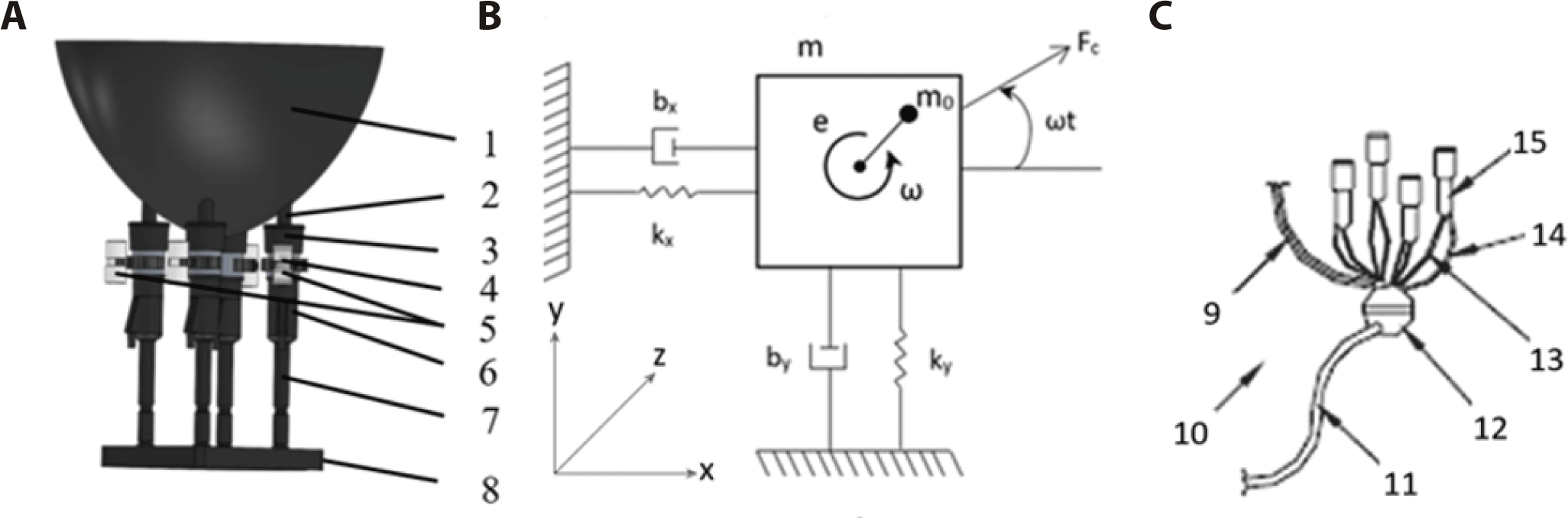
Then the experimental model of a cow’s artificial udder (natural rubber udder of cow-NRUC MODEL produced by Lithuanian University of Health Sciences [LSMU]) (Fig. 2) was developed. The udder was made from 3 mm thick rubber with four teats with holes. The artificial udder holds up to 20 litres of milk. The milking rack is designed to replicate a cow’s hind leg and consists of a frame with a cover plate between which the rubber udder is clamped.
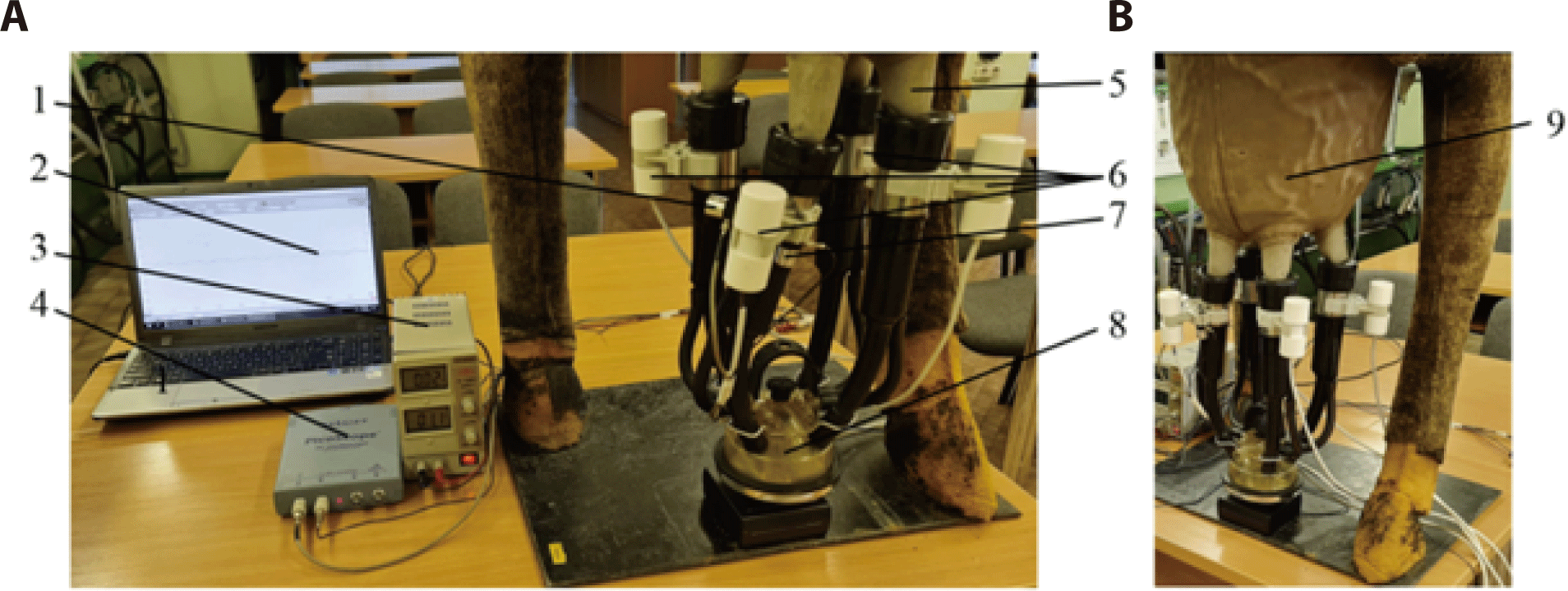
For evaluating the frequency response of the vibrating system, the studies were performed on the developed artificial cow’s udder with and without milk by applying unbalanced vibration motor oscillations in various directions of the artificial udder - in lateral (X-axis) and vertical (Y-axis), with four directions on a-X axis, b-Y axis, c-X axis, and b-Y axis (Fig. 2). More about the research methodology in the chapters “Mathematical dynamic model of an unbalanced rotor” and “Experimental tests in vitro”.
In the third stage, the application of the testing rig for experiments with a low-frequency harmonic oscillation device on a milking system (DeLaval Harmony, DeLaval, Tumba, Sweden) on a live animal was used. More about the research methodology in the chapter “Experimental tests in-vivo.” Invention: Cow Udder Stimulation System. Applicants: Kaunas University of Technology and Lithuanian University of Health Sciences. Date of submission of application No. 2021-542:12.8.2021.
The dynamic modeling of the udder excited with an unbalanced rotating body is based on the so-called Jeffcott model of an unbalanced rotor [16]. It presents a model and consists of an unbalanced rotor attached to the teat cup of the milking unit, of which one end is connected to a teat and the other – to a claw by a milk tube (Fig. 1A). The model has three degrees of freedom described by three differential equations of the second order. The rotor has only two degrees of freedom because an unbalanced vibratory motor is attached to the teat cup with the mass m as a mass point. It can move only in the plane perpendicular to the rotor’s axis. The dynamic model in Fig. 1B presents the vibratory motion of the mass m in the X-Y plane, and the horizontal Z-axis coincides with an unbalanced rotor’s axis. During rotation, the center of gravity moves along an orbit trajectory.
