INTRODUCTION
Spermatogenesis is a fundamental process in maintaining male fertility through the differentiation and self-renewal of male germ cells. Any abnormality in this process can result in subfertility/infertility. In addition to abnormal spermatogenesis, other factors, such as ill-health, disturbance in mating ability, reproductive problems, and behavioral disorders, also negatively affect stallion fertility. In the horse industry, unlike other domestic species, one primary reason for a lower fertility index than the considered standard in a stallion is that selection for breeding purposes is mainly based on performance records and conformation rather than reproductive soundness [1]. These maladies/practices demand more comprehensive research to address fertility in stallions to maximize profitability in the horse industry.
Somatic cells play a pivotal role in spermatogenesis. Different types of somatic cells are present in the lumen (Sertoli cells) and outer membrane (peritubular myoid [PM] cells) of the seminiferous tubules. Leydig cells are present within the connective tissue of the testicular stroma between the seminiferous tubules. In addition to the Leydig cells, nerve fibers, lymphatic vessels, the microvasculature, lymphocytes, fibroblasts, and macrophages surround the tubules [2]. These somatic cells, particularly Leydig and Sertoli cells, are season- and age-dependent in stallions. The number of Sertoli and Leydig cells is almost double in the breeding season compared with that in the non-breeding season [3]. Johnson and Neaves revealed age-related differences between 2- and 20-year-old stallions in the Leydig cell population. They found a five- and three-fold increase in Leydig cell volume and number/testes and a two- and three-fold increase in Leydig cell number and volume/g of parenchyma, respectively [4]. In another study of stallions aged from 6 months to 20 years, the highest number of Sertoli cell populations was recorded up to 4–5 years [5]. The endocrine system composed of complex network of organs and glands, uses the hormones to coordinate and regulate body metabolism, development, and reproduction. Paracrine pathway is cellular communication produce by cell to induce changes in nearby cells while autocrine pathway is a cell signaling originated from one cell and act on the same cell type [6]. Important paracrine-autocrine factors associated with spermatogenesis are included testosterone, estrogen, inhibin, activin, oxytocin, insulin-like peptide 3 (INSL3), proopiomelanocortin (POMC), transferrin, growth factors like transforming growth factor (TGF) alpha and beta, insulin-like growth factor-1 (IGFs). These factors are secreted from different types of somatic cells including Leydig, Sertoli, PM cells. The precise regulation of these somatic cells is required for a normal spermatogenesis. In this review, based on the emerging role of somatic cells in spermatogenesis, we summarized the biological function and development of Leydig, Sertoli, and PM cells and their roles in the regulation of spermatogenesis.
FUNCTIONS OF LEYDIG CELLS IN SPERMATOGENESIS
Leydig cells, which are important somatic cells, reside in the interstitial spaces between seminiferous tubules. The proliferation of Leydig cell precursors (stem Leydig cells), differentiation into immature Leydig cells, and final differentiation into adult Leydig cells are the three steps in the development of these somatic cells [7]. Leydig cells contribute to spermatogenesis in different ways. Leydig cells regulate spermatogenesis by affecting different growth factors. IGFs, inhibin, activin, and INSL3 are influenced by Leydig cells, thereby regulating spermatogenesis. IGF-1 plays a key role in spermatogenesis by differentiating spermatogonia [8] and regulating spermatogonial DNA synthesis [9]. Studies have also revealed the anti-apoptotic effects of IGF-1 on germ and Leydig cells in rats. In addition to that in experimental animals and humans, localization of IGF-1 has been reported in the testicular extract of stallions [10], Sertoli cells, Leydig cells of equine testicular cell culture [11], and stallion germ cells [12]. Hess and Roser found that IGF-1 levels are age- and season-dependent in stallions. They compared the IGF-1 levels in plasma and testicular extracts of stallions aged between 6 months and 23 years in breeding and non-breeding seasons. The highest level of IGF-1 was recorded in stallions less than 2 years of age (colt) compared with other age groups, and the seasonal difference was also recorded (highest level found in the breeding season) in colts. Significant differences in colts and fertility levels and no difference in other groups suggested that IFG-1 is involved in testicular development in stallions [10]. Another study conducted on the presence of IGF-1 and its receptor in stallion testicular tissue, and compared the expression patterns among different age groups found that regardless of the stage-dependent expression in germ cells, IGF-1 expression was found in the cytoplasm of Leydig cells at all stages, including pre-pubertal, pubertal, post-pubertal, and adult stallions. However, strong immunolabeling of IGF-1 and its receptor was found around puberty, suggesting that IGF-1 is involved in the proliferation of Leydig and germ cells via a paracrine/autocrine system [12]. Moreover, IGF-1 has been reported in the Leydig cells of dogs [13] and more transcription has been found in Leydig cells than in germ cells [14]. However, the presence of IGF-1 in somatic and germ cells indicates its significant role in spermatogenesis through proliferation and differentiation.
