INTRODUCTION
Antibiotics have been widely used as growth promoters in livestock to improve animal performance and profitability by improving feed efficiency and animal growth, as well as reducing the incidence of diseases [1]. In particular, nursery pigs are supplemented with antibiotics for disease prevention or to reduce morbidity and mortality [2]. On the other hand, extensive use of antibiotics promotes antibiotic resistance, which could have a negative impact on both animal and human health. Antibiotics used for animal growth are closely related to the class of antibiotics used in human medicine to treat foodborne infections, including penicillin, aminoglycosides, and tetracyclines [3]. The use of antimicrobials in food-producing animals leads to multidrug resistance in both animals and humans [3,4]. Therefore, several countries have banned or placed restrictions on antibiotic use in animal feed [4–7]. Thus, it is necessary to adopt alternative approaches for antibiotic use. During post-weaning, various changes take place in the swine due to stress, new diet, and other factors, which cause increased invasion and colonization of pathogenic bacteria, resulting in infection and diarrhea [8,9]. The immune system is not mature enough in piglets to fight invading pathogens; hence, the post-weaning period is a critical time for maintaining animal health and performance. The gut microbiome plays a major role in immune system development, maintaining nutrient metabolism, performance, disease defense, and health status of the host [10]. Intestinal microbiota might be a potential novel strategy to modulate the general immune system and gut health [11,12]. To modulate the gut microbiota to exert beneficial effects on the host, most researchers have employed prebiotics, probiotics, essential oils [13–15], dietary enzymes, natural herbs, and medicinal plants [16] or phytobiotics [17–19].
Turmeric, also known as the golden spice, is a popular medicinal herb derived from Curcuma longa Linnaeus rhizomes. Turmeric plays a vital role in traditional medicinal purposesas an antimicrobial and anti-carcinogenic agent [20,21]. It contains approximately 69.4% carbohydrates, 5.1% fat, 6.3% protein, 3.5% minerals, and 13.1% moisture [22,23]. The turmeric rhizome contains a major fraction of starch (47%–56% w/w) on a dry basis [24,25]. Isolated turmeric starch contains 48%–50% (w/w) amylose [25]. Indigestible carbohydrates, such as resistant starch and other carbohydrates, are fermented by microbes in the large intestine and produce short-chain fatty acids (SCFAs) and other products. The main bioactive compounds in turmeric are curcuminoids, which constitute 1%–6% of the dry weight of turmeric [26]. The three major curcuminoids are curcumin (80%), desmethoxycurcumin (18%), and bisdemethoxycurcumin (2%) [27,28]. The bioactive compounds of turmeric consist of volatile and non-volatile phytochemicals that are less toxic and have beneficial effects, including antioxidant, antibacterial, anti-inflammatory, antiviral, antifungal, anticarcinogenic, and hypo-cholesteric activities [29–31].
Turmeric has gained attention in recent years as a potential alternative to antibiotic growth promoters in livestock feed. The beneficial effects of dietary inclusion of turmeric on growth performance and digestibility have been reported [32,33]. However, limited studies are available on the influence of turmeric on the gut microbiota of pigs. Furthermore, to the best of our knowledge, there is no metagenomic study on the effects of turmeric supplemented diets on gut microbiota in pigs. Therefore, the objective of this study was to investigate the effect of dietary turmeric supplementation on growth performance, blood parameters, fecal score, fecal SCFAs, branched-chain fatty acids (BCFAs), gut microbiota, and histomorphology of the ileum in weaned piglets.