A simplified dynamical model of the milking unit with an unbalanced motor is presented in Figs. 1A and 1B. The model also contains flexural rigidity and damping of the milk hose and teat, which can be considered spring and damper producing vibration of/in the unbalanced rotor. In this way, the unbalanced vibratory motor is connected with a teat-cup attached to the udder and a claw through springs and dampers. The oscillations in the X and Y or Z directions are actuated by time-variable radial components of the rotating vector of harmonic force. It is a consequence of the unbalanced rotating rotor (Fig. 1B). The rotor axis is perpendicular to a rotating vector of the harmonic force Fc = moeω2 where mo and e are unbalance mass and eccentricity, respectively, and ω is the angular velocity of the rotor. αx, αy and αz are anglers between the axis of a rotor and the X-axis, Y-axis, and Z-axis, respectively.
Some parameters of the dynamical model were determined experimentally, and used in the theoretical investigation and calculations. These parameters were obtained using the DeLaval Harmony model milking unit. They are shown in Table 1.
Motion equations for the described substitute model are derived from the Newton’s Second Law:
where x, y, z – displacements in the X-axis, Y-axis and Z-axis, respectively; bx, by, bz – damping in the X-axis, Y-axis and Z-axis, respectively; kx, ky, kz– stiffness in the X-axis, Y-axis and Z-axis, respectively. For the centrifugal force Fc, caused by unbalance, we can derive:
In this case, we further analyzed a simplified dynamic model with oscillations in the X-axis and Y-axis. It was assumed that the Z-axis coincides with the axis of the unbalanced rotor, and motion in this axis is negligible compared to the motion in X-axis. The equation of motion in the X direction can be written as:
where m is the mass of the vibrating structure, b is the damping present in the system, and k is the system’s stiffness.