Inhibins and activins are glycoproteins and members of the TGF family. Inhibin is composed of a common α subunit primed with either a βA subunit (inhibin-A) or a βB (inhibin-B) subunit [15] considered to participate in regulating spermatogenesis. The inhibin-A isoform is detected in rams [16] and bulls [17] whereas the inhibin-B isoform is detected mainly in hamsters [18], miniature pigs [19], rats [20], and humans [15]. The localization of inhibin in Leydig [21] and Sertoli cells [22] has been reported in stallions. Reduced serum inhibin-B concentration seizes spermatogenesis [23] and is therefore used as a male fertility marker in many species [24]. In humans, it is used as a reliable marker of male fecundity [25]. Immunoreactive (ir-) inhibin in stallions increases together with estradiol, gonadotropin, and testosterone concentrations in the breeding season [21], and a decline in ir-inhibin concentration has been reported in infertile stallions [26]. In another study, ir-inhibin circulating concentration was 8.6-fold higher in stallions during the breeding season than in mares during the entire estrous cycle. Moreover, an 8.5-fold higher concentration was recorded in the testicular vein than in the jugular vein of stallions, whereas gonadectomized mares and stallions had no detectable ir-inhibin [27]. The presence of α and β subunits of inhibin in Leydig and Sertoli cells of stallions showed its possible role in regulating and maintaining spermatogenesis by secreting dimetric (bioactive) inhibin in the circulation of stallions. Furthermore, inhibin plasma concentration is season-dependent and thus can be used as a reliable indicator of testicular function in stallions during the breeding or non-breeding seasons [21]. Activity and production of activin A are necessary for the regulation of the seminiferous epithelium cycle during spermatogenesis [28] and stimulate DNA synthesis in germ cells (intermediate spermatogonia and preleptotene spermatocytes) in a dose-dependent manner [29]. Inhibin-A decreases DNA synthesis, whereas activin A increases the DNA synthesis thus participating in spermatogenesis by modulating DNA synthesis [29]. Activin is involved in the stimulation of follistatin and inhibin from Sertoli cells [30] and suppress steroidogenesis in Leydig cells of rats [31] through the paracrine/autocrine pathway. Studies have revealed the presence of activin in the follicular fluid [32] and placenta [33] of mares, but this has not yet been identified in stallion testes. Although its action in stallions is undefined, its presence in mares suggests that like in other species, it is assumed to regulate follicle-stimulating hormone release at the pituitary level [34]. Therefore, further studies are warranted in equines, especially stallions, to determine their presence and possible role in regulating spermatogenesis in stallions.
INSL3, previously called relaxin-like factor (RLF), secreted solely from Leydig cells, has been identified in several species, including stallions [35]. It has been used to recognize unambiguous Leydig cells in interstitial tissues [36] and differences in seasonal testicular functions [37] thereby acting as a typical marker for Leydig cell differentiation [38]. Recently, the expression pattern of INSL3 in Leydig cells and serum concentration levels have been reported in post-pubertal intact and cryptorchid stallions [35]. Serum INSL3 concentrations were recorded as higher to lower in intact, non-castrated unilateral, hemi-castrated unilateral, and bilateral cryptorchid stallions, respectively, and the expression level of INSL3 was positively correlated with the serum concentration [35]. A similar study by Klonisch et al. [38] revealed an upregulation and significantly strong expression of INSL3 in normally descended testes compared with that in the unilateral cryptorchid testes of stallions [39]. These results indicate a possible role of INSL3 in steroidogenesis and spermatogenesis in stallions.
