INTRODUCTION
The immune system protects the body from foreign bacterial or viral infections and reduces their susceptibility to diseases [1]. Therefore, improving immune activities using natural bioactive compounds is an effective strategy to defend the body [2–5]. Many natural bioactive compounds are known to activate macrophages that play various roles, such as secretion of cytokines, phagocytosis, wound repair, and antigen presentation, in the immune system [6]. The increased production of inflammatory mediators, such as cyclooxygenase (COX)-2, nitric oxide (NO), and inflammatory cytokines (e.g., interferon [IFN]-γ, interleukin [IL]-6, IL-8, IL-10, IL-1β, and tumor necrosis factor [TNF]-α) in macrophages, help to eliminate foreign particles [7].
Egg proteins are a good natural source for producing functional peptides [8]. Egg contains various functional proteins such as ovotransferrin (OTF), ovalbumin, lysozyme, ovoinhibitor, ovomucoid, phosvitin, lipovitellin, livetins, etc. [9]. Some of them are reported to have immune-modulatory activity by stimulating the production of cytokines, activating signaling pathways, and activating immune cells [2,10–12]. OTF is the second major egg white protein and is reported to account for about 12%–13% of egg white protein [13]. OTF has a molecular weight of 78 kDa and has 15 disulfide bonds in a protein structure consisting of 686 amino acids. OTF has antimicrobial activity because of possessing two iron-binding sites where iron ions can bind [14]. OTF is also known to have antiviral [15], anticancer [16], antioxidative, and immunomodulatory activities [17].
Enzymatic hydrolysis is a common method to produce functional peptides from proteins [18], because the same peptides in the native proteins do not show biological activities [19]. It is known that the functional activity of peptides depends on the characteristics of peptide, such as amino acid sequence, length, and the hydrophobic to hydrophilic amino acids ratio in the peptide [20]. Thus, producing functional peptides using a variety of proteolytic enzymes is a better strategy than using a single enzyme. While many researchers purified and identified specific peptides to observe their functionalities, some researchers investigated the functional activity of crude enzymatic hydrolysates [16,21]. Our previous study found that OTF enhanced the immune activity of macrophages by activating the mitogen-activated protein kinase (MAPK) pathway [13]. In present study, we determined the enhancement of the immune-enhancing activity of OTF after enzymatic hydrolysis.
MATERIALS AND METHODS
Bromelain (from the pineapple stem), pancreatin (from the porcine pancreas), and papain (from the papaya latex) for enzymatic hydrolysis were obtained from Sigma-Aldrich (St. Louis, MO, USA). Dulbecco modified Eagle medium (DMEM), antibiotics (containing penicillin and streptomycin), fetal bovine serum (FBS), and phosphate-buffered saline (PBS) were obtained from Hyclone (Logan, MI, USA). Mouse IL-6 and TNF-α ELISA kits were obtained from AB Frontier (Seoul, Korea). SB 202190, PD 98059, and SP 600125 were purchased from Abcam (Cambridge, UK). Thiazolyl blue tetrazolium bromide (MTT), lipopolysaccharides (LPS), Neutral Red, and Griess reagent were obtained from Sigma-Aldrich. Ethyl alcohol, acetic acid, and dimethyl sulfoxide (DMSO) were obtained from Samchun (Seoul, Korea). All other chemical reagents used in this study were of analytical grade.
OTF was isolated from egg white according to the method of Abeyrathne et al. [22]. The yield and purity of isolated OTF were greater than 83% & 85%, respectively. To produce OTF hydrolysates, we have used various enzymes such as alcalase, bromelain, flavourzyme, neutrase, pancreatin, papain, pepsin, and protamex. However, except for bromelain, pancreatin, and papain enzyme hydrolysates, other enzyme hydrolysates were found to have no ability to promote NO production in macrophages, confirming that they had no immune-enhancing activity. Therefore, bromelain, pancreatin, and papain were used in this study. The lyophilized OTF (2 g) was dissolved in distilled water (DW, 100 mL), the pH adjusted to pH 7.0 for bromelain, pancreatin and to pH 6.5 for papain, and then incubated at 50°C for bromelain, pancreatin and at 65°C for papain. Each enzyme was added in a 1:50 ratio (enzyme:substrate), and incubated for 4 h. After incubation, the hydrolysis was terminated by heating at 100°C for 10 min. Finally, the hydrolysate was centrifuged, and the supernatant was lyophilized. The OTF hydrolysates produced by bromelain, pancreatin, and papain were named as OTH-Bro, OTH-Pan, OTH-Pap, respectively.
