INTRODUCTION
In the worldwide animal husbandry industry, pigs account for a large proportion and are the primary source of energy and protein in developing countries [1]. Farms generally screen for boars with better fertility for breeding to enhance genetic value and fertility, but collecting fertility data about boars is time-consuming and labor-intensive [2,3]. The best approach is to use new technology to assist in reproduction. In vitro maturation (IVM) and in vitro fertilization (IVF) are techniques for the completion of fertilization in mammalian sperm and eggs in a manually controlled environment [4,5]. Although porcine embryos produced by IVM and IVF develop to the blastocyst stage, the low incidence of cleavage rate remains a significant impediment [6].
The osteopontin (OPN) protein, which has been identified as an indicator of human male fertility, promotes fertilization and preimplantation embryo development in mice. OPN has been shown to improve sperm capacitation and IVF efficiency in bovines [7,8]. Furthermore, when polyclonal anti-OPN antibodies were incubated with bovine sperm/oocytes in a culture medium, a reduction in sperm-egg binding and fertilization in vitro was observed [9]. The OPN concentration of high fertility bulls was four times that of low fertility bulls [10]. The expression level of OPN is positively correlated with sperm motility and the survival rate of piglets as determined by genotyping analysis in boars [11]. The addition of rat OPN to a modified Tris-buffered medium (mTBM) IVF system reduced polyspermy, improved porcine embryo development, and reduced apoptosis [12,13]. It has been reported that the concentration of OPN is related to the percentage of inactive sperm and litter rate to a certain extent [14]. These findings indicate to varying degrees that OPN plays an irreplaceable role in the development of mammalian gametes.
At present, the literature has agreed that OPN can increase the rate of division of mammalian embryos. Nevertheless, different results have been obtained regarding whether it affects sperm capacitation and the acrosome reaction. Given these additional results, this study’s objectives are to observe the effect of porcine OPN protein before and after capacitation on sperm motility, acrosome reaction, and embryo division by using more reliable experimental materials and methods.
MATERIALS AND METHODS
All procedures involving animals met the guidelines of “The Instructive Notions Concerning Caring for Laboratory Animals” issued by the Ministry of Science and Technology of China, with approval (Approval number SCAU#0025) by the Institutional Animal Care and Use Committee of South China Agricultural University (Guangzhou, China). All efforts were made to minimize animal suffering.
The samples were porcine spermatogonial stem cells (SSCs), sperm, 293FT cells, and Chinese hamster ovary (CHO)-K1 cells. According to the manufacturer’s protocol, total cellular RNA was isolated from samples using the TRIzol method (TIANGEN, Guangzhou, China) and reverse transcribed to cDNA (Vyzame, Nanjing, China). DNase I was used to remove genomic DNA, and first-strand cDNA was made from 1 μg of total RNA. Full-length coding sequences of porcine OPN (see Supplementary Table S1) were amplified from SSCs. The coding sequence of OPN with a 6His-tag (see Supplementary Table S2) was amplified and cloned into the PCDH-CMV-HSA-MCS-6His-EF1-EGFP+Puro vector by directional cloning using HindIII and BamHI sites. The PCDH-CMV-HSA-6His-OPN-6His-EF1-EGFP+Puro plasmid was transfected into 293FT cells. Reverse transcription-polymerase chain reaction (RT–PCR) was performed with primers for OPN and β-actin (Table 1) as confirmation. See the appraisal results for details in Supplementary Figs. S3 and S4, and Supplementary Table S3. 293FT cells and CHO-K1 cells were purchased from the American Type Culture Collection (ATCC). The lentiviral vectors included PCDH-CMV-MCS-EF1-EGFP+Puro plasmid and two lentivirus packaging plasmids (psPAX2 and pMD2G) (SBI, Los Angels, USA). The vector used was PCDH-CMV-HSA-MCS-6His-EF1-EGFP+Puro vector (Supplementary Fig. S1), modified from the PCDH-CMV-MCS-EF1-EGFP+Puro plasmid expressing HSA and 6His-tag. HSA is a signal peptide derived from a human serum protein and contains 63 bases (signal peptide sequence: MKWVTFISLLFLFASAYS). The addition of HSA dramatically increases the amount of protein secreted by CHO-K1 cells [15,16]. The 6His-tag is a tool for protein purification, and lentiviral production was performed (see Supplementary Fig. S2) as previously described [17].