Leydig cells are the main source of androgens [40]. The presence of androgen receptors (AR) in different reproductive parts of stallions, including the epididymis [41], testes [42], and prostate glands [43], and immunolabeling in all somatic cells, including Leydig cells [42], are important in spermatogenesis. Androgens are not only essential for male sexual differentiation and behavior [44] but also play an important role in maintaining spermatogenesis [45]. The possible mechanism is that androgen diffuses into the blood vessels of interstitial spaces and seminiferous tubules and binds to the AR present on Sertoli [46] and PM cells [47] thereby controlling male germ cell signaling and contributing to spermatogenesis [48]. Testosterone is the most common androgen involved in spermatogenesis maintenance. The intratesticular concentration is 10-fold higher than the serum concentration in rats, demonstrating the importance of testosterone in regulating spermatogenesis. The higher intratesticular concentration of testosterone is possibly because of its involvement in the progression of round spermatids, thereby controlling meiosis [49]. Previous studies revealed that testosterone withdrawal results in the failure of round spermatids to complete spermiogenesis [50] and high apoptosis of pachytene spermatocytes and round spermatids [51]. This theory is further strengthened by Stanton et al. [51], who found that deficiency or loss of testosterone influences changes in the expression of proteins involved in DNA repair, cell signaling, metabolism, and apoptosis [52] by facilitating meiotic progression without affecting spermatogonial proliferation [53]. 24-glutathione S-transferase A3 (GSTA3), dehydrocholesterol reductase (DHCR24), and squalene epoxidase (SQLE) are mainly expressed in Leydig cells. In humans and livestock, Δ4-androstenedione is the immediate precursor of testosterone produced by the 3-ketosteroid isomerase activity of the GSTA3 protein. Administration of steroids (dexamethasone) in stallions lowered the biosynthesis of testosterone, resulting in a 60% decrease in the main testosterone concentration [54]. Transcriptome analysis in this study showed that the expression of genes involved in steroidogenesis (GSTA3 and aromatase), cholesterol synthesis (DHCR24 and SQLE), and hormone signaling (luteinizing hormone receptor, α-actinin 4, glucocorticoid receptor α) were sharply downregulated by dexamethasone and hence decreased testosterone levels because of the interference of the Δ5-pathway of steroidogenesis in stallions [54]. In addition to controlling meiosis, testosterone plays an important role in regulating spermatogenesis by maintaining the blood-testes barrier [55], the release of mature sperm [56], and affects the expression level of connexin between round spermatids and Sertoli cells [57]. Oxytocin is an important autocrine, paracrine, and endocrine factor that is primarily involved in steroidogenesis and spermiation. Recently, its expression in different spermatogonia, spermatids, and Leydig cells of stallions has been studied [58]. The presence of oxytocin receptors in Leydig cells suggests that they are involved in steroidogenesis. Dose-dependent treatment with oxytocin results in increased basal testosterone production [59] and 5α-reductase activity in rat Leydig cells [60]. In vitro studies in mice and goats treated with oxytocin showed increased basal testosterone [61] and a 3.5-fold higher dihydrotestosterone concentration [62] respectively. It is suggested that the conversion of testosterone into dihydrotestosterone through 5α-reductase synthesis is regulated by the oxytocin level [63]. This hypothesis is further strengthened by the difference in oxytocin expression in the breeding and non-breeding seasons. The level of oxytocin in stallions was recorded to be higher during the breeding season [58]. However, the role of oxytocin and its exact mechanism in the Leydig cells of stallions and its involvement in stallion steroidogenesis require further studies. Aromatase P450 (aromatase), present in Sertoli and Leydig cells, is responsible for the modulation of steroidogenesis and spermatogenesis by converting testosterone to 