INTRODUCTION
Korea is a peninsula surrounded by the sea on three sides with hot and humid summers, experiencing an average temperature of 24.4°C (mean minimum temperature of 20.7°C, and mean maximum temperature of 29.1°C) and relative humidity (RH) of 77.3% from 2011 to 2021 [1]. In general, beef cattle require more energy due to the increased maintenance energy requirement for thermo-emission, while the energy intake decreases with diminished feed intake during heat stress (HS) [2]. Energy for beef cattle is important because limited energy causes reduced growth performances and productivity. Under HS conditions, insulin secretion increases, resulting in increased glucose absorption in cells and inhibited lipolysis in fat tissue compared with thermoneutral conditions. In addition, insulin inhibits beta-oxidation, which synthesizes energy using fat and increases the availability of monosaccharide glucose and amino acids (AAs) [3,4]. Additionally, protein metabolism is suppressed under HS. As the phenomena continue, first, blood flows into peripheral tissues to dissipate heat, the blood flow to digestive organs reduces, and the absorption of nutrition, including AAs, also reduces [5]. Second, HS inhibits ammonia utilization of the ruminal microbes and reduces the proliferation of ruminal microbes, which eventually results in a decline in ruminal microbial protein synthesis [6,7]. Third, the production of heat shock protein (HSP) 70, which is a protein that prevents the denaturation of other proteins in HS, increases by 200 times in lymphocytes due to HS [8]. Finally, the amount of glucogenic AAs in the blood reduces by approximately 17.1% followed by the production of glucose due to the negative energy balance caused by HS [9]. However, supplementing heat stressed cows with a high dosage of dietary protein could reduce productivity due to an excessive urea cycle [10], thus the optimum dosage, or its component (AA), should be identified with regard to the different physiological effects.
Glutamine (Gln) is a conditionally essential AA in the body of ruminants associated with normal physiological functions, lipid and protein metabolism, and muscle growth [11–13]. Gln is also responsible for various functions in the body including: 1) synthesizing other AAs using Gln; 2) increasing immunity with a proliferation of lymphocyte and increasing-decreasing cytokines; and 3) using respiratory fuel in the mitochondria of intestinal epithelial cells and myocytes [14,15]. Our hypothesis is that the supplementation of glutamine will reduce HS and improve immunity in a lack of energy and protein supply due to reduced intake during HS, and furthermore, it has a positive effect on genes related muscle development.
A previous study in our laboratory revealed that in vitro supplementation with Gln at 0.5% has positive effects on fermentation end products (VFAs) and on the degradability of nutrients without adverse effects on ammonia-N or total gas production compared with 2% and 3% supplementation [16]. However, in vivo studies investigating the effects of Gln supplementation on beef cattle under HS conditions are scant. Moreover, the possible effects of Gln on the prevention of excessive nitrogen sourced from dietary nitrogen in Hanwoo steers under HS has yet to be investigated. Therefore, and following the previous in vitro study in our laboratory [16], the objective of this study was to explore the effects of Gln supplementation on growth performance, physiological traits, HSPs, and gene expression related to muscle development in Hanwoo steers under HS conditions.
MATERIALS AND METHODS
All experimental procedures were followed according to the Konkuk University “Guidelines for the Care and Use of Experimental Animals” (Approval no: KU20001). Originally, eight Korean native beef cattle were included in this study. The animals (initial body weight [BW] = 570.7 kg [SD = 43.6], months of age = 22.3 [SD = 0.88]) were randomly separated into two groups (supplemented Gln group, n = 4; control group, n = 4).
The diets were supplied with a commercial concentration (Hanwoo-love-max, Cargill, Seongnam, Korea) (1.5% of BW as-fed basis) and 1.5 kg of rice straw (as-fed basis) (Table 1). The animals were fed quantitatively according to 1.5% of BW at 08:00 and 16:00 h. The feed residual was measured daily at 07:00 h. BW was measured at 0, 3, 6, and 10 weeks. After evaluating BW, the amount of feeding was reset based on the measured BW. Water was available ad libitum. In the treatment group, an additional average of 51.45 g Gln/day/head (SD = 3.41, Daesang, Seoul, Korea) was supplied via oral administration to each animal for 9 weeks, except for an adaptation period of 1 week. The experiment period was from 27 June to 13 September, 2020, although the summer period in Korea starts from 31 May and ends in September (approximately 4 months) [17]. The amount of Gln fed to the animals was 0.5% of the total diet on an as-fed basis. According to a previous study, the addition of more than 2% Gln showed negative effects on rumen fermentation and a degradability of nutrients in-vitro; however, Gln at an amount of 0.5% of the total diet improved rumen fermentation and the degradability of nutrients [16]. The steers were housed in the same individual 5 m2 pens (2 m [length] × 2.5 m [breadth]).
2) Calculated value using NRC [18] equation is 100 - (CP + EE + NDF - NDIP + ASH).
3) Calculated value using NRC [18] equation is truly digestible (td)NFC + tdCP + (tdFA × 2.25) + tdNDF -7.
4) Calculated value using NRC [19] equation is 0.04409 × TDN.
