INTRODUCTION
Mold spores are spread ubiquitously in the environment [1]. A recent report indicates that mycotoxins are detected in 60% to 80% of agricultural products [2]. Mycotoxins are not only found in cereals (wheat, maize, rice, barley, and sorghum) as the most commonly contaminated products but are also found in animal products (meat, eggs, and milk) [2,3]. Conidia of the fungal genera, Aspergillus, Penicillium, and Cladosporium spp., are also predominant in the air and grow in food- or feedstuff storage areas and processing facilities [1,3]. The distribution of mold is unavoidable in slaughterhouse environments because considerable amounts of water are used for carcass washing and rinsing, creating a relatively humid and nutrient-rich environment (due to the residues of nutrient-rich meat). For these reasons, a common step in cleaning facilities or machines in meat/food processing or manufacturing plants is the use of chemicals. However, many fungal strains are developing resistance to the most widely used fungicides, and consumers are unaware of which residues (chemicals, antibiotics) are eventually being transferred to the food [4]. The microbial load on pork carcasses strongly depends on the spread of microorganisms during the slaughtering process (i.e., stunning, exsanguination, scalding (SC), dehairing (DH), singeing, evisceration, splitting, and cooling) [5]. In slaughterhouses (wall, floor, bleeding, and evisceration room), various microorganisms have been associated with biological risks from the direct or indirect contact with animal matter (fecal, intestine) and the surface of the pig’s skin [5,6]. Appropriate hygiene control must be ensured during the production stages to prevent molds from eventually being transferred to the carcass.
In the slaughterhouse, most equipment (e.g., bucket, knives) is made of stainless steel (SS) [7,8]. Failure to sterilize knives and equipment regularly can result in microbial cross-contamination of the carcass [8,9]. Common practices to maintain hygienic conditions after completion of each process include soaking or washing small implements in hot water and sterilization in a ultraviolet (UV) light device before the next slaughter. The mechanism of UV-C light (200–280 nm) involves primarily the formation of pyrimidine dimers, which inhibit microbial gene transcription and replication, which eventually leads to cell death [10,11]. Although an effect of UV-C irradiation and thermal treatment on bacteria has been reported previously [12], comprehensive scientific evidence for its application in slaughterhouses for reducing mold formation and proliferation is required.
The aim of this study was to investigate the prevalence of mold in pig slaughterhouses in Korea and confirm the appropriate method of combined treatments with UV-C and hot water that can reduce the abundance of four commonly encountered fungi species (Aspergillus niger [A. niger], Penicillium commune [P. commune], Penicillium oxalicum [P. oxalicum], and Cladosporium cladosporioides [C. cladosporioides]) isolated from pig slaughter facilities.
MATERIALS AND METHODS
Sampling was conducted by swabbing 20 facilities and tool surfaces (i.e., nipple drinkers [ND], floor of lairage area [L], walls of lairage area, scale [S], stunning gun [SG], rack after stunning [RS], shackle [SH], container for exsanguination [CB], first cleaning, scald, DH, rack after dehairing [RD], second cleaning [SEC], saw [SAW], intestine tray, intestine table, final cleaning [FC], conveyer belt [CV], hook [H], and wall of chilling room [WC]). We treated the swabs (10 × 10 cm2) with 1 mL saline (3M Microbiology Products, St. Paul, MN, USA). The method was carried out according to the solid surface sampling method proposed by the MFDS [13]. Serial dilutions of the sample suspensions were prepared in 0.1% peptone water and plated on potato dextrose agar (PDA) (Difco, Sparks, MD, USA).
For identification, each separate fungus was grown on PDA at 25°C for 7 d. A single colony was streaked and incubated on PDA. After incubation, a small amount (10 µl) of fungal mycelia was captured with a loop and suspended in 900 µL of UltraPure Distilled Water (Invitrogen, NY, USA). The suspension was boiled at 100°C for 2 min and stored at −70°C after cooling. Samples were sent to SolGent (Daejeon, Korea) to complete DNA sequencing. Internal transcribed spacer (ITS) sequences of nuclear ribosomal DNA were amplified with two universal primers: ITS1 (5'-GTA GGT GAA CCT GCG G-3'), and ITS4 (5'-TCC GCT TAT TGA TAT GC-3'). Polymerase chain reaction (PCR) was conducted with the following conditions: initial denaturation at 95°C for 15 min, 30 cycles of denaturation at 95°C for 20 s, annealing at 50°C for 40 s, and extension at 72°C for 1.5 min, with a final extension for 5 min at the same temperature. The purified PCR products were sequenced by an ABI PRISM 3730LX DNA Analyzer (Applied Biosystems, CA, USA). The sequences were assembled to obtain the full-length sequence, which was identified using the National Center for Biotechnology Information (NCBI) GenBank database (http://www.ncbi.nlm.nih.gov.).