Steady-state amplitude X and phase angle due to vibration caused by rotating unbalance is given by
The dynamics in the Y-axis were investigated analytically using the same methodology with the stiffness and damping coefficients in the Y direction.
In the second stage, the experiment was conducted on an artificial cow udder model produced by LSMU to validate the proposed model. An unbalanced vibratory motor was made for the experimental research and applied to the DeLaval Harmony model milking unit. Four vibrators were attached to each teat cup. Only one vibrator of four was powered during one measurement, and only one - was powered with different frequencies and amplitudes. Measurements were done on all four teats independently. Fig. 2 presents the testing rig setup for the excitation of low-frequency oscillations on the artificial cow udder model.
The frequency responses were measured with an empty artificial cow udder model, and then, to simulate natural udder conditions, the artificial udder model was filled with milk.
In the third stage, the same low-frequency oscillations (range 15–60 Hz) of the directional harmonic oscillation device were applied on the milkers (DeLaval Harmony model) and tested on the cow during the milking process (Fig. 3). Four vibrators were used on each teat cup, but only one was driven in the determined frequency range during measurement. Measurements were done on all four teats independently.
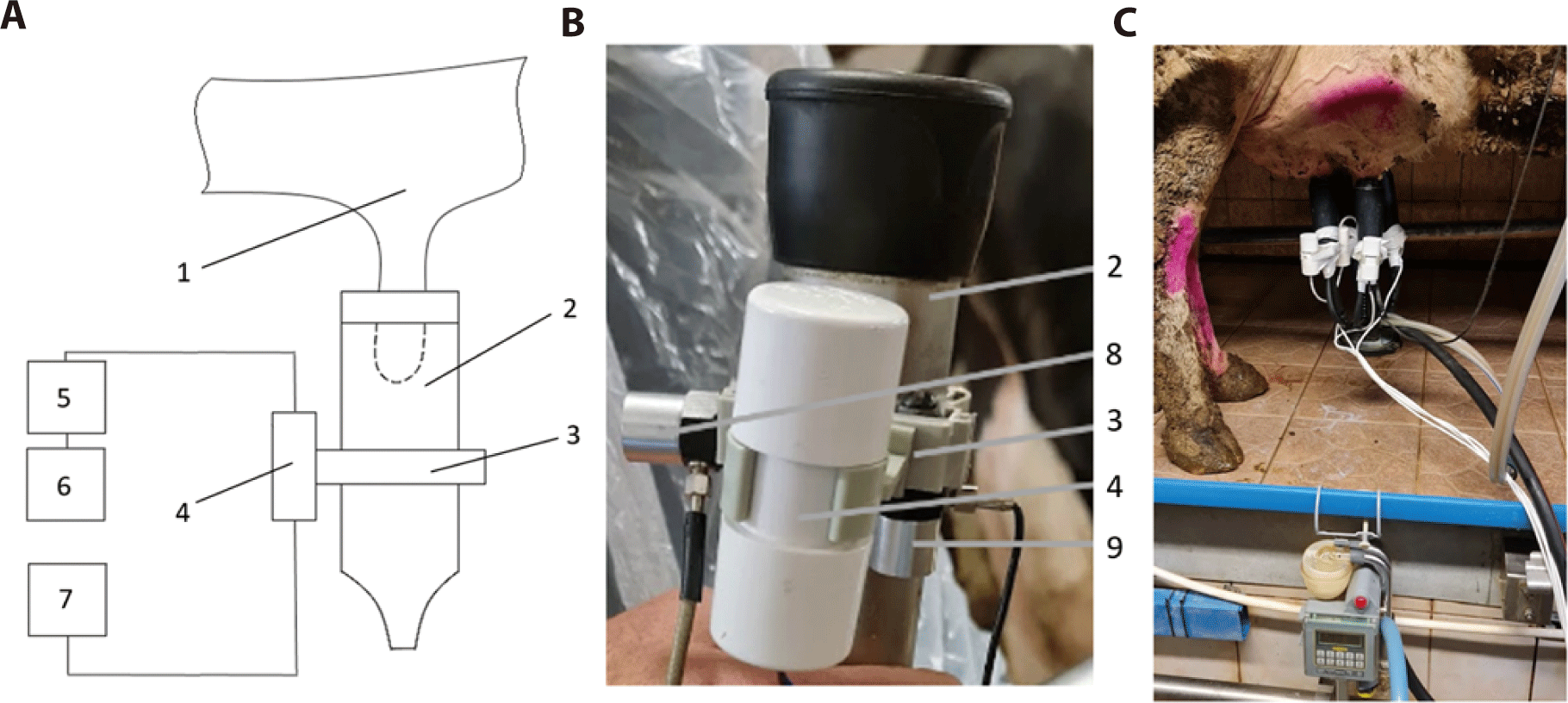
The power supply changed voltage with a step of 0.2V (1.2–3.8V), which resulted in the vibrator’s operating frequency change from 15 Hz to 60 Hz. The tests were performed using the accelerometers KD35 (RFT GmbH). Two single-axis accelerometers were used on the testing rig to measure the vibration simultaneously in the lateral (X) and vertical (Y) directions. All the vibration data was recorded with an oscilloscope and analyzed using PC.