Porcine OPN was obtained from using the CHO cell expression system [18,19]. CHO-K1 cells were resuscitated, passaged, and plated. After culturing for 24 h, lentivirus supernatant and 10 μg/mL polybrene were added to the cells. Green fluorescent protein and Puro (6 μg/mL) selection were performed to select a single clone. After screening the monoclonal CHO cell line, the supernatant was collected, and the cell debris was removed by centrifugation. After concentration, porcine OPN protein was purified by 6His-tag and stored in freeze-dried powder.
Recombinant porcine OPN (1 mg/mL, in phosphate-buffered saline [PBS]; 92% pure) was produced in house. The OPN protein was diluted to 0.1 μg/mL in IVF medium (113.1 mM NaCl, 3.0 mM KCl, 7.5 mM CaCl2-H2O, 20.0 mM tris, 11.0 mM D-glucose 5.0 mM sodium pyruvate, 2 mg/mL bovine serum albumin [BSA] and 2 mM caffeine). The rabbit polyclonal antibody against OPN (82 mg/mL) was purchased from Abcam (ab8448, Cambridge, MA, USA) and diluted (1:50, 1:200, 1:500, 1:1000 and 1:2000) in IVF medium. Normal rabbit IgG (1 mg/mL, in PBS) was purchased from Abcam (ab37415) and diluted (1:50, 1:200, 1:500, 1:1000 and 1:2000) in IVF medium. A blank control (only IVF medium) was also prepared.
Fresh ejaculates were collected from 6 Landrace 12-month boars according to the “gloved-hand” technique [20], diluted and stored in 17°C fantastic boxes, and then brought to the laboratory within 1 h of collection. For the sperm assay, the sperm samples were divided into four groups.
The first group was for the location and expression of OPN protein before and after capacitation sperm. The second group was used for the cyclic adenosine monophosphate (cAMP) assay after capacitation of sperm. The third group was for acrosome reactions in sperm. In this experiment, sperm samples were divided into 4 groups according to different treatments: blank control (untreated), normal IgG treated (negative control), anti-OPN treated, and OPN protein treated incubation for 4 h. The fourth group was for IVF. In this experiment, sperm samples were collected and capacitated for evaluating penetration, polyspermy and embryo division during IVF.
The semen was washed three times with sperm-washing medium (Dulbecco’s PBS supplemented with 0.1% BSA) and resuspended with mTBM [12]. The sperm resuspension was subjected to a discontinuous Percoll gradient (90% [v/v] and 45% [v/v]) to remove dead sperm and seminal plasma [21] and then centrifuged at 300 × g for 20 min. The concentrated sperm of each sample was adjusted to 55 × 106 spz/mL and added to the bottom of a tube with 2 ml of mTBM containing 100 μg/mL heparin sodium. The sperm were then incubated (38.5°C, 5% CO2) for sperm capacitation for 30 min with the sperm swimming-up method to reduce polyspermy during IVF [22,23]. The washed sperm samples were divided into 2 groups: before capacitation and after capacitation. The concentrated sperm of each sample was added to the bottom of a tube with 2 mL of mTBM containing 100 μg/mL heparin sodium. Tyrosine phosphorylation protein is a marker of capacitation changes observed in boar sperm, and its expression significantly increases after capacitation [24].
In addition to Hoechst 33342 (1:100; H3570, Life Technologies, Carlsbad, CA, USA), the antibodies used in this experiment were as follows: anti-OPN antibody (1:500); normal rabbit IgG (1:500); fluorescein-conjugated goat against rabbit IgG (1:1000; A11034; Alexa Fluor 488, Thermo Fisher Scientific, Waltham, MA, USA); and fluorescein-conjugated goat against rabbit IgG (1:1000; A11036; Alexa Fluor 568, Thermo Fisher Scientific).