SS (Grade 304 with 2B finish, 10 × 10 cm) chips were prepared. Prior to using the chips, the SS surface was cleaned with neutral detergent, wiped with 70% ethanol, and dried. The SS chips were sterilized by autoclaving for 20 min at 121°C and then stored at 25°C [14]. The fungal strains (A. niger, P. commune, P. oxalicum, and C. cladosporioides) were incubated for 3–5 d at 25°C. The spore suspensions were set to a final concentration of 106-7 spores/mL. Before the treatment, 1 mL of the suspension was spotted on the SS chips and dried for 3 h at 37°C.
In the hot water (60°C, 65°C, 70°C, and 83°C) treatments, the SS chips inoculated with each mold were immersed in hot water for 0.5, 1, 3, 5, and 10 min. The UV-C irradiation experiments were conducted using a bench scale UV chamber, HD 11077-4002A (Donghwa Plant, Osan, Korea) with a 15 W low-pressure UV-C lamp (Sankyo Denki, Kanagawa, Japan) that emitted monochromatic UV-C irradiation at 260 nm. The dosage of UV light exposure (mJ/cm2) was confirmed using a UV-C light meter, UVC-254A (Lutron, Taiwan). Individual UV-C doses of 15, 30, 90, 150, 300, and 600 mJ/cm2 (0.5, 1, 3, 5, 10, and 20 min) were applied to the surface of the SS chips (10 × 10 cm). To ensure a constant UV intensity output, the UV lamp was kept on for at least 30 min before each experiment, and the distance between the sample and lamp was maintained at 30 cm. For the combined treatment, hot water (60°C) treatment was performed immediately after UV-C irradiation (15, 30, 90, 150, and 300 mJ/cm2). Each step was conducted for the same time as that used for the single treatment described above. The resulting suspensions were serially diluted in diluent saline (3M) and plated on PDA for molds. Samples were incubated at 25°C for 3–5 d.
The antimicrobial effects of the single (UV-C/hot water) and combined treatments (UV × hot water) were examined in triplicate, and treatment differences were evaluated by analysis of variance using the SAS v.9.4 software (SAS Institute, Cary, NC). Values of p < 0.05 were considered significant, and data were grouped using Duncan’s multiple range test.
RESULTS AND DISCUSSION
Fungi and mycotoxin are common contaminants of meat products [2,3,15]. These microorganisms form spores, which facilitate their proliferation in food and the environment. However, their control is limited in enclosed spaces, such as slaughterhouses and meat processing plants. They either cause spoilage or produce mycotoxins, transferring the contaminants to the consumer [16]. In the present study, the prevalence of mold in the five pig slaughterhouses in Korea was investigated. A total of 179 different mold isolates of 46 different genera were identified. The most abundant genera (collectively more than 65% of the total mold diversity) were Cladosporium (20%), Penicillium (15%), Cystobasidium (7%), Aspergillus (6%), Rhodotorula (6%), Cutaneotrichosporon (5%), Trichosporon (4%), and Apiotrichum (3%) spp. (Fig. 1A). Sampling was performed at five pig slaughterhouses and samples were collected from the following 19 spots: ND, L, S, SG, RS, SH, CB, cleaning (C), scalding (SC), DH, RD, SEC, SAW, evisceration tray/table (ET, ETA), FC, CV, H, and WC (Fig. 1B). Cladosporium (16/19 spots) was the most abundant and widely distributed genus throughout the various sites, followed by Penicillium (14/19 spots) (Fig. 1A). Various types of mold (more than ten genera) were detected in the contaminated areas (ND, L, S, and RS), cleaning systems (C and SEC), and tools (H) that were in contact with the pig intestines. In the contaminated areas, various fungi appeared due to feces on the surface of the pigs’ skin. In the case of ET and CB, a diversity of fungi was seen due to cross contamination of fungi from the intestines. In general, the most widely distributed genera around the facilities and tools in contact with carcasses during slaughter were Cladosporium and Penicillium. Another study found Aspergillus, Cladosporium, and Penicillium spp. as the predominant fungi in the slaughterhouse environment [17]. Strains that are frequently detected and of concern in the contamination of carcasses and meat products were selected as targets, which included A. niger, P. commune, P. oxalicum, and C. cladosporioides. These species, including Cladosporium and Penicillium, have been isolated from more than 70% of