MATERIALS AND METHODS
A total of 48 newly weaned piglets (Duroc × [Landrace × Yorkshire]) with an initial average bodyweight (BW) of 7.35 ± 0.3 kg were used in 6-week feeding trail. All piglets were obtained from one farm and weaned at 28 days of age. Animal experiments were performed at the Animal Research Center at Chungnam National University, Daejeon, Korea. Animal care procedures and experimental protocols were approved by the Animal Care and Use Committee of Chungnam National University (Approval# CNU-00611). All piglets were randomly assigned to two dietary treatments: the group fed with basal diet only (control) and the basal diet supplemented with 1% (w/w) turmeric powder (turmeric). Turmeric powder was purchased from a local supermarket in Seoul, Korea. Each dietary treatment had four replicates per treatment, with six piglets per pen. In total, 48 male piglets, 24 piglets in the control group, and 24 piglets in the turmeric group were allotted. Diets in mash form were formulated to meet the requirements suggested by the NRC 2012 [34]. Nutrient composition of the diet and chemical composition of turmeric are shown in Tables 1 and 2, respectively. A general maintenance program was used for sows and piglets during lactation. The diets did not include any antibiotics to avoid antibacterial activity during the lactation and experimental periods. All experimental piglets were housed in an environmentally controlled, slatted-floor facility with a mechanical ventilation system. Each pen was equipped with a self-feeder and nipple water to allow ad libitum access to feed and water throughout the experimental period. The piglets were individually weighed at the start and at weeks 3 and 6 of the experimental period, and feed intake was recorded throughout the experiment to calculate average daily gain (ADG), average daily feed intake (ADFI), and the gain-to-feed ratio (G:F) was calculated using ADG and ADFI.
3) Provided per kilogram of diet: vitamin A, 12,000 IU; vitamin D3, 2,500 IU; vitamin E, 30 IU; vitamin K3, 3 mg; D-pantothenic acid, 15 mg; nicotinic acid, 40 mg; choline, 400 mg; and vitamin B12, 12 μg.
4) Fe, 90 mg from iron sulfate; Cu, 8.8 mg from copper sulfate; Zn, 100 mg from zinc oxide; Mn, 54 mg from manganese oxide; I, 0.35 mg from potassium iodide; Se, 0.30 mg from sodium selenite.
On the final day of the experiment, freshly voided fecal samples from one randomly selected piglet in each pen were collected by rectal stimulation for 16S sequencing and SCFA analysis. The number of samples was determined based on our previous pilot study (data not shown). All samples were stored at −80°C until analysis. Blood samples were collected aseptically through an external jugular vein puncture.
All blood samples were centrifuged at 1,200×g for 10 min at 4°C, and plasma and serum samples were sent to Neodin Vet Lab (Seoul, Korea) on the same day of sample collection for analysis of concentrations of total proteins, creatine, urea, glucose, total cholesterol, triglycerides, and gamma-glutamyl transferase. Plasma samples were analyzed for red blood cell (RBS), white blood cell (WBC), and platelet counts.
Volatile fatty acids (VFA) analysis was performed according to Cho et al. [35] with modifications using gas chromatography (GC) (6890 N, Agilent, Santa Clara, CA, USA), equipped with an HP-INNOWAX column and a flame ionization detector. Fresh fecal samples (1 g) were acidified with 1 mL of 25% phosphoric acid solution, 3 mL of distilled water, and 50 μL saturated mercury solution (Sigma-Aldrich, St. Louis, MO, USA). After 30 min, the samples were centrifuged at 3,000×g for 20 min, and 3 mL of the supernatant was collected. Then, the 3 mL of the supernatant was centrifuged at 13,800×g for 10 min and filtered through a 0.2 μm filter (Whatman, Uppsala, Sweden). The filtrates were mixed with an equal amount of methanol and then placed in 2.0 mL GC vials (Agilent) to measure the concentration of volatile fatty acids. The sample injection volume was 2 μL, with a split ratio of 10:1.
On the final day of the experimental period, a total of 8 piglets (4 piglets per group) were slaughtered, and the intestinal tract was removed. The distal ileum segments were collected and fixed with 4% paraformaldehyde in 0.01 M phosphate buffered saline. The ileum sections were stained with hematoxylin and eosin for microscopic examination to determine villus height (VH), crypt depth (CD), VH:CD, villus width, villus area, and number of goblet cells.