The OPN protein in sperm was detected by Immunofluorescence (IF) staining as previously described [25]. After the sperm were washed 3 times with PBS, the sperm concentration was adjusted to 55 × 106 spz/mL. Fifty microliters of sperm suspension was placed on slides and then dried for 60 min in air. The cells were cultured in a 24-well culture plate for 24 h and washed 3 times with PBS. Then, the samples were fixed with 3.7% formaldehyde at room temperature (RT) for 20 min, washed with PBS, blocked with 10% normal rabbit serum for 1 h, and incubated with sufficient diluted primary anti-OPN overnight at 4°C. The samples were then exposed to fluorescein-conjugated secondary antibodies at 37°C for 1 h and incubated with Hoechst for 5 min to protect them from light. Each sample was supplemented with an antifading solution. OPN protein was detected by laser scanning confocal microscopy (LSM780, Zeiss, Germany).
The prepared samples (55 × 106 cells/mL) containing cells before and after capacitation boar sperm were homogenized in ice-cold protein extraction buffer (KeyGEN BioTECH, Guangzhou, China) to collect soluble protein. The protein (20 μg lysates) was boiled for 10 min, subjected to 12% sodium dodecyl sulfate polyacrylamide gel electrophoresis (SDS–PAGE), transferred to a polyvinylidene fluorid (PVDF) membrane (Roche Diagnostics), and blocked with 5% nonfat dried milk in Tris-buffered saline-Tween 20 for 1 h at RT. Western blotting (WB) analysis was carried out overnight at 4°C for primary antibodies and at 37°C for 1 h for secondary antibodies. Immunodetection of OPN/tyrosine phosphotyrosine proteins was performed by enhanced chemiluminescence (ECL). Then, the membranes were stripped and reprobed with alpha-tubulin (α-tubulin)/beta-actin (β-actin) as loading standards.
The antibodies used were anti-OPN (1:1000; ab8448, Abcam), mouse monoclonal phospho-tyrosine antibody (clone P-Tyr-100) (diluted 1:1000; 9411 s, Cell Signaling Technology, Danvers, MA, USA), mouse monoclonal anti-α-tubulin (clone B-5-1-2) (1:3000; T6074, Sigma-Aldrich, St. Louis, MO, USA), mouse monoclonal anti-β-actin (clone AC-15) (1:3000; A1978, Sigma-Aldrich), mouse monoclonal anti-GFP antibody (clone GSN149) (1:1000; G1546, Sigma-Aldrich), HRP AffiniPure goat anti-mouse IgG (1:3000; E030110; Earth Ox, Millbrae, CA, USA), and HRP AffiniPure goat anti-rabbit IgG (1:3000; E030120, Earth Ox).
To analyze the cAMP concentration in sperm, after capacitation, sperm samples were analyzed using a cAMP direct immunoassay kit (Abcam) [25]. Sperm samples included the OPN protein group, anti-OPN group, and control group (nothing added). Sperm samples (55 × 106 spz/mL) were lysed with protein lysis buffer for the cAMP assay. The optical density (OD) was measured at 405 nm with an ELISA plate reader. For the porcine sperm cAMP assay, each experiment included a standard well, sample well, control well and blank well. Detailed experimental steps were described according to the manufacturer’s instructions. A standard curve correlation coefficient > 0.95 was needed. Six replicates were performed for each assay. The cAMP concentration was calculated for each sample by the standard curve provided by the kit’s manufacturer.
The acrosome reaction was measured with a fluorescein isothiocyanate-conjugated Pisum sativum agglutinin (FITC-PSA; L0770, Sigma-Aldrich) label labeled the acrosomal matrix glycoproteins. Propidium iodide (PI; P4170, Sigma-Aldrich) was used to determine the number of dead sperm. After washing twice in PBS, after 4 h of capacitation, sperm (55 × 106 spz/mL) were incubated with PI (5 μg PI/1 mL PBS) for 10 min at RT. Samples were incubated with a FITC-PSA solution (10 μg FITC-PSA/1 mL PBS) at 37°C for 30 min in the dark and analyzed. Acrosome-intact sperm were stained with weak fluorescence. The presence of green acrosomal fluorescence on the top of the sperm head or equator indicated an acrosome reaction. Immunofluorescent staining was analyzed by a BD FACSCalibur flow cytometer (FCM) using CellQuest software (BD Biosciences, San Jose, CA, USA). All flow cytometry analyses were conducted within 1 h of Prostate specific antigen (PSA) staining.