The study was carried out on an organic dairy herd of 1,330 Holstein-Friesian cows in 2021. The dairy parlors were equipped with an online computerized DeLaval Herd milking system. About 14 thousand kg of milk was milked during one milking with an average milk yield of 22.9 ± 2.1 kg. Thirty multiparous lactating Holstein cows (mean 653.15 ± 100.24 kg of body weight, mean 137.93 ± 98.53 days in milk, mean 2.40 ± 1.40 of parities) were selected in the experiment. Cows were randomly assigned to control and experimental groups. The data collection period lasted for about two weeks. We analyzed all collected data on both, cows not treated with low-frequency vibration devices during milking (control group n = 15) and cows treated with low-frequency vibration devices during milking (experimental group n = 15). Milk yield was measured in real-time at each milking session by the automated milking system installed at the milking parlor. The cows were milked twice a day at 05:00 and 17:00 h in a milking parlor. The animals were kept in a loose housing system and were fed a feed ration throughout the year at the same time balanced according to their physiological needs. The composition and feeding value of diet for all cows were as follows: Dry matter (DM) content of silage 55.6%, Metabolic Energy 9.87 MJ/kg DM, net energy for lactation (NEL) 5.76 MJ/kg DM, crude protein 13.1% DM, crude fat 2.75% DM, crude fibre 26.8% DM, ash 8.6% DM. Cows had unlimited access to fresh water and were fed a total mixed ration (TMR) ad libitum.
During the experiment in vivo, we analyzed the effect of low-frequency vibrations and evaluated daily milk yield, milk composition and quality, udder surface temperature, and dairy cows’ welfare. Milk samples (n = 60) were collected from each cow for the milk’s composition and quality analysis. For determining somatic cell count, the milk samples (50 mL) were preserved with bronopol (2-bromo-2-nitropropane-1,3-diol and 2-bromo-2-nitropropanol) in micro tabs and analyzed by the flow cytometric analysis method using a Somascope cell counter (Foss, Hillerød, Denmark), according to the LST EN ISO 13366-1:2008+AC:2009 microscopic method standard. The lactose, fat, urea and milk protein levels were determined using the spectrophotometric method with infrared meter LactoScopeFTIR (FT 1.0. 2001, Delta Instruments, Drachten, Netherlands).
Thermography provides valuable information for identifying simple thermal patterns resulting from changes in blood flow, which can be used to detect the inflammation or pathology presence in a target area. In this study, we used an infrared thermography camera T450sc (FLIR Systems, Wilsonville, OR, USA) to determine the skin surface temperature changes in the mammary gland. The thermal image was captured when the entire test area (hindquarters of the udder) was visible on a camera screen. The average temperature of the mammary gland test surface was determined from the selected points in the thermogram.
The comparative analysis of frequency-amplitude characteristics in the lateral (X) and vertical (Y) directions showed the higher amplitude of vibrations at the 25 Hz frequency, which coincided with the resonance frequency in the lateral (X) direction. It was selected as the operating frequency for the thermography tests. The temperature measurements were taken using the 25 Hz frequency vibrations before and during the milking process.
The cow’s grimace scale was assessed before and during the milking process. Cows’ welfare was calculated by applying the cow pain scale. For this purpose, cow discomfort was analyzed by facial expression, ear-head-eyes-nose position score-scale (0–2) adapted by Gleerup et al. [18,19]. Moreover, back position evaluation was determined where pain scale score meanings were as follows:
Facial expression: 0 - attentive, neutral look, focused on a task, e.g., eating, ruminating, sleeping; 1,2 - strained, tense/worried face, furrows above, eyes and wrinkles above the nostrils.
Ear position: 0 - both ears forward/one ear forward or back and the other listening; 1- ears back/asymmetric ear movements, both ears back/moving in different directions; 2 - both ears to the sides and lower than usual, pinna facing slightly down.