Two experimenters visually checked the behavior parameters from 10:00 to 18:00 h four times at 0, 3, 6, and 10 weeks. The frequency of lying and standing at 0, 3, 6, and 10 weeks was calculated from 10:00 to 18:00 h. The rectal temperature (RT) was checked for four times at 0, 3, 6, 10 weeks at 14:00 h.
The THI was recorded inside and outside the barn at 1 second intervals using a sensor (MHB-382SD, ZL 2008 2, Lutron, Shanghai, China), and the daily average (00:00 to 24:00) values of ambient temperature and RH were calculated. The THI was calculated based on dry bulb temperature (Tdb) and RH using the following formula: THI = (1.8 × Tdb + 32) – [(0.55 – 0.0055 × RH) × (1.8 × Tdb – 26.8)], in accordance with a previous study [18]. The daily THI data are presented in Fig. 1.
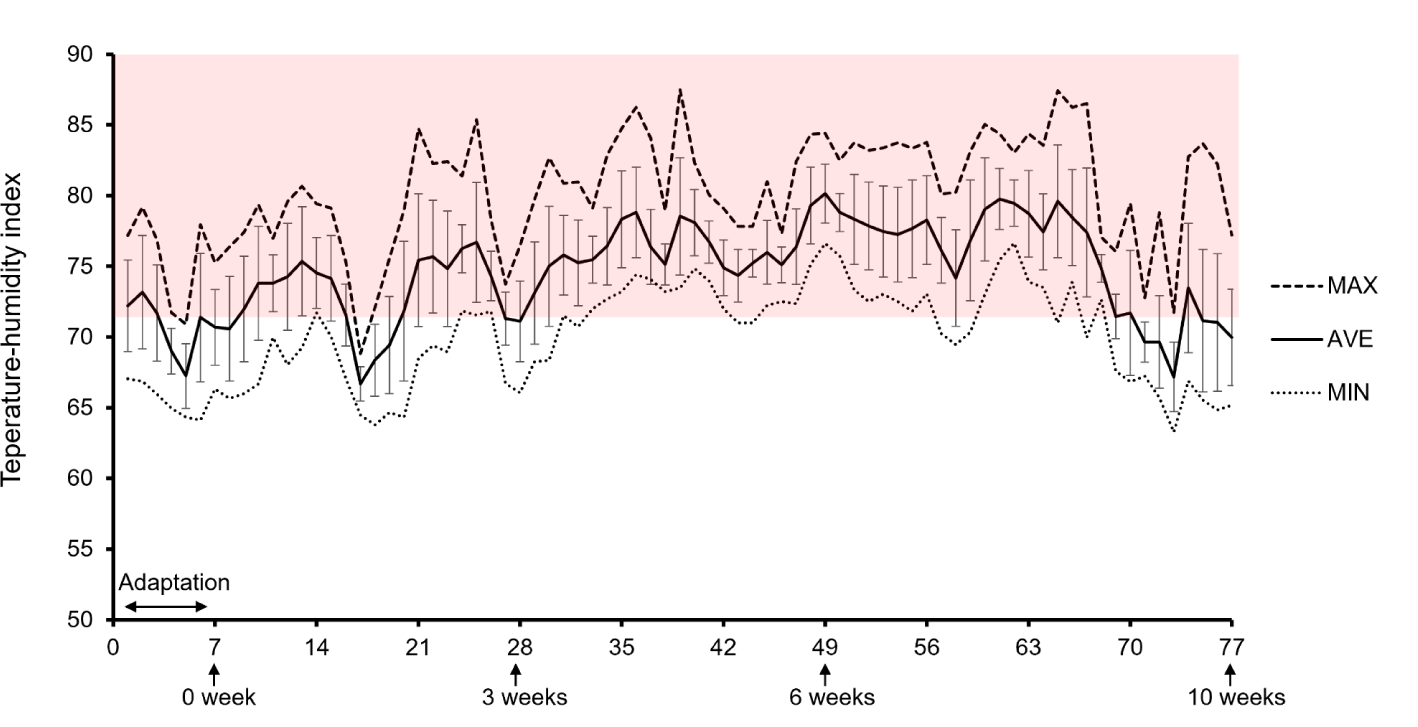
Blood samples were collected from the jugular vein of each beef using 18-gauge needles at 08:00 h at 0, 3, 6, and 10 weeks. Serum tubes, K2 EDTA (K2E) 7.2 mg Plus tubes (BD Vacutainer, Franklin Lakes, NJ, USA), and sodium heparin (10 IU/mL) (BD Vacutainer) were prepared, and the collected blood samples were stored on ice before being transferred to the laboratory, except for heparin-treated blood samples, which were used to isolate peripheral blood mononuclear cells (PBMCs). Next, to separate the serum and plasma, the blood samples were centrifuged at 2,740×g for 15 min at 4°C. The serum and plasma were then transferred to a 1.5 mL tube and stored in a freezer (–80°C) before further analysis. The K2 EDTA blood samples were temporarily stored at 4°C for subsequent analysis.