Unless otherwise stated, all chemicals were purchased from Sigma–Aldrich, and the media were purchased from Gibco (Thermo Fisher Scientific). The IVF procedure was based on a previous study with some modifications [26]. Oocyte maturation medium (TCM199; Gibco BRL, Rockville, MD, USA) was supplemented with 0.1 mg/mL L-cysteine, 10 ng/mL epidermal growth factor (EGF), 10% (v:v) fetal bovine serum (FBS), 10% (v:v) porcine follicular fluid (PFF), and hormones (10 IU/mL human chorionic gonadotropin [hCG] and 10 IU/mL pregnant mare serum gonadotropin [PMSG]). L-cysteine, EGF, hCG, and PMSG were dissolved in TCM199 medium in a concentrated dilution, filtered through 0.22 μm filters, and stored at -20°C until use. PFF was obtained from 3-6 mm diameter follicles and centrifuged at 1900×g and 4°C for 30 min. The supernatant fluid was filtered through 0.45 μm and 0.22 μm filters and stored at −20°C until use.
Ovaries were collected within 2 h from slaughtered prepubertal pigs and stored in 0.9% sodium chloride (NaCl) solution at 37°C. Cumulus-oocyte complexes (COCs) were selected from ovarian follicles (3–6 mm in diameter) and precipitated three times in Dulbecco phosphate-buffered saline (DPBS)-poly(vinyl alcohol) (PVA) solution. Fifty COCs were transferred to oocyte maturation medium for the first 22 h in a four-well Nunclon plate (176740; Gibco; Thermo Fisher Scientific) containing 500 μL of oocyte maturation medium at 38.5°C (5% CO2) and then cultured in oocyte maturation medium without hormones for another 22 h [22,27]. Each oocyte maturation medium was previously equilibrated at 38.5 C and 5% CO2 in air for 3 h.
The sperm cells were treated as described above (sperm assay). Oocytes that showed the first polar body and homogeneous cytoplasm were placed into IVF medium. Approximately 10 oocytes were placed into a droplet of 40 μL IVF medium covered with mineral oil that had been equilibrated for 6 h. Ten microliters of sperm solution at a concentration of 5 × 106 spz/mL was added to the oocyte-containing IVF medium to obtain a sperm concentration of 1 × 106 spz/mL. Fifty oocytes were cultured in 500 μL of porcine zygote medium (PZM) in a four-well Nunclon plate at 38.5°C in a humidified atmosphere of 5% CO2, 5% O2 and 90% N2 [12], and penetration, polyspermy and the cleavage rate of the embryos were determined.
The experimental data of the percentages for the acrosome reaction (AR) and the cAMP measurements were analyzed with the Statistical Package for the Social Science (SPSS version 18.0, SPSS, Chicago, IL, USA) software. Data were tested for normal distribution and homogeneity of variance. cAMP significance testing was performed using the T test followed by the least-significant difference (LSD) test. P values < 0.05 were considered significant, and the data are expressed as the SEM derived from three independent experiments. Experiments were repeated nine times. One-way repeated-measures ANOVA was used to analyze the percentages for each response category (AR and acrosome-intact). All dependent variables were examined for normality using the Wilcoxon test (SAS Institute, Cary, NC, USA). The penetration rate and polysaccharide fertilization rate were arcsine transformed. The sperm number penetrated in oocytes was logarithmically transformed to approach a normal distribution. Significance testing was performed using a t test and one-way ANOVA, and the data are expressed as the mean ± SEM. Experiments were repeated at least three times, and the data were pooled for analysis.