carcass or meat products from dry-cured fermented/smoked meat, and Aspergillus has been isolated from canned beef and poultry [16,18]. Cladosporium cladosporioides and P. commune can form black and green spots on the surface of fermented meat [18,19]. Cladosporium spp. was the most frequently isolated genus related to contamination in the slaughterhouse. A study of bacterial prevalence in pig slaughterhouses found the minimum count of bacteria to be 3.0 Log CFU/cm2 on the hook surface of the chilling room [20]. In the present prevalence study, various fungi, such as Cladosporium spp., Cystobasidium spp., and Penicillium spp., were also detected on the WC (Fig. 1A). Condensation occurred on the wall due to the temperature difference between the chilling room and the carcass. This humid environment is conducive to mold growth. Compared to the FC step, fungi, such as Penicillium spp., Cladosporium spp., and Cystobasidium spp., were also detected in the F and SEC steps (Fig. 1A). Although high water pressure (30–40 psi) hosing is suggested by theMAFRA [21] while cleaning facilities, moisture accumulates around the equipment over time, which is conducive to the formation of scattered invisible spores on the skin [21]. Microbiological contamination can also be caused by blood droplets, meat tissue, gut contents, and ineffective removal or movement of the products from one point to another within the slaughter area [20]. From the standpoint of operating a pig slaughterhouse, research on the management and control of Penicillium spp., which are highly prevalent in slaughterhouses, is necessary.
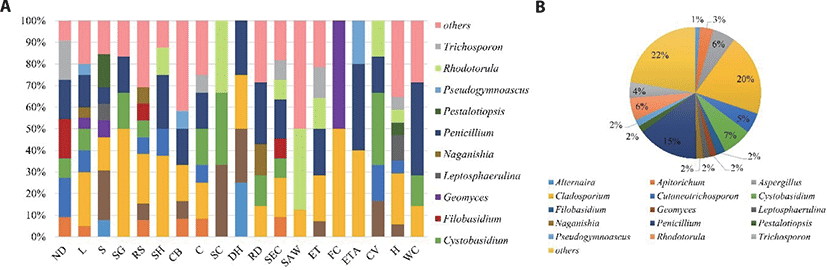
In this study, P. commune and P. oxalicum comprised 40.7% of Penicillium spp., A. niger comprised 30.0% of Aspergillus spp., and C. cladosporioides comprised 33.3% of Cladosporium spp. As these four species were detected at high proportions in the slaughterhouse, they were selected and used for further experiments on the reduction of fungal populations.
Consumer concerns about chemical residues being transferred to carcasses are increasing, and physical technologies (UV-C and hot water) have, therefore, been applied to remove fungi from SS materials. A reduction in the four fungi (p < 0.05) was observed during individual UV-C treatments for a maximum of 20 min (Fig. 2). Because most equipment in the slaughterhouse is made of SS, the inhibitory effect of individual physical treatments on the SS chips was tested in the laboratory. More than 20 min, heat was generated on the sur face of the SS chips, and the effect could not be excluded, so a single treatment was performed for up to 20 min. The initial concentration of the inoculum on the SS chip was 106-7 spores/mL. The average population of the control on the PDA was 7.6 Log CFU/cm2. The fungi were reduced as the UV-C dose increased (p < 0.05). The reduction effect was low after 5 min of UV-C irradiation (≤ 1.0 Log CFU/cm2), but increased in P. oxalicum and A. niger to 1.3 and 1.6 Log CFU/cm2, respectively, at 20 min. The maximum reduction with the UV-C treatment was approximately 2.0 Log CFU/cm2 at 600 mJ/cm2 and was the most effective on P. commune; in contrast, C. cladosporioides (0.8 Log CFU/cm2 reduction) appeared to be UV resistant. Since the maximum reduction of A. niger, P. commune, P. oxalicum, and C. cladosporioides was 1.6 (97.3%), 2.0 (98.9%), 1.4 (95.6%), and 0.8 (84.9%) Log CFU/cm2, respectively, the inhibitory effect of the UV-C sterilizer used in the slaughterhouse was insufficient for treating these four fungi. Similarly, A. niger (a UV resistant strain) was reduced by 1.0 Log CFU/cm2 with a UV-C dose of 150 mJ/cm2 (p < 0.05) and decreased by 0.85 Log CFU/cm2 when irradiated with 120 mJ/cm2 UV-C [22]. Penicillium commune on the SS chip was reduced by 2.0 Log CFU/cm2 at 600 mJ/cm2, which is the highest response to date. Another study reported a 2.3–4.4 Log CFU/cm2 reduction at 100 mJ/cm2 by inoculating Penicillium spp. on a membrane filter [23]. Membrane filters are thinner than SS chips, and the inoculated cells are susceptible to the UV dose; thus, a higher effect can be observed even at low irradiation doses. Studies describing the effect of UV on Penicillium spp. conidia are limited. Moreover, the available studies are difficult to compare with each other due to differences in the matrices [23].