Analytical reagents for measuring blood urea nitrogen, glucose, total protein, albumin, globulin, and creatine phosphokinase levels were purchased from Fujifilm healthcare (Tokyo, Japan). All these parameters were analyzed using a biochemistry analyzer (Fuji Dri Chem 7000i, Fujifilm).
Blood AAs were performed as described in the AA analyzer manual (Sykam 433, Sykam, Eresing, Germany). Briefly, 200 µL of plasma was deproteinized with an equal volume of 10% (w/v) sulfosalicylic acid and incubated for 30 min at 4°C, then centrifuged at 20,800×g for 3 min at room temperature. The supernatant fluid was filtered using a 0.2 µm nylon filter. The AAs were measured photometrically at 570 nm. The run time for each sample was 130 min.
The isolation of PBMCs was performed with a minor modification to the previously described method [21]. Blood samples were stored at room temperature and transferred to the laboratory for blood separation and further analyses. For the isolation of PBMCs, the blood samples were processed within 8 h of the sample collection. Density gradient centrifugation was used to separate PBMCs from the whole blood. The whole blood was diluted 1:1 with 1 × phosphate-buffered saline (PBS; Hyclone, Laboratories, Logan, UT, USA) and layered gently over Histopaque-1077 (Sigma-Aldrich, St. Louis, MO, USA). All the PBMC isolation steps were performed at room temperature as per the manufacturer’s instructions. The isolated PBMCs were washed twice with 1 × PBS. TRIzol Reagent (Life Technologies, Seoul, Korea) was then added to the pellet of PBMCs, and it was stored at –80°C until RNA extraction.
The collection of hair follicles was performed according to the previously described method [22]. Tail hair (25 to 30 hairs) was pulled from each steer at 08:00 h. Individual hairs were grasped as close to the skin as possible and then rapidly pulled out. The hair follicles were washed using diethylpyrocarbonate (DEPC)-treated water. After the follicles were washed, the bottom centimeter of each hair, containing the hair follicle, was cut, and placed in a 5 mL specimen jar filled with RNAlater™ (Ambion, Austin, TX, USA). The samples were stored at room temperature for 1 to 14 days until RNA extraction. For RNA extraction, only the hair follicle was cut and placed in 1 mL of TRIzol, then finely ground with a homogenizer.
Longissimus dorsi muscle sampling and total RNA extraction were performed by surgical biopsy according to previously described methods minor modifications [23]. Tissue samples (approximately 2 g) were collected from the longissimus dorsi muscles of the steers (n = 8) via biopsy at week 10 of the experiment. The biopsy procedure followed the Konkuk University “Guidelines for the Care and Use of Experimental Animals”. Briefly, local anesthetic agents were administered at 6 equidistant points around the resection area, namely, the longissimus dorsi muscle at positions 12 to 13 of the ribs. The veterinarian resected 5 cm of skin in the direction of the muscle and then dissected the longissimus dorsi muscle tissue using surgical equipment. The collected tissue was immediately washed with DEPC-treated distilled water (diethylpyrocarbonate, Sigma-Aldrich, Seoul, Korea), placed in liquid nitrogen until completely frozen, and placed on dry ice until transferal to the laboratory. The tissue samples were then ground into powder in liquid nitrogen. Total RNA was extracted using TRIzol Reagent. An amount of 0.1 g of tissue sample was mixed with 1 mL of TRIzol. Next, the sample was homogenized using a homogenizer (IKA T10 basic, IKA, Seoul, Korea). The muscle tissue was stored at –80°C until RNA extraction.
The method used for extracting total RNA from the PBMCs, hair follicles, and muscle tissue were the same. The samples were centrifuged at 4°C and 12,000×g for 10 min; then, the upper aqueous phase was transferred into a new 1.5 mL tube. Next, 200 μL of chloroform was added, and the mixture was incubated for 2 min at room temperature after vortexing, followed by centrifugation at 12,000×g for 15 min at 4°C. The upper transparent aqueous phase was transferred into a new 1.5 mL tube. Isopropanol (500 μL) was added, and the sample was incubated for 10 min at room temperature after vortexing, followed by centrifugation at 12,000×g for 10 min at 4°C to precipitate the RNA. The supernatant was then discarded, and the RNA was washed by adding 1 mL of 75% ethanol to the tube. After vortexing, the mixture was centrifuged at 4°C and 12,000×g for 10 min. To collect the RNA, the wash step was repeated with 1 mL of 100% ethanol following the same procedure. After washing, the extracted RNA was dried under a vacuum for approximately 15 min, redissolved in 30 μL of DEPC-treated distilled water, and then incubated at 60°C for 10 min. The RNA concentration was determined using spectrophotometric analysis (NanoDrop 1000, Thermo Scientific, Seoul, Korea).