RESULTS
Before sperm capacitation, OPN was located in the neck of the sperm (Fig. 1B). When the sperm were capacitated, OPN was detected in the acrosome, neck, and tail of the sperm (Fig. 1E). In addition, OPN expression increased after sperm capacitation (Fig. 1G). Tyrosine-phosphorylated proteins were probed with an anti-phosphorylated antibody, showing increases after capacitation (Fig. 1H).
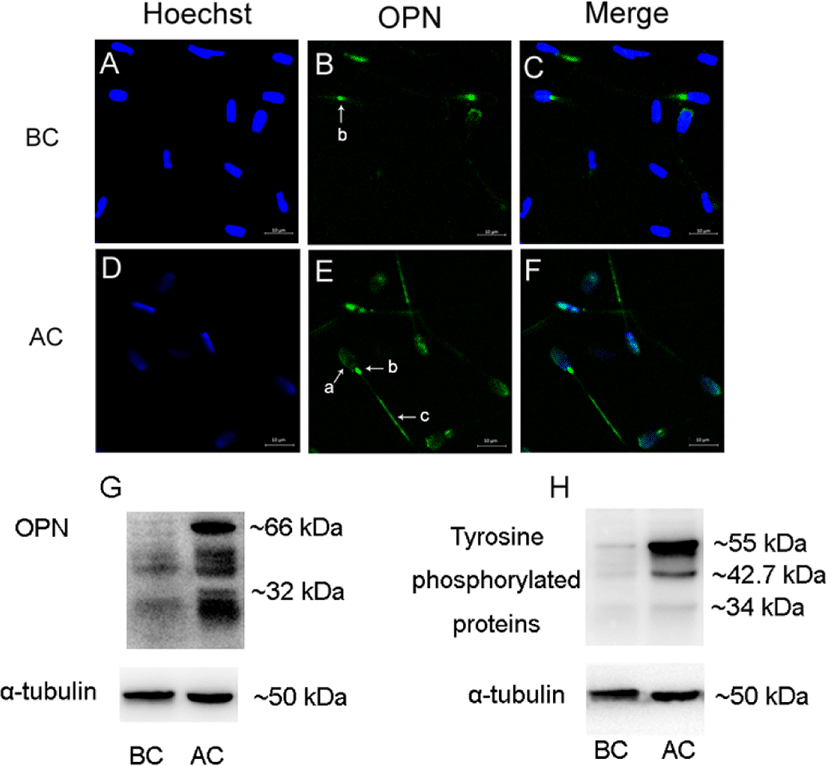
Measurement of cAMP content in sperm treated with OPN protein for 4 h. The cAMP concentration in OPN-treated sperm was significantly higher (p < 0.01) than that in untreated control sperm (5.962 ± 0.196 pmol vs. 5.144 ± 0.180 pmol). For the anti-OPN-treated group, the cAMP concentration in sperm was significantly decreased (p < 0.01) compared to the untreated sperm (4.163 ± 0.164 pmol vs. 5.144 ± 0.180 pmol). Data are expressed as the mean ± SEM (Table 2).
Control (pmol) | OPN | anti-OPN antibody |
---|---|---|
5.144 ± 0.180 | 5.962 ± 0.196 | 4.163 ± 0.164 |
There was no significant difference between the blank control and negative control (4 h). The fluorescence of the OPN protein group was significantly higher than that of the other three groups (Fig. 2). The fluorescence intensity of the anti-OPN group was significantly lower than that of the other three groups. This suggests that OPN can significantly improve the acrosome reaction of sperm.