RAW 264.7 cells were purchased from Korean Cell Line Bank (Seoul, Korea) and cultured in DMEM medium supplemented with 1% antibiotics and 10% FBS at 37°C humidified 5% CO2 incubator.
MTT assay was used to determine cell viability [23]. Briefly, RAW 264.7 cells were cultured in 96-well plate at a density of 2 × 105 cells/well, and the cells were treated with OTF hydrolysates (250 and 500 μg/mL) for 24 h. Subsequently, cells were treated with a MTT solution (2.5 mg/mL) and further cultured for 4 h. After removing the supernatant, DMSO was added to each well and the absorbance was measured by microplate reader (Bio-Rad, Hercules, CA, USA) at 570 nm.
The Griess reagent was used to determine the effects of OTF hydrolysates on NO production of RAW 264.7 cells [24]. Briefly, RAW 264.7 cells were cultured in a 96-well plate at a density of 2 × 105 cells/well, and the cells were treated with OTF hydrolysates (250 and 500 μg/mL) for 24 h. After transferring the supernatant to a new 96-well plate, Greiss reagent was added and reacted for 15 min. The absorbance was measured at 540 nm. NO concentration was calculated from a standard curve obtained using sodium nitrate.
The quantitative real-time polymerase chain reaction (qRT-PCR) assay was used to measure the effects of OTF hydrolysates on inducible nitric oxide synthase (iNOS) production of RAW 264.7 cells. Briefly, RAW 264.7 cells were cultured in a 6-well plate at a density of 1 × 106 cells/well, and incubated for 24 h. Thereafter, the cells were incubated with OTF hydrolysates (250 and 500 μg/mL) for an addition 24 h. The RNA was isolated from the cells using an RNA isolation kit (Qiagen, Milan, Italy) and the isolated total RNA was synthesized into cDNA using the cDNA synthesis kit (Thermo Fisher Scientific, Carlsbad, CA, USA). The iNOS mRNA expressions were analyzed using the SYBR Green reagent (PhileKorea, Daejeon, Korea) on a qRT-PCR system (PikoReal™, Thermo Fisher Scientific). The amplified data were analyzed using the comparative cycle threshold method and were normalized using the expression level of β-actin. The primer sequences (5’-3’) were shown as follows: iNOS, forward CCCTTCCGAAGTTTCTGGCAGCAGC, reverse GGCTGTCAGAGCCTCGTGGCTTTGG’; and β-actin, forward GTGGGC CGCCCTAGGCACCAG, reverse GGAGGAAGAGGATGCGGCAGT.
The effects of OTF hydrolysates on pro-inflammatory cytokine (TNF-α and IL-6) production of RAW 264.7 cells were determined by using an ELISA assay. Briefly, RAW 264.7 cells were cultured in a 12-well plate at a density of 4 × 105 cells/well and incubated for 24 h. Thereafter, the cells were incubated with OTF hydrolysates (250 and 500 μg/mL) for an addition 24 h. The amounts of pro-inflammatory cytokines were measured by an ELISA kit according to the manufacturers’ instructions.
The neutral red uptake method was used to measure the effects of OTF hydrolysates on the phagocytic activity [25]. Briefly, RAW 264.7 cells were cultured in a 24-well plate at a density of 2 × 105 cells/well and incubated for 4 h. Thereafter, the cells were incubated with OTF hydrolysates (250 and 500 μg/mL) for an additional 24 h. After removing the supernatant, neutral red solution (0.075%, dissolved in PBS) was added to cells and incubated for another 1 h. The cells were washed 3 times with PBS, the neutral red was dissolved by adding the lysis reagent. The absorbance was measured at 540 nm.