Head position: 0 - held high; 1- lower than withers; 2 - very low.
Nose: nostrils dilatated; facial muscles relaxed, not in pain (1,2).
Eyes: 0 - clear eyes, bright, healthy/no pain; 1,2 - tense muscles above the eyes/in pain.
Back position: 0 - normal; 1- slightly arched back; 2 - arched back.
For the theoretical equations, we used an analytical method. For the result analysis in vivo we used R statistical software (v. 4.1.2). In terms of animal welfare, Pearson’s χ2 test was used to compare the observed cows’ emotional body responses. One-way ANOVA and t-test were used to analyze milk yield, quality, and udder temperature. Significance was declared at p ≤ 0.05 throughout this study. Data are presented as means and SD.
RESULTS
To determine amplitude-frequency characteristics of the udder model filled with milk and without milk, theoretical and live animals were used. The results were obtained in two X (lateral) and Y (vertical) axes directions (Fig. 4). Milkers were applied on all four teats, but only one teat was excited during one vibration session. The resonance frequency was about 22.5–26.5 Hz on X-axis and Y-axis - about 37.5–41 Hz (Table 2). The experimental udder model filled with milk showed higher frequency values than the model without milk 6% at the lateral (X) direction and 8.5% at the vertical (Y) direction.
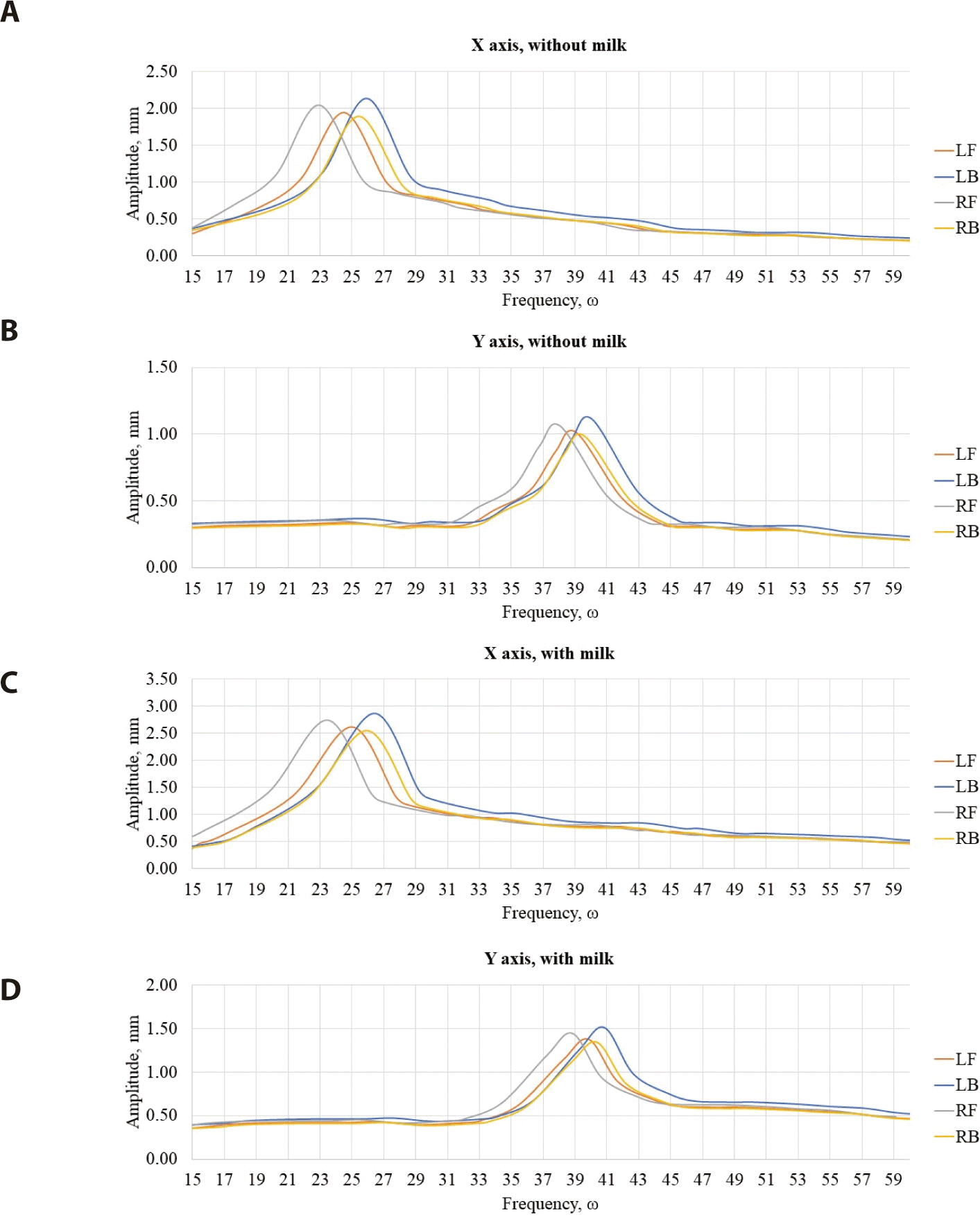
X axis | Y axis | |
---|---|---|
Stand with the milk | 24.97 | 39.86 |
Stand without the milk | 24.48 | 39.05 |
Alive animal (cow) | 24.33 | 39.63 |
Theoretical calculations | 25 | 39 |
We can confirm that the frequencies on a live animal are nearly the same as on the model or in the theoretical calculations. The result was such because the cow udder was not affected by the resonance frequency system; only the structure of the milker and its rubber parts mainly were excited by the oscillations. The results are shown in Fig. 5.