The diarrhea score of each piglet was recorded at weeks 1 to 6 of the trial. Diarrhea was assessed visually based on consistency of the feces, and fecal scores were determined using the following fecal scoring system: 1 hard, dry pellet; 2 firm, formed stool; 3 soft, moist stool that retains shape; 4 soft, unformed stool that assumes shape of container; 5 watery liquid that can be poured. The fecal score was assessed in a treatment-blinded manner by two trained individuals. Scores were recorded on a pen-basis observation of individual piglets and signs of stool consistency in the pen [36].
Total DNA was extracted from fecal samples using the PowerSoil® DNA Isolation Kit according to the manufacturer’s protocol. The quantification of DNA and DNA quality was measured using PicoGreen and Nanodrop (Thermo Scientific, Waltham, MA, USA). The primers used for 16S V3-V4 rRNA gene amplification are listed in Table 3.
Direction | Primer |
---|---|
Forward | 5’TCGTCGGCAGCGTCAGATGTGTATAAGAGACAGCCTACGGGNGGCWGCAG |
Reverse | 5’GTCTCGTGGGCTCGGAGATGTGTATAAGAGACAGGACTACHVGGGTATCTAATCC |
Input gDNA (12.5 ng) was amplified with 16S V3-V4 primers, and a subsequent limited‐cycle amplification step was performed to add multiplexing indices and Illumina sequencing adapters. Amplicons from PCR were pooled using PicoGreen and used as input for Illumina library preparation. The size of the libraries was verified using the LabChip GX HT DNA High Sensitivity Kit (PerkinElmer, Waltham, Ma, USA). Samples were sequenced using an Illumina MiSeq (Macrogen, Seoul, Korea).
Sequencing reads obtained from Illumina MiSeq were filtered and trimmed using CD-HIT-OUT software and rDNA Tools [37]. To perform taxonomic assignment, operative taxonomic units (OTUs) were selected based on a 97% threshold of sequence similarity using the QIIME-UCLUST program. The filtered reads were clustered and OTUs were generated using CD-HIT-DUP. The sequences that passed from the quality filters were analyzed using the QIIME pipeline, which included features to calculate diversity indices and phylogenetic diversity (PD) rarefaction curves. Alpha-diversity indices including OTUs, Shannon, Chao1, and Simpson index were measured for each sample, and beta-diversity of the two groups were illustrated using principal component analysis (PCA) and principal coordinate analysis (PCoA) based on weighted UniFrac distances. The Ribosomal Database Project (RDP) classifier was used for taxonomic assignment of the fecal microbiome of the two groups.
Data of hematological and biochemical analyses, VFA concentrations, histometric analysis, and microbial diversity indices were analyzed by Student’s t-test using the SPSS Statistics Version 23 software package (IBM, Armonk, NY, USA). Growth performance data were statistically analyzed using the GLM procedure of the Statistical Analysis System (SAS Institute, Cary, NC, USA). The Mann-Whitney U test was used to determine the statistical significance of the relative abundance of microbial communities in two groups at the phylum, class, genus, and species levels (SPSS version 23, IBM). Statistical significance was reported at p < 0.05, and trends were noted when 0.05 < p < 0.10.
RESULTS
In the current study, piglets supplemented with turmeric diet had increased final BW compared to piglets fed the control diet (Table 4). Furthermore, dietary supplementation with turmeric had significant effects on ADFI and tendency effects on G:F at week 3 without affecting ADG. At week 6, increased ADG (p = 0.026) and tendency effects on G:F (p = 0.09) did not affect ADFI. Overall, turmeric supplementation had increased (p < 0.04) ADG and tendency toward G:F (p = 0.078) without affecting total ADFI (p = 0.349). The diarrhea incidence scores are presented in Table 5. During the experimental period, none of the piglets suffered from diarrhea. Supplementation of turmeric with the basal diet improved the fecal score during week 6 (p = 0.009).