The blocking assay using specific inhibitors of p38, ERK, or JNK pathway (SB 202190, PD 98059, and SP 600125) was conducted [2]. Briefly, RAW 264.7 cells were cultured in a 12-well plate at a density of 4 × 105 cells/well and incubated for 24 h. Thereafter, the cells were incubated with 500 μg/mL of OTF hydrolysates and each 10 μM specific inhibitors for 8 h. The cells were then incubated for an additional 24 h after being replaced with a fresh medium. The amounts of production of NO, TNF-α, and IL-6 were determined as described above.
The data were analyzed with SPSS statistics 18.0 (SPSS, Chicago, IL, USA) and presented as the mean ± SD from triplicate measurements of the analyses. The student’s t-test and one-way analysis of variance (ANOVA, followed by Duncan’s multiple comparison procedure) were used to measure the differences.
RESULTS AND DISCUSSION
The effects of OTF hydrolysates on cell viability are shown in Fig. 1. The viability of RAW 264.7 cells was not affected by the OTF hydrolysates. All treatment groups showed over 95% cell viability, indicating that OTF hydrolysates (250 and 500 μg/mL) exhibited no toxic effect on the RAW 264.7 cells. In contrast, cell viability was significantly reduced at concentration of OTF hydrolysates higher than 500 μg/mL (data not shown).
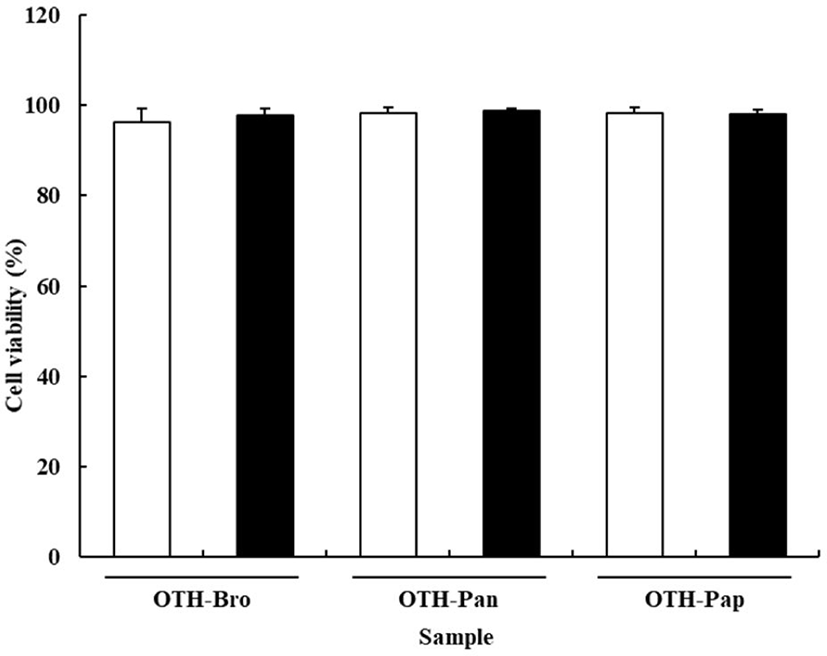
NO is one of the most important molecules in immune response, which is secreted as a free radical when macrophages are activated. Furthermore, it is well known to be lethal to intracellular parasites and bacteria [3]. Our previous study indicated that OTF stimulated NO production in RAW 264.7 cells [13]. The results showed that all the OTF hydrolysate groups increased the production of NO (Fig. 2A). At 500 μg/mL level, the OTF-treated RAW 264.7 cells produced NO at 17.96 ± 3.76 μM. However, the OTF-hydrolysates-treated groups (OTH-Bro, OTH-Pan, and OTH-Pap) produced more NO (24.22 ± 3.63, 30.15 ± 3.26, and 27.50 ± 2.85 μM, respectively.) than the OTF-treated group. At lower concentrations (250 μg/mL), the OTF-hydrolysates-treated groups also produced more NO than the OTF-treated group.