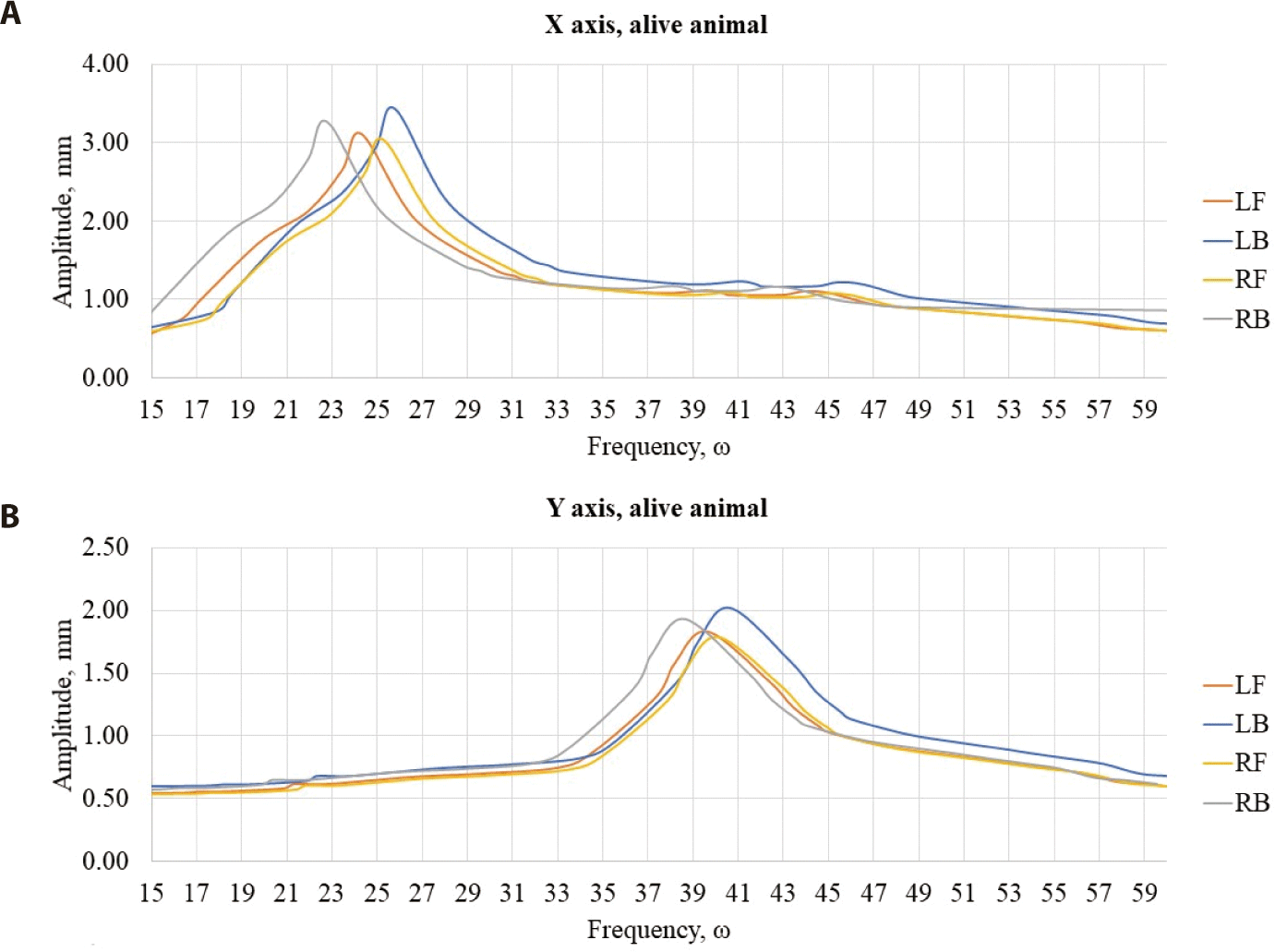
Also, using theoretical calculations, we get nearly the same results as in the experiments, and the character of the curves is mostly the same. It is shown in Fig. 6.