17β-estradiol. Thus, estrogen is important for spermatogenesis and plays a key role in the development and maturation of reproductive tissues in males including stallions [64]. Leydig cell functions, controlled by follicle stimulating hormone (FSH) receptors located in Sertoli cells [65], and luteinizing hormone (LH) receptors present in Leydig cells are responsible for the production of testosterone and estrogen [64]. The expression of aromatase in Leydig cells [66], epididymis [67], Sertoli cells [66], seminiferous tubules [68], and prostate gland [67] in stallions was studied. The presence of aromatase in Sertoli cells indicates that, in stallions, estrogen production is not only controlled by Leydig cells but also by Sertoli cells via the paracrine pathway [66]. Studies have shown that immunolabeling and expression levels of aromatase are age-dependent in stallions. Hess and Roser found that the expression of aromatase in Leydig cells was more distinct as the age of stallions advanced, while immunolabeling in seminiferous tubules decreased, and no immunostaining was found in postpubertal stallions [68]. Aromatase expression in 2-year-old stallions was observed in two different types of Leydig cells, that is, Leydig cells with good reactivity (more distinct expression) and a weak reactivity (light expression) and no seasonal difference (from February and April to June) were found [66]. More studies are warranted to determine the role, estrogen plays in the stallion.
FUNCTIONS OF SERTOLI CELLS IN SPERMATOGENESIS
Sertoli cells provide structural, nutritional, and immunological support to spermatogonial stem cells (SSCs) and are often named the “nurse” or “mother” cells for SSCs. These are important parts of the testicular niche that play key roles in the development of SSCs and the formation of functional testes [69]. Sertoli cells in the seminiferous tubules can be recognized by their biochemical phenotypes and morphology. There are several distinctive features of Sertoli cells regarding the phenotype, such as FSHR, GATA4, Wilms’ tumor suppressor gene1 (WT1), SRY-box transcription factor 9 (SoX9), and vimentin. Morphologically, Sertoli cells have a smooth endoplasmic reticulum, abundant lipid droplets, well-developed nucleoli, intercellular junction complexes, mitochondria, and irregularly shaped nuclei [70]. SSC destiny is highly dependent and regulated by Sertoli cells in various manners.
Sertoli cells are involved in the self-renewal and differentiation of SSCs [71]. Stallion spermatogonia are usually classified into eight subtypes: As, Apr, Aal, A1, A2, A3, B1, and B2 spermatogonia [72]. Symmetric or asymmetric division of SSCs occurs during the infancy of spermatogenesis. The dominance of differentiation or self-renewal of SSCs may result in Sertoli cell-only syndrome (SCOR) or tumors, respectively [73]. Therefore, understanding the regulation mechanism of the precise equilibrium between the differentiation and self-renewal of SSCs is highly important for diagnosing male infertility. As a “mother” and important part of the niche, Sertoli cells play a significant role in modulating differentiation and self-renewal of SSCs by secreting different cytokines and growth factors. Retinoid acid (RA), a molecule derived from vitamin A in Sertoli cells, plays an important role in SSC differentiation. Primordial germ cells failed to enter meiosis in the absence of RA within the seminiferous cords of fetal testes [74] and increased meiotic activity and upregulation of receptor tyrosine kinase (KIT) expression (a marker of the switch from undifferentiated to differentiating spermatogonia) were observed in vitro after treatment with RA [75]. It has also been observed that Vitamin A-deficient rats or -mice have only type A spermatogonia and Sertoli cells with spermatogenesis arrest [76]. The consequences of aging result in different deleterious alterations, including developmental and genetic defects, stochastic, environmental, and epigenetic events, as well as innate aging processes [77]. Lower Sertoli cell ratios (germ cells / Sertoli cells), germ cell number, and volume [78] along with a reduction in sperm quality and fertility [79] are consequences