In the muscle tissue, fatty acid–binding protein 4 (FABP4), glycerophosphate dehydrogenase (GDP), heat shock protein beta 1 (HSPB1, lipoprotein lipase (LPL), myoblast determination (MyoD), myogenic factor 5 (MYF5), myogenic factor 6 (MYF6), myogenin (MyoG), peroxisome proliferator-activated receptor-gamma (PPARγ), stearoyl-CoA desaturase (SCD), 18S ribosomal RNA (18S), glyceraldehyde-3-phosphate dehydrogenase (GAPDH), and ribosomal protein lateral stalk subunit P0 (RPLP0) primers were designed using the National Center for Biotechnology Information (NCBI) Primer-BLAST (Table 2). In the PBMCs, HSP70, HSP90, beta-2-microglobulin (B2M), and ribosomal protein S15a (RPS15A) primers were also designed using the NCBI Primer-BLAST (Table 2). In the hair follicle, we used HSP70, HSP90, and GAPDH designed above.
To synthesize cDNA, 1 µg of RNA was reverse transcribed in a 100 μL reaction volume with an iScriptTM cDNA Synthesis Kit (Bio-Rad, Hercules, CA, USA) according to the manufacturer’s protocol. Quantitative real-time polymerase chain reaction (qPCR) was performed on duplicate samples by using a CFX ConnectTM Real-Time System (Bio-Rad, Seoul, Korea) with IQTM SYBR Green Supermix reagents (Bio-Rad, Seoul, Korea). The following PCR conditions were used: 95°C for 3 min and 40 cycles at 95°C for 10 s, 51°C–65°C for 30 s and 72°C for 30 s. The threshold cycles for each sample were normalized to housekeeping genes (muscle tissue: RPLP0, GAPDH, 18S; PBMCs: B2M, RPS15A; hair follicle: GAPDH). The relative expression of the target gene was quantified as the fold change of the expression of the target gene relative to the expression of the thermoneutral control according to the 2-ΔΔrCT method [24].
Growth performance, complete blood cell count, blood metabolite, and blood AA were analyzed using SAS 9.4 Proc Mixed and repeated-measures analysis (SAS Institute, Cary, NC, USA). The model used was as follows:
where Yijk is the observation of Hanwoo steers k at sampling time j for given treatment i; μ represents the overall mean, αi denotes the fixed effect of treatment i (control and Gln supplementation group); γ(α)ijk is the random effect of Hanwoo steers k nested in treatment i, and sampling time j (3, 6, 10 weeks, or every day); and εijk represents the residual effect. Covariance structures (autoregressive order 1 and unstructured) for the repeated measures model were tested. The structure that best fit the model was chosen based on the smallest value of Schwarz’s Bayesian information criterion. The first day of sampling in the adaptation period was included as a covariate to correct the means. The covariate factor was included in the model when appropriate but was removed from the model when it was insignificant. Data are presented as least square means and associated with standard errors.
Data of behavior, RT, and mRNA expression from each sample including muscle, PBMCs, and hair follicle, were assessed using independent (unpaired) sample t-tests for unequal variances. The model used was as follows:
where Yik represents the observation of Hanwoo steers k for given treatment i; μ is the overall mean; bi denotes the fixed effect of treatment i (control and Gln supplementation group); and εik is the residual effect.
Data of behavior, RT, and mRNA expression from the PBMCs and hair follicles were determined using the one-way ANOVA procedure with Tukey’s test. The model used was as follows:
where Yik represents the observation of Hanwoo steers k for given sampling times i; μ is the overall mean; δi was the fixed effect of sampling times i (3, 6, and 10 weeks); and εik stands for the residual effect. Differences observed between means were considered significant at p < 0.05, and the tendency was declared at 0.05 ≤ p < 0.1.
RESULTS AND DISCUSSION
For this study, which was conducted at the hottest time of the year in Korea, 17 July to 31 August, the animals were under HS conditions, as revealed by an average temperature of 26.2°C (range 24.3°C–28.9°C), a RH of 84.0% (range 69.6%–93.7%), and an average THI of 76.9 (range 73.1–80.2) (Fig. 1). The average THI for the entire experiment was 74.6, the average maximum THI was 80, and the average minimum THI was 69.9 (Fig. 1). In the ambient temperature 25°C and RH 45%–50% (THI 72.8–73.3) which is upper critical temperature because Bos taurus increased slightly the respirations but remained RT and heat production in the THI [25]. Furthermore, fattening cows have difficultly dissipating heat because their surface area relative to weight becomes smaller, increasing the insulation effect by increasing the body condition score [2].
Based on the studies mentioned above, in this experiment, the Hanwoo steers experienced sufficient HS, and the RT measurements are shown in Fig. 2B. In Fig. 2A, the mean THI, maximum THI, and minimum THI were identified at the measuring time for RT and behavior. The average THI was maintained at approximately 75.1 until 67 days after the start of the experiment, and the average THI decreased over time (Fig. 1). Thus, the average RT of 38.6°C at 10 weeks was 0.26°C lower than the average RT at 0, 3, and 6 weeks. The difference in RT resulting from Gln supplementation was not shown (p > 0.05).