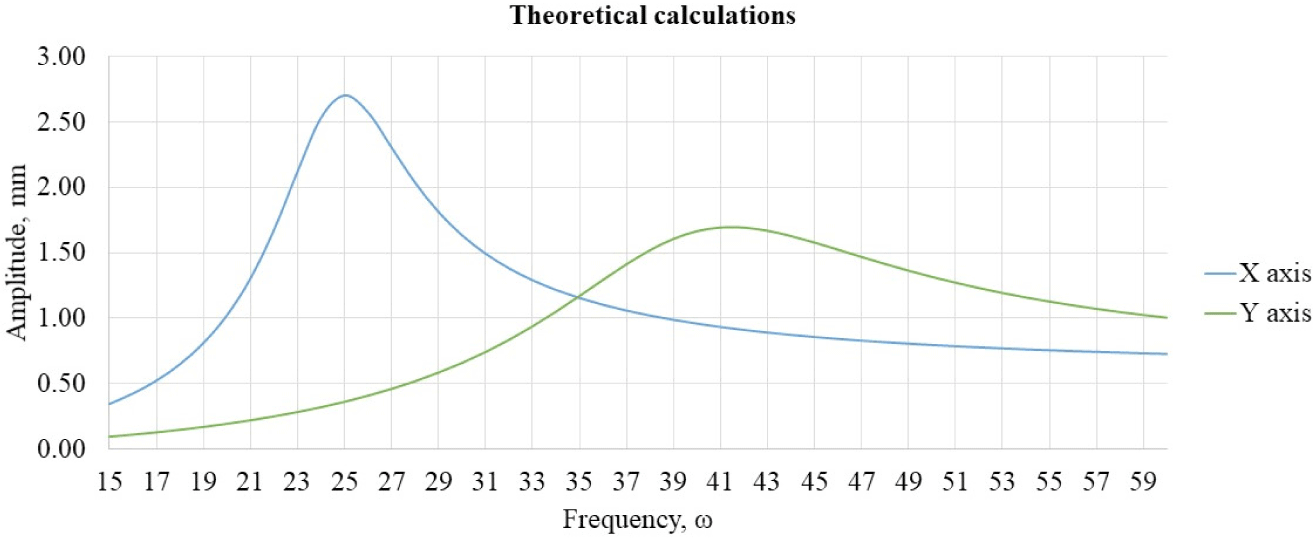
We get a higher resonance frequency of 39.00–39.86 Hz (Table 2) on the Y-axis because the stiffness of the milker is higher on this axis.
The theoretical and experimental results show that the amplitudes on the X-axis are much higher (about 50%–70%) than on Y-axis. The amplitudes on a live cow are higher in both axes than in other results.
The mechanical vibrations, excited by the vibrator in the milker during the milking process of live animals, propagate through the teats to the udder and produce a physiotherapeutic effect by increasing the udder surface temperature. The obtained results showed that the surface temperature of the udder during milking of the cows in the experimental group increased by 1.1°C compared to the surface temperature of the udder before milking. Meanwhile, the surface temperature of the udder at the end of milking of the control group cows increased by 0.53°C compared to the surface temperature of the udder before milking. The data of temperature studies showed (Fig. 7) that mechanical vibrations during milking induced a more intense increase in the surface temperature of the udder (0.57°C [p < 0.001]) compared to the temperature before milking.
In the present study using the new device and applying the mechanical vibration of low frequency (25 Hz), we noticed that cows’ facial expression, the position of eyes, ears, head, back, and tense of nostrils did not change (Table 3). Low-frequency vibration did not adversely affect any animal welfare parameters, including the cows’ emotional state.
The effect of low-frequency vibrations on the milk yield, milk composition, and quality was analyzed. The study results revealed that the average somatic cell count and urea values of milk had increased by 3.5% and 2.88%, respectively, compared to the milk not affected by low-frequency vibrations, but this difference was not significant. Mechanical vibrations also had no significant effect on the milk yield and quality parameters mean values (Table 4).
DISCUSSION
The researchers interested in physiological animal body responses to vibration have had one primary concern - the possibility to improve peripheral blood flow without pharmacological intervention [1,10–12].