of testicular aging. Recently, it was revealed that the lack of retinoic acid receptor-related orphan receptor-alpha (ROR-α) increases testicular aging [80] and abnormal Sertoli cells, reduction in Leydig cell number, vacuolation of seminiferous tubule epithelium, hypo-spermatogenesis, and poorly developed sperm were noted in 3-month-old RORα-/- mice [81]. It is suggested that during different stages of seminiferous epithelial development, bone morphogenetic protein 4 (BMP4), an important member of the TGF-β family released from Sertoli cells and spermatogonia in adult testes, helps in the differentiation of SSCs through autocrine and paracrine pathways [82]. BMP4 receptors (BMPRIa and BMPRII) promote RA signaling in mouse SSCs in vitro, and Noggin, a BMP4 antagonist, prevents the RA-induced expression of KIT and Stra8 [83]. KIT, an important molecular marker, is a receptor for stem cell factor (SCF). KIT localization in differentiating SSCs in all stages of stallions, including pre-pubertal, pubertal, post-pubertal, and adult stages, indicates the role of this protein in spermatogenesis and makes it a potential candidate for the isolation and identification of differentiating germ cells from stallions [84]. KIT and SCF are believed to regulate the differentiation or proliferation of SSCs by activating the PI3K/Akt pathway via type A spermatogonial DNA synthesis [85]. In vitro spermiation is achieved by the induction of CSF from telomerase-immortalized mouse type A spermatogonia [86]. A reduced apoptosis by up to 21.80%, 37.45%, and 44.40% in a short-term stallion testicular culture treated with SCF combined with granulocyte–macrophage colony-stimulating factor (GM-CSF), leukemia-inhibiting factor (LIF) + GM-CSF, and estradiol (E2) + LIF + GM-CSF, respectively [87]. Reduction in apoptosis in this study demonstrating the key role of SCF in the differentiation of SSCs. The expression levels and localization of RA and BMP4 and their in vitro studies in stallion testes are needed to determine their actions and species-specific characteristics for a better understanding of spermatogenesis in stallions.
Sertoli cells are involved in SSC self-renewal. Among TGF-β family members, glial cell line-derived neurotrophic factor (GDNF) is a major growth factor produced by Sertoli cells. Previous studies have revealed a connection between GDNF and SSC self-renewal [88]. Aggregation of undifferentiated spermatogonia was observed in GDNF-overexpressing mice, in contrast to a reduced percentage of spermatogonial proliferation in an age-dependent manner in Gdnf+/− mice [89]. GDNF family receptor alpha-1 (GFRA1) expressed in undifferentiated spermatogonia type A of stallions [72] is considered to be important for SSC self-renewal in stallions, as in mice [90]. More than 90% prevalence of GFRA1 labeling of undifferentiated stallion spermatogonial cells suggests that almost all subtypes of undifferentiated spermatogonia (As, Apr, and Aal) express the GFRA1 receptor in stallions, but further studies such as whole-mount analysis are warranted to confirm this finding [72]. In another study, TGF-α and vascular endothelial growth factor (VEGF) and its receptor VEGF-R2 were investigated in a dose-dependent treatment of peripubertal stallions with Durateston [91]. The expression of TGF-α and VEGF-R2 in peripubertal stallions castrated after four weeks of treatment was significantly higher than that in the control group. Similarly, significantly higher expression of VEGF-R2 was observed in Sertoli cells of stallions castrated after 12 weeks of treatment than after four weeks of treatment and the control group [91]. These studies showed that TGF is more important than other factors, such as IGF, LIF, and fibroblast growth factor 2 (FGF2), for SSCs self-renewal [92]. Transferrin, a glycoprotein is largely secreted from Sertoli cells and involved in the meiotic progression and responsible for the import of iron to the seminiferous tubules necessary for spermatogenesis [93]. In a study, a decrease in transferrin receptors in mice results in decrease of pachytene spermatocytes and accumulation of zygotene and leptotene spermatocytes, denoting early meiotic arrest [94].