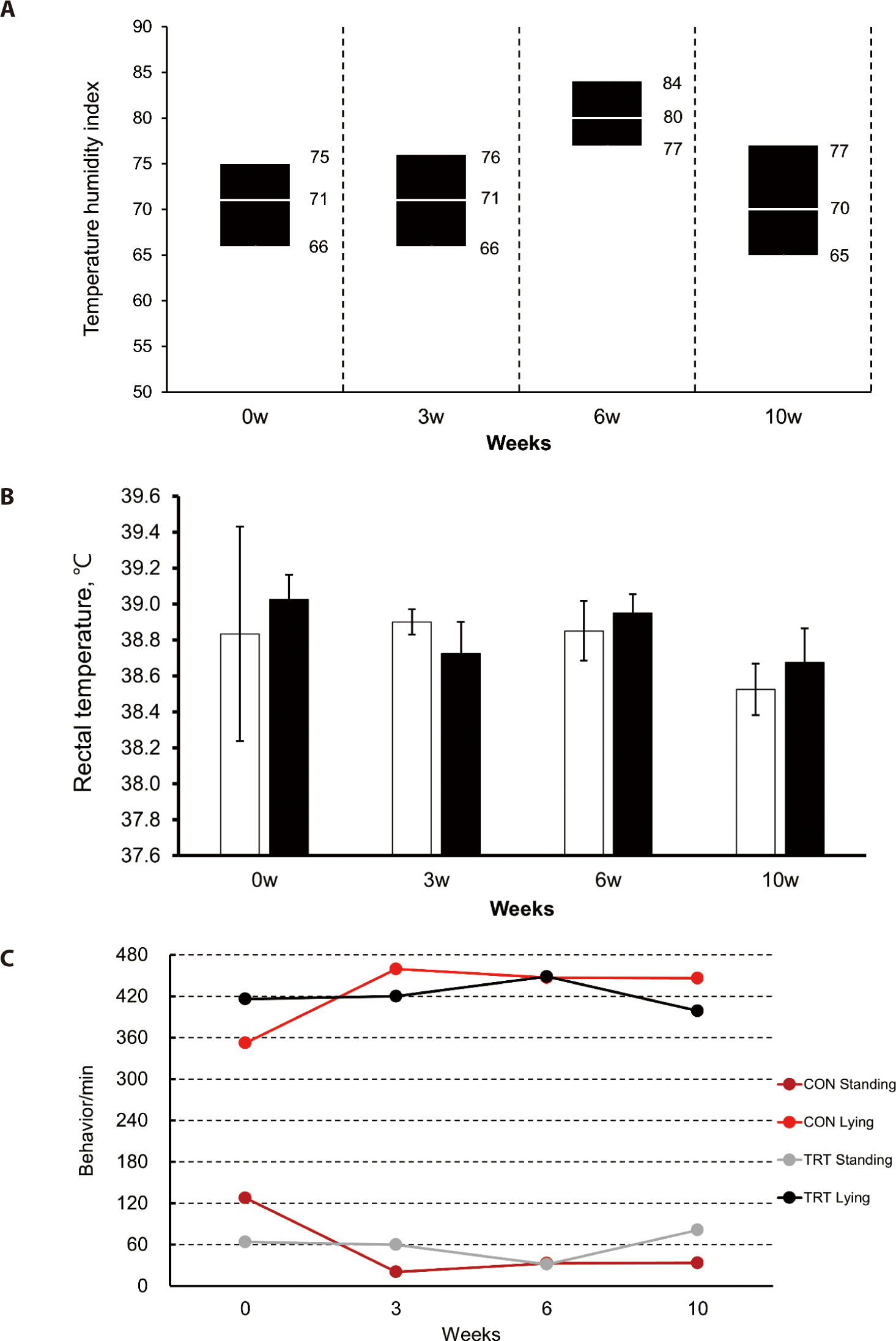
Through looking at standing behavior time and lying behavior time, we could confirm whether there was a difference in behavior according to Gln supplementation. The average standing time in the control group was 426.3 min/480 min, and in the treatment group, it was 420.8 min/480 min (Fig. 2C). Lying time in the control group was 53.8 min/480 min, and in the treatment group, it was 59.2 min/480min (Fig. 2C). Although Gln supplementation resulted in no difference in behavior (p > 0.05), the standing time was approximately 7.5 times longer than the lying time (from 10:00 to 18:00 h). This long standing time results in an increase in surface area contact with outside air to reflect heat in HS [26,27]. Consistently, Pinto and Hoffmann [28] reported that a longer standing time increases the efficiency of respiration in cows.
The tendency of L-citrulline and L-glutamic acid to increase compared with the control groups was confirmed by Gln supplementation under HS (p < 0.10). Blood glutamic acid (Glu) increased 2.27 times compared with the control group to show a concentration of 28.2 µmol/L (p = 0.083). Gln is transferred from the arterial blood to the inner cell. The Gln that enters the cell is converted into Glu by glutaminase, and Glu acts as an energy producer or intermediate, or is discharged into venous blood [29]. As a result, the amount of blood Glu increased in the 0.5% Gln supplemented group compared with the control group.
Blood L-citrulline increased 1.3 times compared with the control group to show a concentration of 30.2 µmol/L (p = 0.061). According to a previous study, approximately 6% of the carbon is used to form L-citrulline through Gln metabolism, and 34% of the nitrogen from Gln is used to produce L-citrulline [30]. Moreover, cyclical L-citrulline increases as the clearance of L-citrulline is reduced because Gln and L-citrulline share transporters [31].