The hematological parameters for the turmeric and control groups are shown in Table 6. Dietary turmeric supplementation did not influence leukocyte, erythrocyte, and thrombocyte counts, mean corpuscular volume (MCV), mean corpuscular hemoglobin (MCH), and mean corpuscular hemoglobin concentration (MCHC). There were no significant differences between the two groups (p > 0.05). The effects of dietary turmeric on the biochemical variables are summarized in Table 7. Turmeric supplementation did not significantly affect the levels of total, LDL, and HDL cholesterol. Numerically, HDL-cholesterol was higher in the turmeric group, but not significantly (p = 0.776).
TP, total protein; ALB, albumin; T.Bil, total bilirubin; BUN, blood urea nitrogen; GTP, glutamyl transpeptidase; LDH, lactic acid dehydrogenase; Chol, cholesterol; TG, triglyceride; HDL, high density lipoprotein; LDL, low density lipoprotein; AST, aspartate amino transferase; ALT, alanine aminotransferase.
As shown in Table 8, dietary turmeric supplementation increased SCFA production (p < 0.05). Acetic, propionic, and butyric acids were predominant. The highest acetic acid levels were observed in the turmeric group, followed by propionic and butyric acids. The levels of isobutyric and isovaleric acids were not significantly different between the two groups; however, the concentrations showed an increasing tendency in tumeric group (p = 0.057).
Turmeric supplementation had no effect (p > 0.05) on VH and CD (Table 9). However, the VH:CD ratios were higher in the turmeric group than in the control group (p = 0.04). There was no difference in the surface area and width of villi in the turmeric group compared to the control group.
A total of 437,000 read bases were obtained from the sequencing of fecal samples from the control and turmeric groups. After filtering and removing low-quality sequences, an average of 39,289 and 41,318 reads were obtained for control and turmeric group samples, respectively. A total of 870 OTUs belonging to bacteria and archaea were identified at the 97% threshold level.
Alpha-diversity analyses, including Shannon, Simpson, and Chao1 indices were analyzed to explore the effect of dietary turmeric on the richness and evenness of gut microbiota. The α-diversity metrics are shown in Figs. 1A, B, and C. According to the data, all diversity indices were not significantly different (p > 0.05) between groups, while turmeric administration showed a decreasing tendency in the Shannon index (p = 0.055).
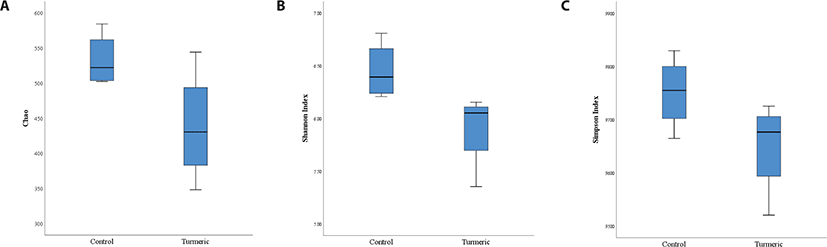
α-Diversity rarefaction curves based on observed OTUs, PD whole tree, and sequence for samples between the two groups indicated sufficient sequencing depth (Figs. 2A and B). PCoA based on weighted Unifrac distance showed two clusters containing each sample of both control and turmeric groups, except for one sample in each group (Fig. 3).