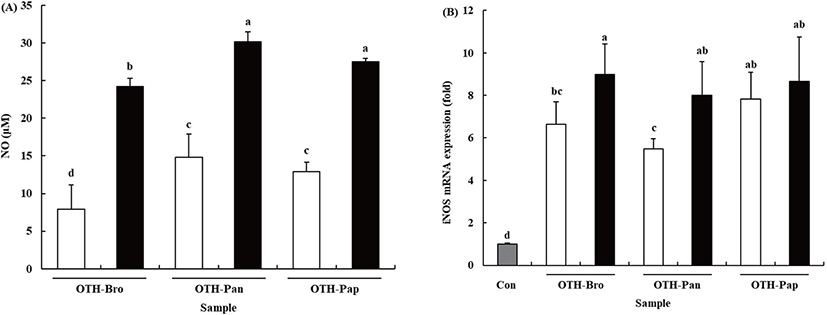
NO is synthesized by a family of nitric oxide synthase (NOS) with three isoforms: endothelial NOS (eNOS), neuronal NOS (nNOS), and iNOS [26]. Among them, nNOS and eNOS regulate NO concentration under normal conditions. However, iNOS induces a higher amount of NO under inflammatory situations [27]. Therefore, iNOS is strongly related to the increased NO production.
OTF hydrolysates increased the mRNA expression level of iNOS in RAW 264.7 cells (Fig. 2B, p < 0.05). Compared with the control, all OTF-hydrolysate-treated groups showed increased iNOS mRNA expression. At 500 μg/mL concentration, OTH-Bro showed the most increased iNOS mRNA (8.99-fold) expression level. The OTH-Pap and OTH-Pan groups showed an 8.65-fold and 7.99-fold increase in the level of iNOS mRNA, respectively. At lower concentrations (250 μg/mL), the OTH-Pap showed the highest iNOS mRNA expression level (7.82-fold), which was similar to that at 500 μg/mL level. OTH-Bro and OTH-Pan showed a 6.63-fold and 5.48-fold increase in iNOS mRNA, respectively. These results indicated that all OTF hydrolysates increased the expression level of iNOS mRNA, resulting in increased NO production in RAW 264.7 cells.
Pro-inflammatory cytokines, the key regulators of the immune response, are produced in the macrophages when they are activated [28]. Treating RAW 264.7 cells with OTF hydrolysates increased TNF-α and IL-6 production (p < 0.05) (Fig. 3). At 500 μg/mL OTF hydrolysates level, all OTF hydrolysates increased TNF-α production more than 50 ng/mL (OTH-Bro: 54.60 ± 5.23, OTH-Pan: 53.46 ± 6.76, OTH-Pap: 54.14 ± 6.13 ng/mL) in the RAW 264.7 cells, but no significant difference was found among the hydrolysates. At 250 μg/mL concentration, OTH-Pap showed significantly higher (p < 0.05) TNF-α production than other hydrolysates. Treating RAW 264.7 cells with OHT-Pap and OTH-Pan showed higher IL-6 production than OTH-Bro at all concentrations. The 500 μg/mL of OTH-Pap and OTH-Pan increased the IL-6 production (22.94 ± 2.80 and 22.18 ± 2.09 ng/mL, respectively), while the amount produced was 14.63 ± 0.94 ng/mL with OTH-Bro treatment.