HS reduced the feed intake but increased the maintenance energy requirement for sweating and panting to reduce body temperature [3]. Gln could be a respiratory fuel in immune cells, muscles, the liver, and digestive organs [13]. As Gln was used as energy, it was able to increase blood glucose by reducing the amount of use of blood glucose; however, there was no difference between the two groups (Table 3, p = 0.104). When multiparous Holstein cow duodenums were infused with Gln 300g/day, which amount of Gln was a higher amount of Gln than in this study, there were no differences in energy metabolisms such as glucose, non-esterified fatty acids, and beta-hydroxybutyrate [32].
There was no difference in the amount of blood urea nitrogen (p = 0.837) and creatine phosphokinase (p = 0.684) according to Gln supplementation. Previous studies have confirmed the increase in blood urea nitrogen due to Gln treatment. This study supplied Gln at approximately 51.45 g per day, which is less than Gln 300 g per day in previous studies [32,33].
Gln supplementation tended to reduce total protein in the blood (p = 0.097) and albumin (p = 0.059). The total protein decreased due to the decrease in albumin. Albumin has various functions, such as regulating the plasma oncotic pressure and transporting nutrients. Contrary to this study, a previous study found that albumin was increased as the formalin-treated Gln was increased by 150, 250, and 350 g per day [34]. Moreover, the intake of Gln increases albumin in situations where albumin reduced due to surgery [35]. Albumin concentrations decrease as albumin escapes into the urine as a result of renal difficulties, or or as albumin synthesis decreases due to liver disorders [36]. However, given the albumin concentrations in this study are within the normal range of 2.73 to 3.65 g/dL, they were not altered by renal or liver disorders [37]. The results of this experiment need further research to identify the effect of different ratios of Gln infusion or supplementation on blood metabolites and to warrant the obtained results.
To the best of our knowledge, there have been no experiments to confirm growth performances as a result of the addition of Gln to cows. Until now, there have been studies that provided Gln to cows to boost the immune system and milk production [32,34]. Even though there have been no previous studies on an improvement in growth performance with the addition of Gln in cows, it was confirmed that growth performance, with the addition of Gln, was improved under HS conditions in broilers [38,39]. We supplied Gln at 0.5% of the total diet (as-fed basis) for 9 weeks under HS conditions, but we observed no difference in feed intake, average daily gain, final BW, or gain-to-feed ratio (p > 0.05). The reason why there were no differences in growth performances could be explained by the following two speculations. First, the addition of 0.5% Gln in the presence of HS may be considered insufficient to indicate a change in growth performance. Second, because the purpose of this study was to focus on physiological phenomena during HS conditions, the experiment period was relatively short (10 weeks). A longer experiment period may result in the observation of significant changes in growth performance parameters, and this could be a hypothesis for further research. It is suggested that various amounts of Gln be supplied to cattle during HS to observe the possible positive effect on performance; however, the amount of supplementation should be limited to an amount of Gln lower than 2% of the total diet, due to possible toxic effects. This is because, if Gln supplementation exceeds 2% of the total diet, the ammonia concentration in rumen fluid exceeds the normal range [16].
Gln supplementation increased the number of white blood cell (WBC) by 1.26 times (p = 0.058), lymphocytes by 1.30 times (p = 0.068), and granulocytes by 1.28 times (p = 0.031), but the proportion decreased as it did not affect the number of monocytes (p = 0.058) (Table 4). HS reduced bovine lymphocyte proliferation because HS may impair the T helper cell 1 and T helper cell 2 balance [40]. However, Gln supplementation increased the lymphocyte and granulocyte under HS conditions. The first reason for this could be that Gln is utilized as energy sources for immune cells, especially lymphocytes [15]. The second reason could be that Gln can convert a substrate for nucleotide synthesis [14]. Finally, Gln stimulates cytokines including interferon γgene expression in the hair follicle was, Interleukin 2, interleukin 4, and interleukin 10, for direct or indirect lymphocyte proliferation [14].
WBC, white blood cell; LYM, lymphocyte; GRA, granulocyte; MON, monocyte; RBC, red blood cell; HGB, hemoglobin; HCT, hematocrit; MCV, mean corpuscular volume; MCHC, mean corpuscular hemoglobin concentration; MCH, mean corpuscular hemoglobin; RDW, red blood cell width; PLT, platelet; MPV, mean platelet volume; PCT, plateletcrit; PDW, platelet distribution width.
In addition, there were no differences in erythrocyte instruments and platelet instruments between the two groups (Table 5, p > 0.05).
At the cellular level, all animals respond to HS by synthesizing HSPs, which protect cells from heat-induced injury. HSP70 and HSP90 are molecular chaperones that protect cells from HS by refolding denatured proteins back into their correct conformations [41]. There were no differences in HSP70 and HSP90 expressions in PBMCs between the groups (p > 0.05, Figs. 3A and 3B). Only HSP70 tended to change in the control group according to the sampling weeks (p = 0.093, Fig. 2A).