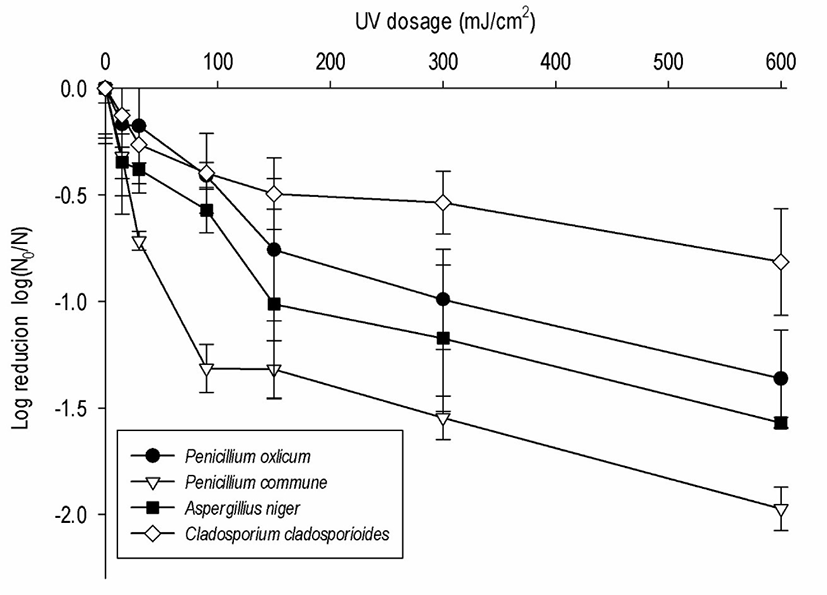
To clean tools and equipment in the slaughterhouse, workers dip and spray them in hot water. The maximum temperature of the water was set at 83°C according to theMAFRA [21]. Rebecca [24] demonstrated that immersion of knife blades in 70°C and 75°C hot water for 60 s was as effective as dipping them in water with a temperature of 80°C and 82°C; her results, however, revealed no significant reduction in Listeria monocytogenes on knives sterilized using the abovementioned methods at these temperatures [25]. Also, the study showed that water at 82°C could be substituted with water at 60°C when sterilizing knives in slaughterhouses, as water at both temperatures provided an equivalent microbiological outcome [25]. Moreover, the use of water at 60°C h would be more economical and safer for the workers. Therefore, four water temperatures ranging from 60 to 83°C were used in the present study, and the inhibitory effect of various temperatures and sterilization times on the fungal population was evaluated. To confirm the reduction in fungi by increasing the water temperature, the inhibitory effect was verified by inoculating the fungi onto the SS chips (the main material used in slaughterhouses), and immersing them in hot water (60°C, 65°C, 70°C, and 83°C). All the fungi decreased, depending on temperature and immersion time (p < 0.05) (Table 1). Though UV-C was not effective on C. cladosporioides, this fungus was the most sensitive to the thermal treatment. There was no residue after immersion for 1 min at 60°C. When A. niger was treated for more than 5 min at 65°C, its abundance was reduced below the detection limit. Additionally, another study indicated that conidia (Aspergillus, Penicillium, and Cladosporium spp.) have adequate heat resistance, with a D-value of 5 min at 55°C–64°C [1]. Usually, a hot water (65°C) spray for 30 s is sufficient to remove blood and meat tissue from the floor [20]. However, at both 70°C and 83°C, no fungi were detected when treated for more than 5 min; P. oxalicum appeared more resistant to heat treatment. As most fungi survived at 60°C, a combined treatment was conducted at a relatively low temperature (60°C) with UV-C (15, 30, 90, 150, 300, and 600 mJ/cm2) to confirm the proper treatment time and dose.