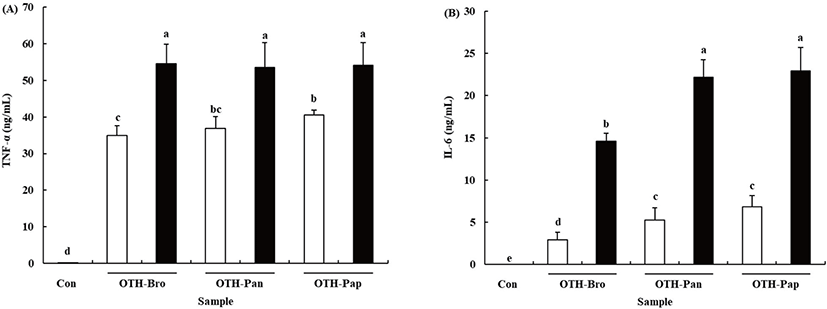
Cytokines are linked to innate and adaptive immunities and play important roles in the activation of macrophages [29]. The TNF-α is one of the cytokines released first when macrophages are activated. It upregulates cell adhesion molecules that initiate the migration of inflammatory cells into tissues and activate the secretion of other cytokines and the reactive oxygen species [30]. The IL-6, which serve to promote the differentiation of lymphocytes, is a pivotal cytokine in immune response [31]. Previous studies showed that many natural materials derived from plants, animals, and fishes have immune-enhancing or stimulating activity by boosting the production of pro-inflammatory cytokines in various immune cells [1,2,24,31]. Thus, OTF hydrolysates boost the immune system by promoting the secretion of pro-inflammatory cytokines.
Phagocytic activity is one of the characteristics of macrophages to defend the host against pathogens [32]. The neutral red uptake assay was used to confirm the effect of OTF hydrolysates on phagocytic activity of macrophages. All OTF hydrolysate groups showed increased phagocytic activity compared than the control group (p < 0.05) (Fig. 4). The OTH-Pan group (500 μg/mL) showed the highest phagocytic activity (123.27% of the control), whereas the OTH-Pap group (250 μg/mL) showed the lowest phagocytic activity (107.18% of the control), suggesting that OTF hydrolysates help eliminate foreign pathogens and improve the immune function of the host [4].
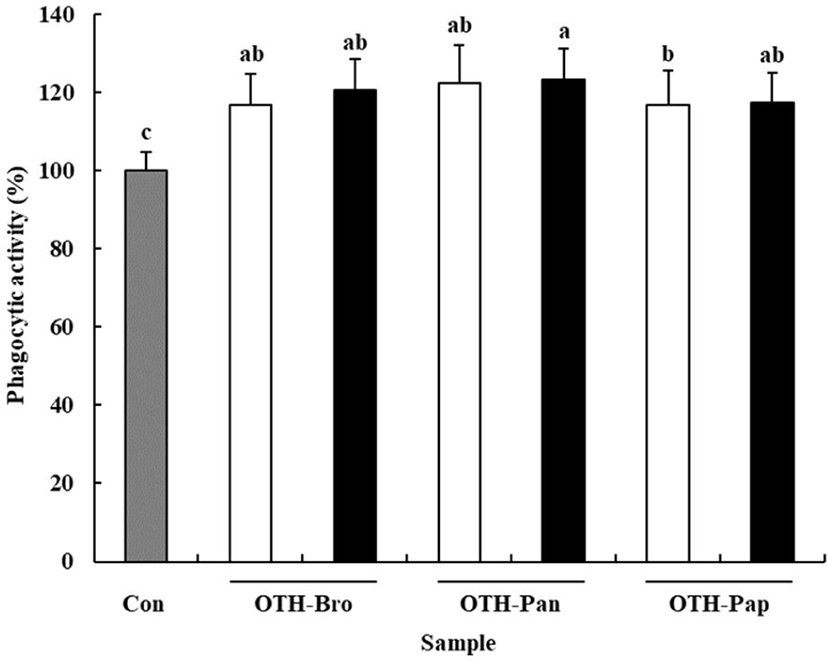
To investigate the activation mechanism of macrophages by OTF hydrolysates further, we conducted specific inhibitor studies using mitogen-activated protein kinase (MAPK) signaling pathway inhibitors [2]. The inhibitors used were SB 202190, PD 98059, and SP 600125, which inhibit p38, ERK, and JNK, respectively. As shown in Figs. 5A,B and C, all OTF hydrolysates (500 μg/mL) increased the production of NO in macrophages. However, the macrophages treated with specific inhibitors (SB 2002190 and SP 600125) had a lower level of NO production than the control (without treated any inhibitors) (p < 0.001). SP 600125 treatment, which inhibits the JNK, showed the highest decrease in the rate among the specific inhibitors. However, PD 98059, which inhibits the ERK, showed an increased NO production level than the control (p < 0.001). Similar to our study, Youn et al. [33] reported that PD 98059 did not affect NO production. For TNF-α, all three MAPK inhibitors affected the TNF-α production induced by OTF hydrolysates (Figs. 5D,E and F). SP 600125 treatment group showed the highest decrease (p < 0.001). Unlike NO, TNF-α production was inhibited by PD 98059 (OTH-Bro, p < 0.001; OTH-Pan, p < 0.001; OTH-Pap, p < 0.01). The production of IL-6 induced by OTF hydrolysates were also inhibited by all three MAPK inhibitors (Figs. 5G,H and I). Similarly, the SP 600125 treatment group significantly inhibited the production of IL-6 in all OTF hydrolysates-treated groups.