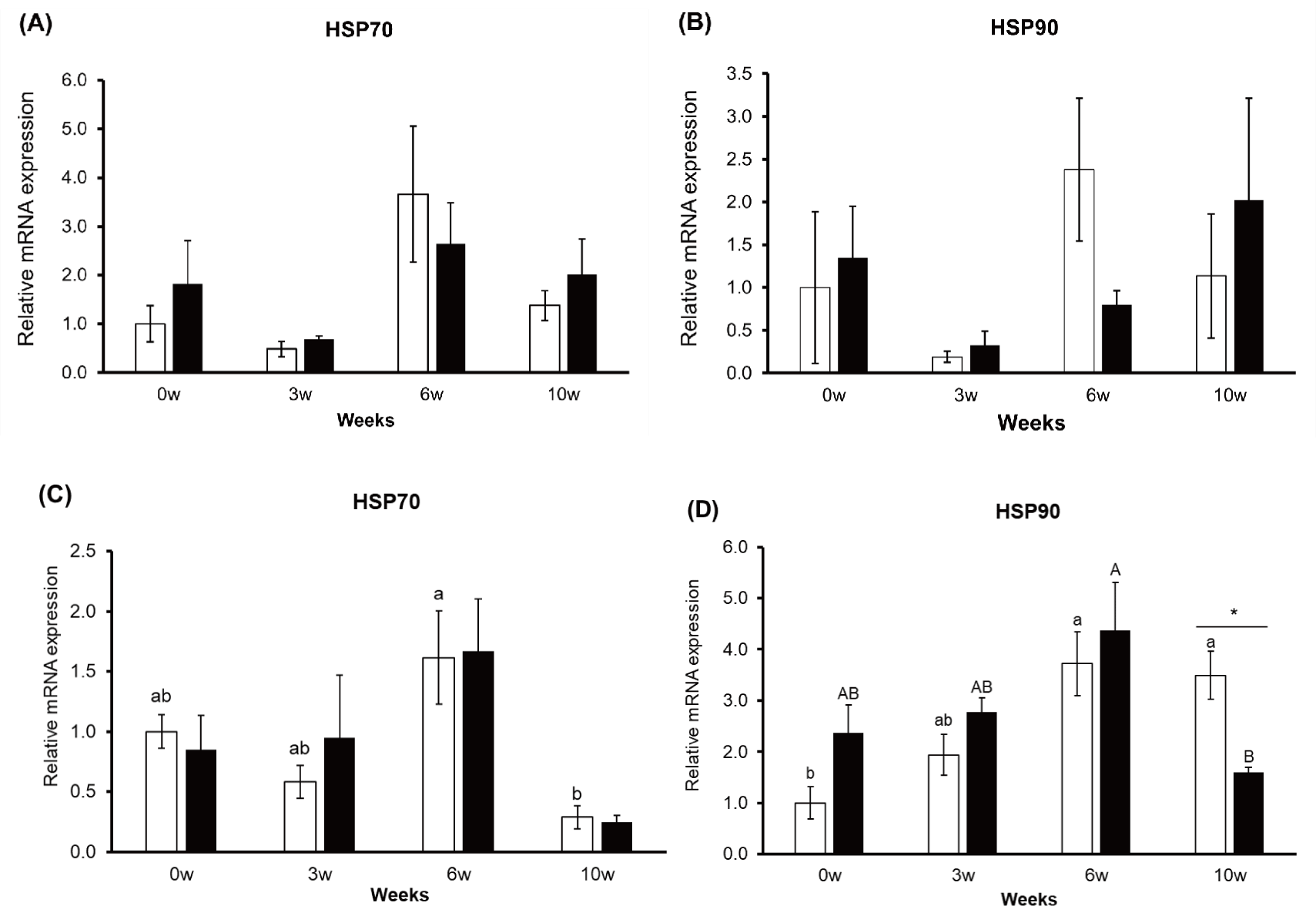
HSP70 and HSP90 gene expression were changed according to sampling weeks except HSP70 in the treatment group (p < 0.05, Figs. 3C and 3D). HSP90 gene expression in the hair follicle was decreased in the treatment group at 10 weeks (p < 0.01). The expression of HSP70 and HSP90 in the hair follicle is among the indicators of HS responses [22]. In the treatment group (0.5% Gln), the expression of these two HSPs was less than in the control group, meaning that Gln had a positive effect on the reduction in HS in 10 weeks. Thus, supplementation with Gln at 0.5% may attenuate HS at 10 weeks.