Sertoli cells act as phagocytic agents of SSCs. Death or expansion of germ cells is necessary to maintain the ratio of germ cell-Sertoli cells to regulate spermatogenesis [73]. Over 50% of differentiating spermatogonia undergo apoptosis, a programmed death of SSCs. However, an increase in apoptosis may lead to subfertility/infertility, resulting in considerable financial loss in the equine industry. Normally, the number of differentiated A1 spermatogonia decreases to approximately 25% because of A2, A3, and A4 spermatogonia apoptosis [95]. For normal and efficient regulation of spermatogenesis, dead spermatogonia and residual bodies are degraded and phagocytosed by Sertoli cells through a phospholipid phosphatidylserine (PS)-dependent pathway [96]. In addition to the involvement of Sertoli cells in differentiation, self-renewal, and apoptosis, they play a key role in the de- [97] and trans-differentiation [98] of spermatogonial germ cells. Furthermore, alterations in the number or maturation [99], damage to cell junctions [100], and alteration of hormone receptors on [101] of Sertoli cells result in impaired spermatogenesis.
FUNCTIONS OF PERITUBULAR MYOID CELLS IN SPERMATOGENESIS
PM cells, thin smooth-muscle-like cells disseminate over the peripheral surface of the basal lamina of seminiferous tubules. Compared with other somatic cells, relatively little is known about their role in spermatogenesis [102]. These are an important part of the testicular niche and are considered to be involved in tubule contraction, which helps in spermiation [103]. They also help to form the basal lamina of the tubules by facilitating the secretion of extracellular matrix materials such as type I and IV collagens, fibronectin, and proteoglycans [104]. Decreases in testicular size, number of germ cells, and sperm production have been noted in a conditional knockout of the Ar gene in PM cells [47]. Furthermore, PM cells have recently been shown to play an important role in postnatal testicular growth by affecting the proliferation of Sertoli cells [105]. Based on these findings, we will discuss the possible and prominent role of PM cells in the structural integrity of seminiferous tubules and their participation in regulating steroidogenesis, spermatogenesis, and testicular function. The interactions of factors from all somatic cells including Leydig, Sertoli and PM cells in controlling spermatogenesis are illustrated in Fig. 1.
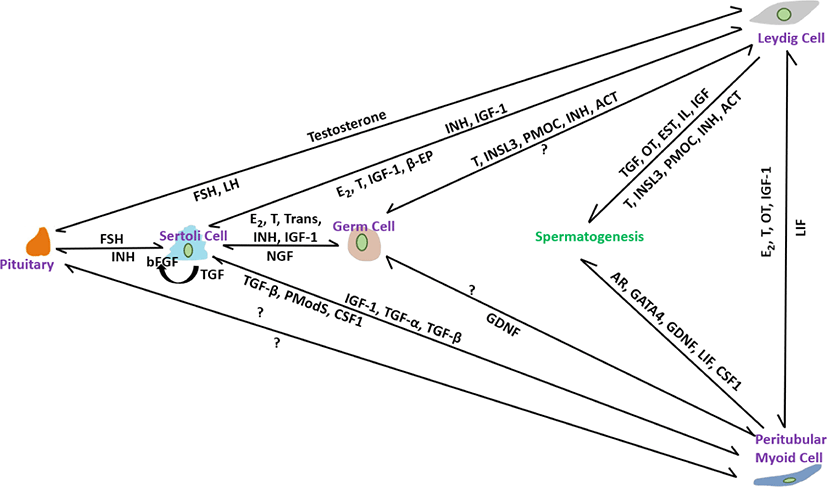
PM cells contribute to SSC proliferation. Previously, it was considered that GDNF produced by Sertoli cells secretes auxin-binding protein (ABP) and transferrin (TRF) and manipulates the differentiation of SSCs. However, testosterone treatment of PM cells has recently been shown to provoke GDNF secretion and regulate SSC development [106]. Reduced sperm count in the cauda epididymis [106] and a significantly low number of sperm and testicular volume [107] were noted in Gdnf-defective and SM cell AR knockout (PTM-ARKO) mice, respectively. Androgen is necessary for the manipulation of spermatogenesis and its receptors found in all somatic cells, including PM cells, at all ages of stallions, including pre-, peri-, and post-pubertal [42]. No age-specific differences in AR immunolabeling were recorded in stallion testes, suggesting that steroidogenesis and spermatogenesis are initiated and regulated by androgen via somatic cells, including PM cells, by the paracrine/autocrine pathway system, but not via germ cells. It has been hypothesized that androgen deposition is maintained by the interaction of PM cells with Leydig Cells because aberrant spermatogenesis is prominent in abnormal PM cells [108]. Reduction in the Steroidogenic factor (SF)-1 gene, which is essential for the differentiation of stem Leydig cells [109], and a decrease in the proportion of germ cell attachment to Sertoli cells [47] in PTM-ARKO mice indicated that PM cells play a role in the regulation of Leydig and Sertoli cells. In another study [42], presence