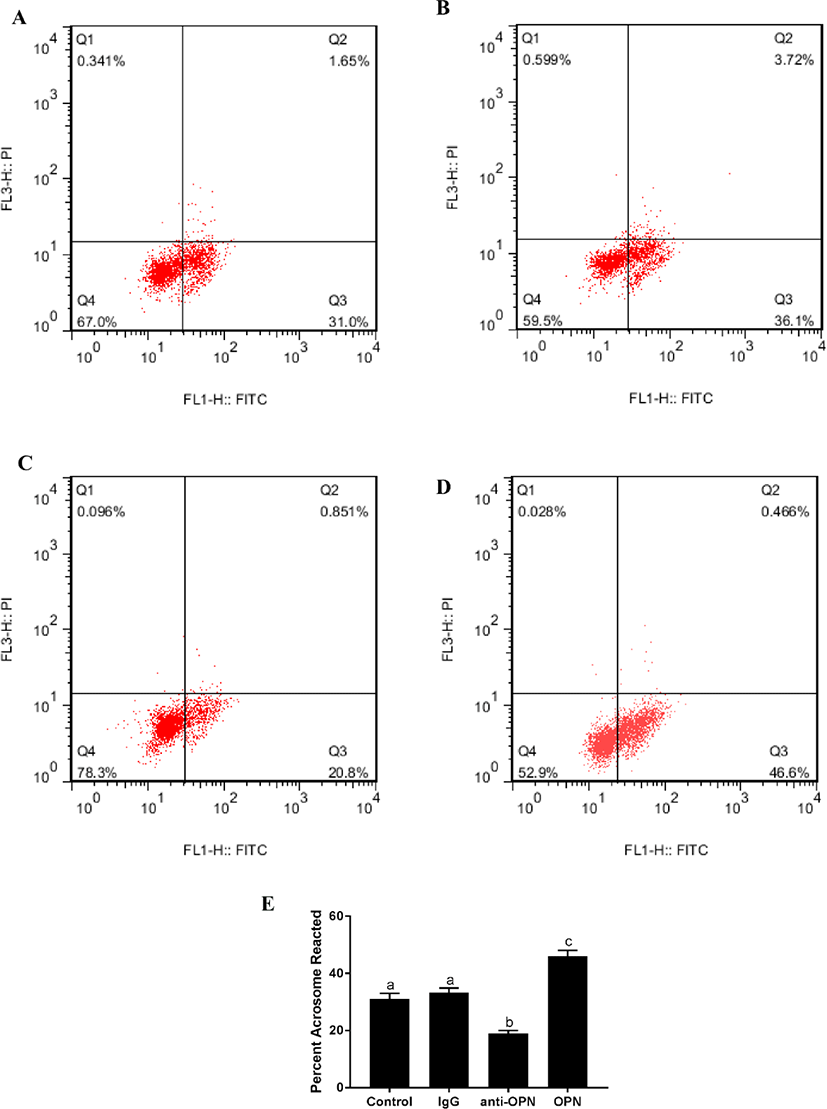
Normal rabbit IgG, porcine OPN protein, and anti-OPN antibody were added to IVF medium separately. Based on the cleavage results, a dilution of the anti-OPN antibody of 1:1000 was used (p < 0.05) (Table 3). The IgG concentration was not related to embryo division (Table 4). The difference in the cleavage between the OPN protein group and control group was significant, with higher cleavage in the former (p < 0.01); that between the anti-OPN antibody group (1:1000) and the control group was also significant, with lower cleavage in the former (p < 0.05). In addition, sperm treated with 0.1 μg/mL OPN protein had significantly increased permeability and polyspermy. The antibody group showed reduced penetration and cleavage and increased polyspermy (Table 5).
Anti-OPN antibody | No. of oocytes | Cleavage at 48 h (%) |
---|---|---|
0 | 207 | 52.54 ± 0.90a |
1:50 | 186 | 50.48 ± 0.28a |
1:200 | 196 | 49.11 ± 0.93a |
1:500 | 182 | 48.58 ± 1.35b |
1:1,000 | 195 | 43.03 ± 2.52c |
1:2,000 | 201 | 47.63 ± 1.74b |
IgG | No. of oocytes | Cleavage at 48 h (%) |
---|---|---|
0 | 232 | 53.21 ± 1.20 |
1:50 | 213 | 52.32 ± 1.08 |
1:200 | 182 | 54.65 ± 0.43 |
1:500 | 196 | 51.46 ± 1.52 |
1:1000 | 215 | 54.73 ± 2.18 |
1:2000 | 233 | 53.92 ± 1.74 |
DISCUSSION
In previous studies, the literature agreed on the results of OPN’s ability to promote embryo cleavage, but it is controversial through which pathways work [8,12]. In this study, we found that OPN protein diffused from the neck of the sperm to the head and tail before and after sperm capacitation and significantly increased its expression. This suggests that OPN may be involved in specific processes in the capacitation of sperm. Sperm capacitation is a prerequisite for the sperm acrosome reaction and egg binding. During the capacitation period, membrane permeability and intracellular signaling pathways are actively changed [28]. In a previous study, OPN treatment of noncapacitated sperm did not change the motility of sperm, but it significantly promoted the rate of embryonic division [12]. This may be because in IVF, sperm are subjected to capacitation and will be in a state of continuous capacitation before sperm-egg binding. We obtained new findings when we were incubated with OPN after sperm capacitation. This suggests that OPN is likely to affect sperm performance by binding membrane proteins to activate membrane signals during sperm capacitation.