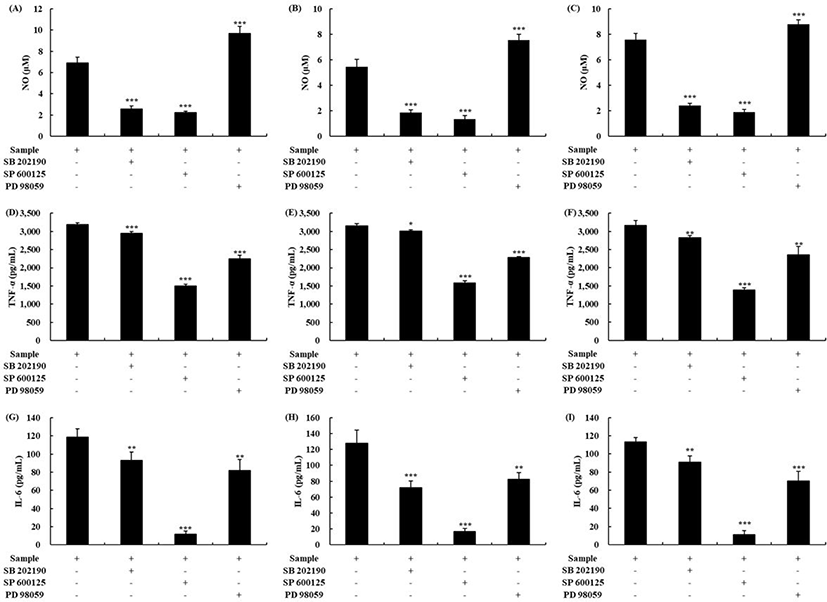
MAPK are reported to activate macrophages and control the production of inflammatory mediators such as NO and inflammatory cytokines (IL-1β, IL-6, IL-10, IFN-γ, and TNF-α) [1,13]. When p38, ERK, and JNK were inhibited by specific inhibitors, the production of inflammatory mediators decreased in macrophages, indicating that the OTF hydrolysates activated macrophages by the MAPK. When lysozyme boosted the production of inflammatory mediators, it was suppressed by adding the MAPK inhibitors [2]. A study of polysaccharide from Ecklonia cava reported that the secretion of IL-2 by polysaccharides was inhibited by adding the NF-κB or JNK inhibitor, which was similar to our results [34].
In conclusion, we examined the immune-enhancing activity of OTF hydrolysates using various assays. We found that OTF hydrolysates promoted phagocytic activity. OTF hydrolysates increased the production or secretion of NO/iNOS, TNF-α, and IL-6 by activating macrophages. It was confirmed that macrophages activation by OTF hydrolysates is induced through the MAPK signaling pathway. The activated macrophages secreted a variety of cytotoxic proteins to help eliminate virally infected cells, cancer cells, and intracellular pathogens. These findings suggest that OTF hydrolysates could be used as a functional food ingredient with immune-enhancing activity in the food industry in the future.