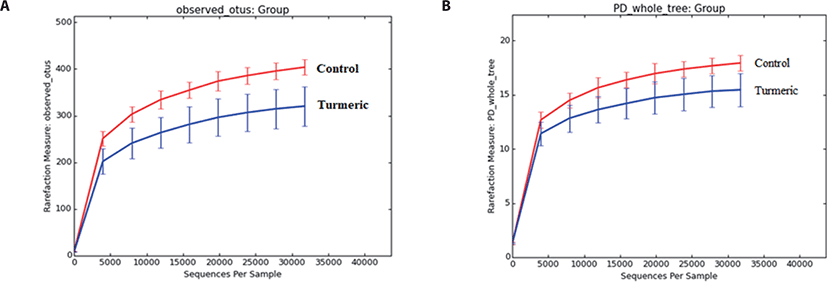
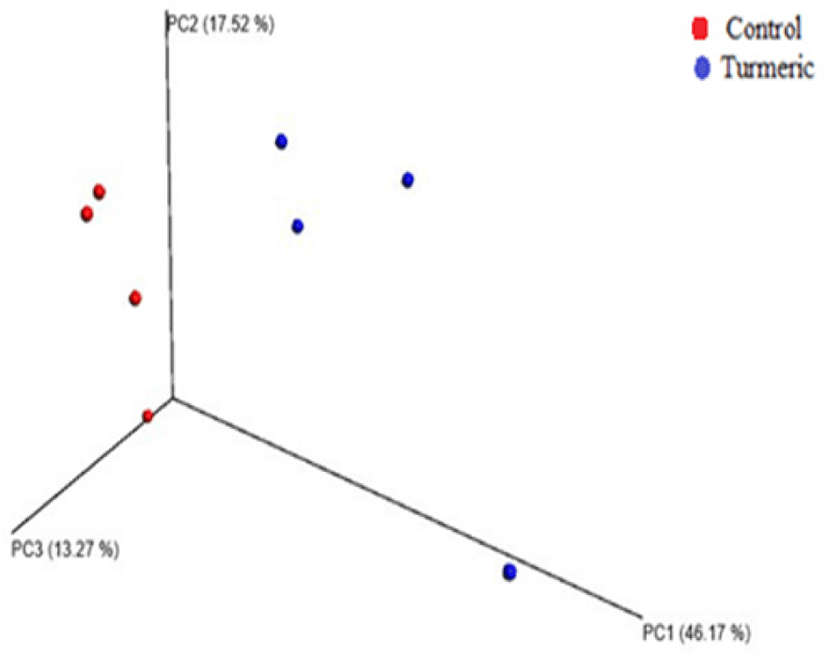
The effects of turmeric supplementation on fecal microbial composition were observed at different taxonomic levels. At the phylum level, 10 phyla were observed in each sample. Bacteroidetes and Firmicutes were the predominant phyla, accounting for 90% of the total relative abundance. The Firmicutes abundance ratio was similar in the control (51.8%) and turmeric (52.46%) groups (Fig. 4). Bacteroides abundance decreased in turmeric (27.62%) fed piglets than in control (39.61%) fed piglets (p = 0.021).
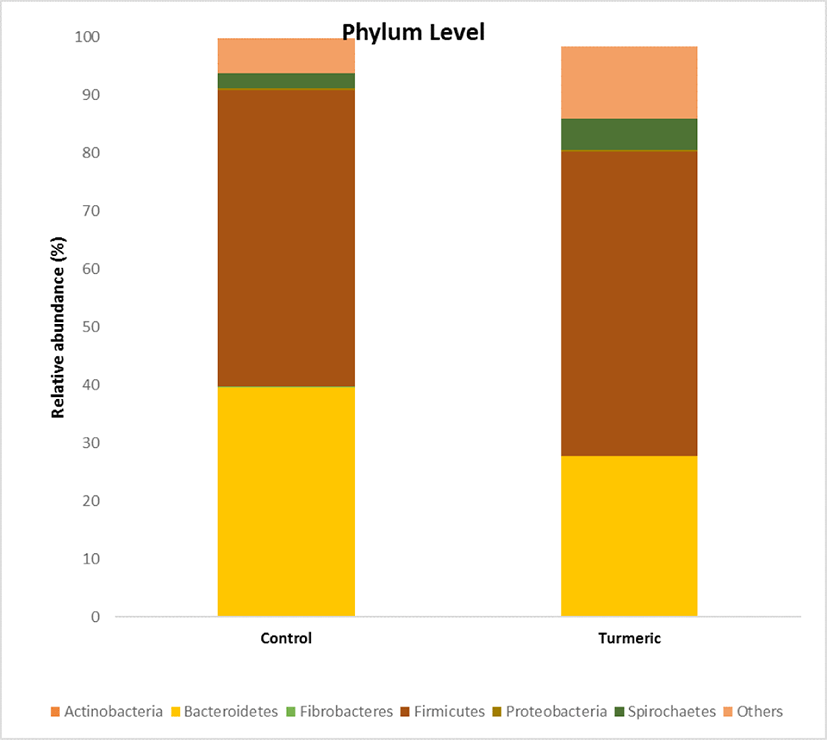
As shown in Fig. 5, Bacteroidia and Clostridia were the predominant classes in both the control and treatment groups. Twelve classes were identified. The relative abundance of Bacteroidia was significantly decreased in turmeric-fed piglets (p = 0.021). However, the remaining bacterial abundance was not affected by the turmeric diet.