of estrogen receptor-alpha (ESR-α) in PM cells in post-pubertal stallions but absent in pre-and peri-pubertal stallions suggesting that perhaps, in post-pubertal, the paracrine factors like peritubular myoid substances (Pmods) produced by PM cells regulate the functions of Sertoli cells [110]. Furthermore, ESR- β was not identified in PM cells of all age groups; therefore, it is hypothesized that in pre-and peri-pubertal stallions, estrogen actions are carried out in Leydig and Sertoli cells through ESR- β [42]. LIF produced mainly from PM cells regulate the proliferation and survival of SSCs [111]. The structural integrity of seminiferous tubules and the formation of the blood-testes-barrier are regulated by the paracrine effect of LIF [112]. Stallion testicular cultures treated for 6 h with GM-CSF, LIF, SCF, and E2 reduced apoptosis by 44.40% [87]. PM cells participate in SSC self-renewal. CSF1 found in PM cells, in combination with other growth factors, such as GDNF and FGF2, promotes SSC self-renewal. Oatley et al. [110] found 2.1-and 3.2-fold higher SSC numbers in CSF- treated testicular cell culture at 35 and 63 days, respectively [113]. Intermediate filaments composed of vimentin are used to calibrate the functional state of individual cells found in all somatic cells, including PM cells [93]. In stallion testicular tissue, strong immunoexpression was observed in somatic cells, including PM cells, in normal seminiferous tubules, whereas weak immunoexpression was observed in abnormal seminiferous tubules with low or no spermatogenesis [114]. Vimentin filaments are believed to be involved in the maturation of spermatogenesis via gap junction intercellular communication and maintenance of cell integrity therefore, their roles in stallion testes require further study for a better understanding of the regulation of spermatogenesis.
CONCLUSION AND FUTURE DIRECTION
Fertility is maintained by overlapping functions of different cell types. Although these functions are responsible for regulating spermatogenesis by different correcting or compensating factors when environmental or genetic factors impact the normal testicular structure. This overlap makes it difficult for researchers to understand the individual roles of different somatic cells in the self-renewal, proliferation, and apoptosis of SSCs. In vivo and in vitro cell/organ culture systems in laboratory animals are helping researchers understand the mechanisms of spermatogenesis; however, this type of research is limited to domestic animals, especially stallions. Culture studies will enable researchers to highlight and help diagnose the important cascade and cell type-specific factors involved in the fate of SSCs and provide a more conclusive understanding of the role of an individual cell or the contribution of distinct signaling molecules during spermatogenesis in domestic animals.
Most studies have been conducted within the seminiferous tubule (Sertoli cells-germ cells interaction) or interstitial (role of Leydig cells) compartments, compared with studies conducted to understand the influence and interaction of different somatic cells across the two compartments. More focus on the seminiferous tubule compartment and assumption of the “nurse-like” quantities of Sertoli cells results in more research focus on Sertoli cell-germ cell interaction pathways and less on other somatic cells, particularly PM cells. However, with the advancement of cell/tissue culture studies in laboratory animals, it is evident that the SSC niche is regulated by signals received from cells residing in interstitial and peritubular compartments. In addition, recent studies have revealed that the paracrine, autocrine, and endocrine signals provided by all testicular cells, including macrophages and somatic cells, influence germ cells directly or indirectly through Sertoli cells, thereby influencing spermatogenesis. Therefore, a comprehensive study of somatic cells, particularly PM cells, is required to understand spermatogenesis.