As mentioned above, hot water sanitation alone is insufficient for the elimination of some mildly heat-resistant fungi. To achieve the desired safety using only one technology necessitates a high severity that may have negative consequences for the nutrient content of the meat [26]. Research on mold reduction through combined treatment with hot water and UV light has not been conducted extensively. For these reasons, the effectiveness of a single treatment has various limitations, and therefore, a combination treatment, in this instance, of hot water and UV-C, is indicated. UV-C (0.25 J/cm2) and mild heat (51.3°C) was effective in reducing Saccharomyces cerevisiae by 5.0 Log CFU/cm2, and the reduction was ~2.0 Log CFU/cm2 greater than that of the single heat treatment [27]. In the current study, when UV-C (150 mJ/cm2) was applied after 5 min immersion in hot water at 60°C, the abundance of the target fungi decreased (p < 0.05) by 6.3–6.5 Log CFU/cm2. Fig. 3 summarizes the changes in the abundance of three fungi on the SS chips after treatment with UV-C and hot water (60°C). Cladosporium cladosporioides was not detected after each treatment; C. cladosporioides and P. oxalicum were not detected and decreased by more than 6 Log CFU/cm2 after 10 min of UV-C and 3 min of hot water immersion. Similar to these fungi, the abundance of A. niger also decreased (p < 0.05) by 4.0 Log CFU/cm2 with 3 min irradiation, and the fungus was not detected after 5 min. However, P. commune was more resistant to UV-C (150 mJ/cm2) than the remaining three strains and should, therefore, be treated for longer than 5 min in hot water. The maximum reduction on SS chips was > 6.5 Log CFU/cm2 when the sample was treated with a combination of 150 mJ/cm2 and water at 60°C (5 min). Therefore, while washing tools made of SS with UV-C rays in lukewarm water at least 60°C, we recommend sterilization under the above-mentioned conditions.
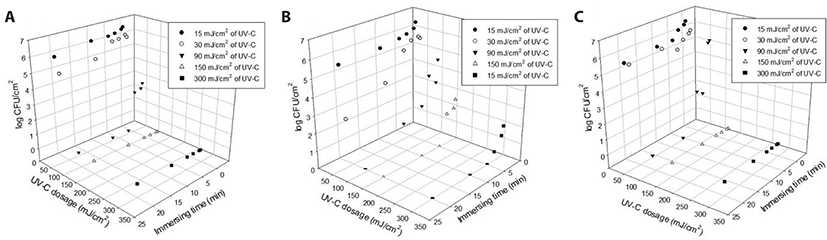
In conclusion, the present study demonstrated the prevalence of fungi during pig slaughter at each step in the process. To prevent fungal contamination of the carcass, four fungi were targeted in the following order of detection: A. niger, P. commune, P. oxalicum, and C. cladosporioides. Cladosporium was the most abundant and widely spread genus throughout the various sites, followed by Penicillium and Aspergillus. A reduction study was conducted on the four most prevalent fungi in the slaughterhouse. The combined treatment of UV-C irradiation and hot water had an inhibitory effect on A. niger, P. commune, P. oxalicum, and C. cladosporioides on the surface of SS chips. The maximum reduction on the SS chips was > 6.5 Log CFU/cm2 when the sample was treated with a combination of 150 mJ/cm2 and hot water at 60°C (5 min). These findings may contribute to reducing the growth of fungi in slaughterhouses and assist in the sanitization of tools (knives, hooks, and sharpeners) and facilities (ET), leading to the production of cleaner carcasses and meat products. The main limitation of this study was that the evaluations were conducted under laboratory conditions and were not applied practically to the treatments in pig slaughterhouses. Therefore, the selected optimal conditions in a slaughter processing facility should be tested further to confirm the results.