To further explore the effects of OPN on sperm during capacitation, we tested it at the molecular level. At the molecular level, capacitation involves cholesterol removal and modifications of plasma membrane lipids; activation of the cAMP/protein kinase A/tyrosine kinase (cAMP/PKA/TK) signaling pathway that causes an increase in protein tyrosine phosphorylation (Tyr phosphorylation) and protein phosphatase inactivation; increases in intracellular pH and Ca2+ concentration; and sperm plasma membrane potential hyperpolarization, actin polymerization, and cytoskeleton remodeling [29,30]. Furthermore, the synthesis and activation of cAMP occur in the initiation phase of the capacitation reaction, which directly affects the efficiency of the sperm acrosome reaction [31–33]. Since the subsequent influence of OPN on the acrosome reaction ability of sperm was examined, we selected cAMP as a marker to measure the state of capacitation. In the present study, the cAMP content in the capable processing sperm of the experimental group was significantly higher than that of the control group after 4 hours of incubation. This suggests that OPN affects the physiological state of the cells when sperm are capacitated.
Although the literature agrees on the results of OPN’s ability to promote embryo cleavage, previous studies have shown inconsistent results regarding the effects of OPN on sperm acrosome reactions. Hao found that OPN could promote the cleavage rate of pig embryos but reduce the acrosome reaction ability of sperm. In contrast, Monaco found that OPN enhances the ability of sperm to produce acrosome reactions and increases the rate of bovine embryonic division [7,8,12]. In this study, we used FCM technology to test the ability of sperm to react with acrosomes and found that OPN can significantly improve the acrosome reaction ability of sperm, and it is more reliable than using eye observation to detect sperm state. Since Hao performed experiments on porcine sperm with murine OPN, however, the cDNA sequence of OPN in pigs does not exhibit high homology with the corresponding sequences in humans, rhesus monkeys, cattle, rats, mice, and chickens, with similarity ranging from 31.2% to 80% [34]. We used an optimized porcine OPN protein in this study. In addition to the results of the acrosome reaction, there are different results in processing time, most likely due to the effects of protein sources. In addition, we added an antibody-treated control group to IVF, which is more convincing.
Observations of the induction of sperm capacitation suggest that assessing the integration of OPN in IVF medium can increase fertilization rates and improve IVF efficiency. Compared to previous studies, we added antibody sets to the IVF trial to better describe the effect of OPN on fertilization. The IVF results showed that OPN increased the total penetration rate and embryo division and reduced polyspermy. Notably, the recombinant porcine OPN protein had a better effect on the capacitation, acrosome reaction, and fertilization efficiency of pig sperm at the same concentration (0.1 μg/mL).
CONCLUSION
In previous studies, the detection of OPN protein function in porcine sperm was mainly used as a murine protein in IVF. The results were inconsistent with whether OPN promoted or inhibited the acrosome reaction. We reconstituted the porcine OPN protein and found that it can enhance the acrosome reaction and embryo division. Compared with previous studies, we used more reliable experimental materials and a more accurate IVF protocol to detect whether OPN promotes and inhibits the sperm acrosome reaction and further found that the cAMP concentration changed during sperm capacitation by OPN treatment. In conclusion, this study found that 0.1 μg/mL porcine OPN protein can significantly enhance cAMP concentration after capacitation sperm, acrosome reaction ability, and embryo division during IVF and provides new clues to explore the mechanism of OPN’s function on sperm.