Several different growth factors, hormones, receptors, and cytokines procured from all three somatic cells, including Sertoli, PM, and Leydig cells, involved in the regulation and manipulation of spermatogenesis are illustrated in Table 1; however, the mechanisms by which these signals interact within somatic and germ cells are largely unknown in domestic animals, especially stallions. Therefore, thorough studies are warranted to understand these factors in-order to establish treatment regimens for infertility/subfertility in domestic animals including stallions. It is critical to maintain the fertility of stallion, therefore it is necessary to consider the reproductive soundness during selection of stallions for breeding purpose than the performance records and conformation. Moreover, establishment of a comprehensive protocol for stallion cell culture studies to explore different paracrine/autocrine factors produced from somatic cells, and treatment of somatic and germ cells (whole cell study) with different growth factors are necessary to improve the fertility in stallion.
Factor/receptor | Identified in stallion | Localization within testes | Effects | Reference |
---|---|---|---|---|
IGF-1 | +++ | Leydig cells | Proliferation of Leydig cells and germ cells | [12] |
Inhibin | +++ | Leydig cells, Sertoli cells | Suppression of FSH for maintaining spermatogenesis | [21,22] |
Activin | --- | Germ cells, Leydig cells, Sertoli cells | Modulate the release of FSH from the pituitary | [29] |
INSL3 | +++ | Leydig cells | Development of the gubernaculum testis | [39] |
AR | +++ | Germ cells, Leydig cells, Sertoli cells, PM cells | Sexual differentiation and behavior. Regulate steroidogenesis and spermatogenesis | [43,44] |
GSTA3 | +++ | Leydig cells | Testosterone production via 3-ketosteroid isomerase activity | [54] |
DHCR24 | +++ | Leydig cells | Cholesterol synthesis and regulate steroidogenesis | [54] |
SQLE | +++ | Leydig cells | Regulate steroidogenesis | [54] |
Oxytocin | +++ | Germ cells, Leydig cells | Regulate 5α-reductase synthesis activity | [58] |
Aromatase P450 | +++ | Leydig cells, Sertoli cells | Convert testosterone into 17β-estradiol | [64] |
LH receptors | +++ | Leydig cells | Production of testosterone and estrogen | [66] |
ESR-α | +++ | Germ cells, Leydig cells, Sertoli cells, PM cells | Regulate steroidogenesis | [42] |
ESR- β | +++ | Germ cells, Leydig cells, Sertoli cells | Regulate steroidogenesis | [42] |
KIT | +++ | Germ cells | Differentiation or proliferation of SSCs by PI3K/Akt pathways | [84] |
BMP4 | --- | Germ cells, Sertoli cells | differentiation of SSCs | [82] |
VEGF | +++ | Germ cells, Leydig cells, Sertoli cells | Proliferation of endothelial cells | [91] |
LIF | --- | PM cells | Regulate proliferation and survival of SSCs | [111] |
GDNF | --- | Sertoli cells, PM cells | self-renewal of SSCs | [88] |
GFRA1 | +++ | Germ cells | SSCs self-renewal | [90] |
Transferrin | --- | Sertoli cells | Iron supplementation to seminiferous tubules | [93] |
FSHR | +++ | Germ cells, Sertoli cells | Regulate steroidogenesis | [65] |
IGF-1, insulin-like growth factor-1; FSH, follicle stimulating hormone; INSL3, insulin like peptide 3; AR, androgen receptor; PM, peritubular myoid; GSTA3, glutathione S-transferase A3; DHCR24, dehydrocholesterol reductase; SQLE, squalene epoxidase; LH, luteinizing hormone; ESR, estrogen receptor; KIT, receptor tyrosine kinase; SSCs, spermatogonial stem cells; PI3K, phosphoinositide 3-kinases; Akt, Ak strain transforming; BMP4, bone morphogenetic protein 4; VEGF, vascular endothelial growth factor; LIF, leukemia inhibitory factor; GDNF, glial cell line-derived neurotrophic factor; GFRA1, GDNF family receptor alpha-1; FSHR, follicle stimulating hormone receptor.