There were no differences between the groups in gene expression related to muscle tissue, including HSPB1, MyoD, MYF5, MYF6, and MyoG, and gene expression related to fat tissue, including FABP4, GDP, LPL, PPARγ, and SCD (Fig. 4, p > 0.05). Gln is known to be related to the development of muscle cells, such as the role of respiratory fuel in muscle cells, the synthesis of proteins of myosin heavy chain, and precursors for nucleotide [13]. Moreover, Gln reduced the HS in cells by increasing the gene expression of HSPB1 in bovine embryonic fibroblast cell [11]. As Gln was added by 0, 1, 2, and 4 mM, the expression of HSPB1 was linearly increased [11]. HSPB1-increased gene expression related to the differentiation of myocytes including MyoG and Desmin, and the increased cellular protein of myocytes [11]. Therefore, it could be expected that the expression of muscle development-related genes would sufficiently increase by the above functions of Gln under HS; however, there was no difference between the two groups in the current study (p > 0.05). The reasons could be speculated as follows. First, the supplementation of Gln was used in immune cells rather than muscle cells. The increase in immune cells, which is an essential factor for survival, is more important than muscle development. Second, as the amount of Gln stored varies depending on the muscle type, the reaction may differ depending on the Gln supplementation. Type I muscle fibers have approximately three times more Gln storage that type II fibers [12]. Type I muscle fibers have a higher activity of Gln synthetase and higher availability of ATP for Gln synthesis [12]. In the case of longissimus dorsi muscle sampled in this study, the number of type I muscle fiber was approximately 33.1%, and the area of type I muscle fiber was approximately 24.3% of muscle fiber in Hanwoo steers [42]. The amount of Gln in the longissimus dorsi muscle was higher than for round and chuck [43]. Thus, additional Gln treatment may be less effective because the longissimus dorsi muscle already stores a lot of Gln. Furthermore, we need to confirm the effect of Gln supplementation on muscle development according to the parts of muscle or the type of muscle fiber in cows.
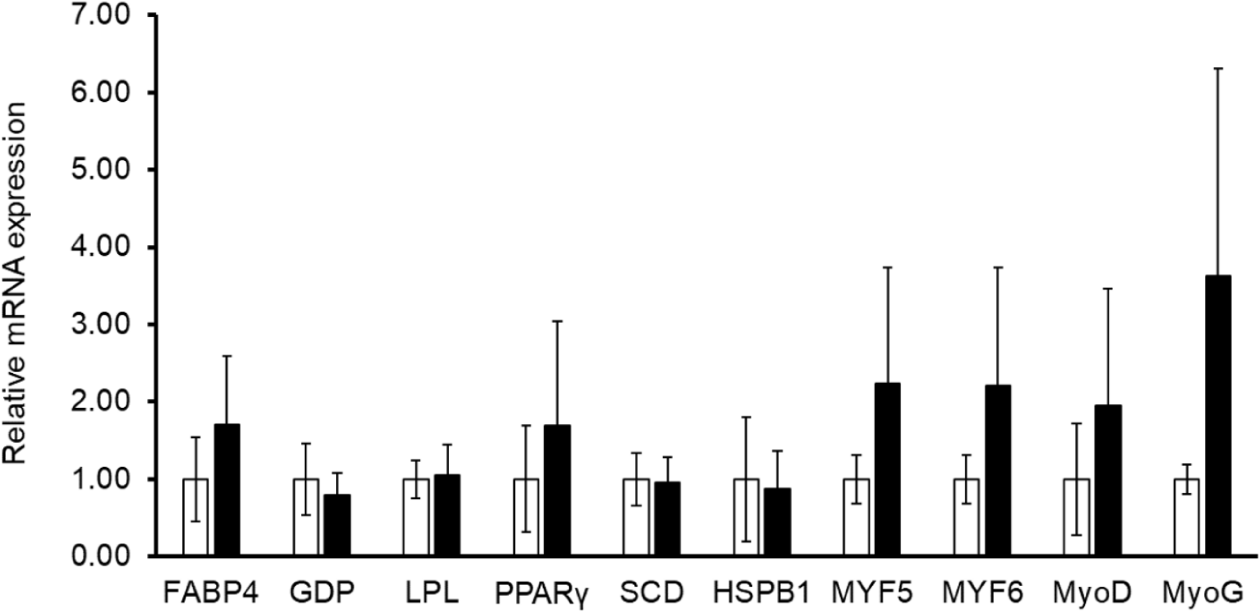
It was important to analyze the gene expression related to adipose developments in the longissimus dorsi muscle because this part of the muscle has interaction between muscle tissue, intra-, and intermuscular fat tissue. Carbone sourced from Gln was synthesized to fatty acid in adipocyte. Gln stimulated the gene expression of fatty acid synthase and GPD [13]. However, there was no difference between the control and supplementation groups for FABP4, GDP, LPL, PPARγ, SCD, HSPB1, MyoD, MYF5, MyF6, and MyoG in this study.
CONCLUSION
Short-term supplementation with Gln at 0.5% of the total diet (as-fed basis) stimulated lymphocyte and granulocyte proliferation despite the HS that caused immune system decline. Despite no differences in physiological and behavioral characteristics between the control and Gln supplementation group, our results indicated that HSP90 was more resistant to HS in the Gln supplementation group. Gln may prioritize immune improvement under HS condition as shown in this study by boosting immune parameters, including granulocyte, lymphocyte, monocyte, and WBC. Thus, supplementation with 0.5% Gln could be beneficial in improving the immune system in beef cattle under HS conditions. Further study is needed to examine whether muscle development improves when higher concentrations of Gln are added for longer periods.