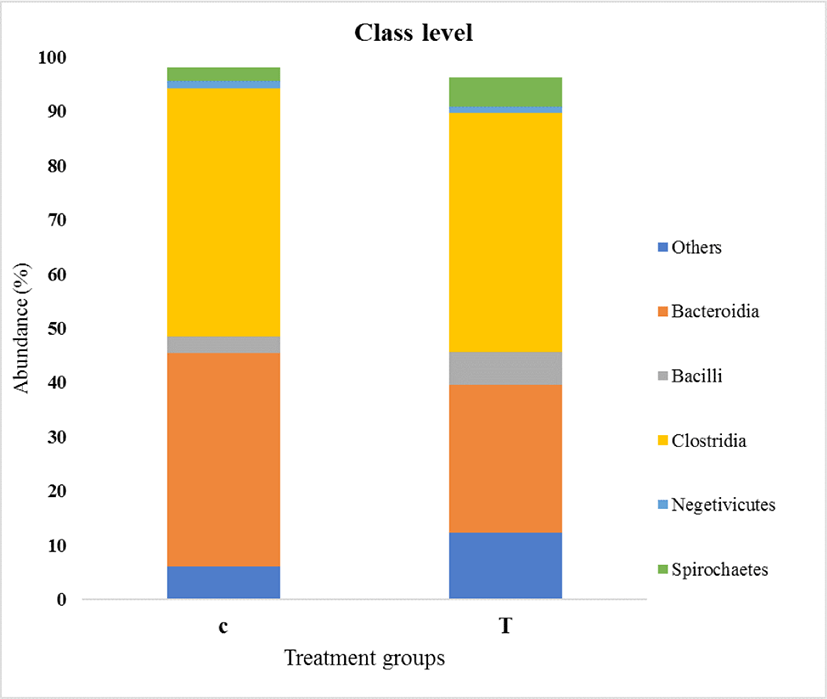
At the genus level, approximately 105 genera were found in fecal samples from both dietary groups. The 15 most abundant genera in the two groups are shown in Fig. 6. Among the abundant genera, eight belonged to Firmicutes, 6 from Bacteroidetes, and 1 from Spirochaetes. The unclassified bacteria at the genus level were higher in the turmeric group than in the control group (p = 0.043). The relative abundance of Prevotella genera was significantly lower in the turmeric group than in the control group (p = 0.021). The Lactobacillus genus showed an increasing trend in the turmeric group (p = 0.083). The remaining genera did not differ significantly between the two groups. At the species level, a total of nine species abundance ratios were statistically significant between the two groups, including Lactobacillus spp. (Table 10).