Cows are usually milked for 4–8 minutes, but the duration of milking and the rate at which milk is delivered are highly dependent on the preparation of the udder, the milk yield, the individual characteristics of the cow, the construction and the technical condition of the milkers [20,21]. Many studies have shown that the amount of milk and the duration of milking depend on the position of the udder quarters [22,23]. After analyzing all previous studies, we chose to apply the 8 min long vibration as this time was the average milking time of the whole cow herd.
The results showed that using vibrations of 25 Hz frequency for up to 8 minutes (as long as the milking process takes) increased the udder surface temperature by 0.57°C, thus confirming that low-frequency vibrations stimulate and activate blood flow to the cow’s udder [24]. The conducted research showed that whole-body vibration influenced the blood flow in arteries [6,25,26]. However, such studies were meant to evaluate the influence of high frequency vibration on the human body, and only a few studies described low frequency vibration effects. Other authors, who have investigated whole-body vibration effects on the blood flow in legs, claim that the body is more sensitive to 5 Hz vibration than to 50 Hz vibration [7].
In this study a vibration system to excite the cow’s udder has been analyzed theoretically and developed for experimental research. Four unbalanced motor vibrators were attached to each teat cup of the milker (DeLaval Harmony model), and the low-frequency range vibrations were induced in the cow udder. The 2D dynamic model of the vibration system has been established, and the frequency response of the vibrator-milker assembly has been solved analytically and verified experimentally. A frequency sweep test was performed on the developed vibration system in the frequency range of 15–60 Hz. The calculated and experimentally obtained frequency responses of the vibrations in lateral and vertical directions are close. The test values of the first-order effective frequency of the vibration system in the lateral (X) direction are 23.5–26.5 Hz, the effective frequency of the simulation analysis is 25.0 Hz, and the relative error is 6%. The test values of the first-order effective frequency of the vibration system in the vertical (Y) direction are 37.5–41.5 Hz, the effective frequency of the simulation analysis - 41.0 Hz, and the relative error - 8.5%. The operational frequency of 25 Hz was defined for a developed vibration system, at which higher amplitude and lower damping of vibrations were gained.
An indicator of the healthy udder of a cow is the components of the milk secretion of the udder: the number of somatic cells, organoleptic components (milk uniformity, and other indicators) [11].
This study analyzed the effect of low-frequency vibrations excited on the cow udder during the milking process. Also, we have evaluated some parameters such as milk yield, composition and quality, temperature, and welfare of dairy cows.
One more Acoustic Pulse Therapy device was designed to produce deep penetrating acoustic pulses that are distributed over a large treated area at a therapeutic level [12]. At the experiment daily milk yields of the treated cows increased significantly (p < 0.05) and the percentage of cows with log somatic cell count under 5.6 cells/mL was significantly higher (p < 0.001). Milk of the infected quarters appeared normal with lactose greater than 4.8%, but this difference was not significant [10]. Low-frequency vibrations had no statistically significant effect on the mean values of milk yield, milk composition, and quality in our study.
Welfare has been evaluated on cows under low-frequency vibration during milking. As a cow’s face reflects an emotional state, the cow’s facial expressions can demonstrate pain and sickness even in the early stages. The facial expression should always be observed when animals are disturbed as it can impact animal welfare, milk production, and reproductive efficiency. Moreover, dairy cows’ ear postures are reliable indicators of low arousal and/or positive emotional state [27].
Facial expression is a pain recognition tool in animals, and new technology application has been associated with animal welfare on farms. On-farm use of the facial expression to recognize pain in cattle may override some of the difficulties in animal pain diagnosis and, consequently, allow for rapid and consistent intervention to alleviate suffering [28,29]. Many studies have focused on eye white and ear posture as potential promising indicators for interpreting emotions in dairy cows [30]. Researchers now globally recognize that emotions are part of the complex life of dairy cows [31].
Our study results demonstrate that applying low-frequency oscillations on the mammary gland does not change a cow’s facial expression, eye, and ear postures and does not adversely affect cow’s welfare.
CONCLUSION
The mechanical vibration device developed and tested in the study was mounted on a DeLaval milking machine, which excited the udder with low-frequency oscillations. It allowed the analysis of input parameters (temperature, oscillation amplitude) and, using feedback data, changing the device parameters such as vibration frequency and duration.
Applying low-frequency (X-axes – 25.0; Y-axes – 41.0 Hz) vibration on cow’s udder during the automatic milking statistically significantly increased udder temperature by 0.57°C (p < 0.001) and did not adversely affect the studied animal welfare indicators, milk yield and quality parameters.
The perspective is to carry out experiments on a larger number of cows and to confirm our hypotheses regarding animal welfare, prevention and treatment of mastitis, and increasing the amount of milk produced.
Further studies will be carried out in the following research phase on clinical and subclinical mastitis cows.