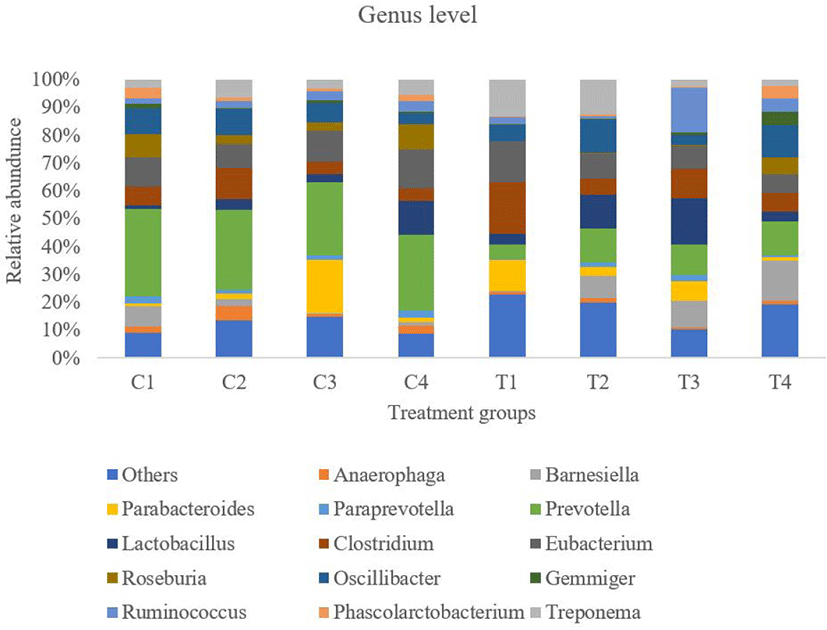
DISCUSSION
Turmeric root powder is commonly used for medicinal purposes and as a spice in traditional cooking. A couple of studies have investigated the effects of turmeric supplementation on the growth performance of piglets [32,33]. However, more studies are needed to explore the effects of turmeric on intestinal morphology and gut microbial communities. Gut microbiota provides not only fuel to colonocytes, but also helps in immune system development and maintenance of intestinal homeostasis [10]. In this study, we describe the impact of dietary turmeric on growth performance, gut morphology, and microbiota in a porcine model. Turmeric inclusion in piglet diets improved ADG and showed higher average final BW and lower feed intake than the control group. Similarly, a previous study indicated that diets containing turmeric powder at 2%, 4%, and 6% improved final live weight and feed conversion ratio compared with basal diet fed pigs [38]. Maneewan et al. [32] reported that the effects of low levels of dietary turmeric supplementation on nursery pigs at doses of 0.05%, 0.10%, and 0.20% did not influence ADFI, ADG, and feed efficiency. The beneficial effects of turmeric on growth performance might be due to the enhanced secretion of amylase, trypsin, chymotrypsin, and lipase enzymes [38]. Similarly, Singh et al. [39] reported that 1% dietary turmeric resulted in increased BW gain in broiler chickens, however, turmeric did not influence the feed efficiency in this study. Tubcharoen et al. [40] reported similar results in growing-finishing pigs. Furthermore, in this study, fecal scores were much higher in the control group. The incidence of diarrhea among piglets was reduced when the diet was supplemented with turmeric.
Hematological and biochemical variables were determined to determine the health and stress status of the animals. There were no significant differences in the values of RBC, WBC, MCV, MCHC, MCH, and platelets. However, all hematological parameters were within the normal range for swine [41]. This indicates that turmeric causes no variations in the hematology of piglets and that none of the animals experienced stress during the experimental period. The serum biochemical variables were not affected by turmeric. The main active compound, curcumin, in turmeric exhibits hypocholesterolemic activity. Curcumin showed beneficial effects in high-fat fed animals [42]. In the present study, turmeric did not influence cholesterol levels in piglets. These results contrast with those of a previous study that showed that curcumin supplementation decreased serum LDL cholesterol levels in weaned piglets [2]. The lipid-lowering effects of turmeric and curcuminoids are associated with the dose and solubility of curcumin. Considering the poor solubility and bioavailability of curcumin, Porn-anek et al. [43] developed a carrier-based turmeric oleoresin using a solid dispersion technique to enhance curcumin solubility. Pigs fed with the newly developed turmeric oleoresin had increased HDL cholesterol and lowered LDL cholesterol, total cholesterol, and triglycerides.
Intestinal metabolites such as SCFAs play a major role in the regulation of gut homeostasis [42]. SCFAs are the end products produced by bacterial fermentation of non-digestible carbohydrates in the colon [13,44–46]. Primary SCFAs are acetic, propionic, and butyric acids, with butyric acid being the main energy substrate for colonic epithelial cells [44,47]. In this study, turmeric diet-fed piglets produced higher levels of SCFAs. Acetic, propionic, and butyric acid concentrations were higher among all the SCFAs. Catabolism of turmeric polysaccharides provides energy for fermentative bacteria such as Lactobacillus spp. and Clostridium spp., which results in an increase in SCFA production [28]. Similarly, Han et al. [48] reported that in vitro fermentation of spent turmeric powder with pig fecal bacteria resulted in higher concentrations of acetate and propionate. Microbial abundance, particularly Lactobacillus abundance in the gut, is associated with gut SCFA production. The status of gut health can be determined by intestinal morphology, such as VH, CD, and the VH:CD ratio. Villi plays a major role in increasing nutrient absorption, especially in the small intestine [12,49]. In this study, turmeric-fed piglets showed a higher VH:CD ratio than the control group. Increased VH:CD ratios indicate improved nutrient absorption function [50]. This result is consistent with a previous study that piglets consuming dietary curcumin showed an improved VH:CD ratio [49].
Turmeric has been reported to be an antimicrobial and anti-inflammatory agent [21,22]. Avanço et al. [51] reported that α-turmerone, β-turmerone, and ar-turmerone components of turmeric showed antifungal, antimycotoxigenic, and antioxidant activities. Thus, turmeric could alter microbial communities in the intestine by inhibiting pathogenic bacteria. The taxonomic analysis in this study showed that Firmicutes and Bacteroidetes were the most abundant phyla in both groups of piglet gut microbiota, as reported in previous studies [52,53]. The abundance ratio of Bacteroidetes decreased in turmeric-fed piglets. However, Firmicutes phyla were not altered by turmeric. Bacteroidetes are gram-negative anaerobic bacteria normally present in the intestinal flora. Bacteroides are generally beneficial to the host through their metabolism of dietary polysaccharides; however, Bacteroidetes are involved in inflammatory pathology when the gut microbiota is in an imbalanced state [54,55]. Moreover, Zhao et al. [56] reported that fecal Bacteroides were negatively correlated with SCFAs and amino acids in mice. We also observed a decreased abundance of Bacteroidetes and increased concentrations of SCFAs in turmeric-fed piglets. Hence, low Bacteroides and higher SCFAs are predicted to promote the gut health of piglets in the post-weaning period. Turmeric had no impact on the overall microbial diversity and richness, except for the lower trend of the Shannon index. Similarly, Shen et al. [57] reported that oral administration of curcumin tended to decrease microbial diversity and richness with no significant differences. Despite no significant differences in microbial diversity, the abundance of specific bacteria, including Lactobacillus and Prevotella, were altered in turmeric fed piglets. At the genus level, Prevotella genera decreased in the turmeric group, similar to previous studies [57]. Little is known about the role of Prevotella in health promotion. Like other bacteria in normal microflora, Prevotella spp. act as opportunistic pathogens and have been associated with infections [58,59]. Turmeric increased the abundance of Lactobacillus spp. and Clostridium spp. and decreased the abundance of Prevotella spp. Similarly, Han et al. [48] reported that spent turmeric fermented with swine microbiota showed increased Lactobacillus populations compared to other groups. Kosti et al. [60] also observed higher Lactobacillus counts and lower E. coli counts in turmeric-fed hens than in the basal diet group. Some studies have suggested that the phenolic compound curcumin in turmeric root powder possesses alterations in gut microbial composition [28]. Moreover, curcumin has been found to improve the barrier function of the intestine by modulating intracellular signaling pathways [61]. The results obtained from this study revealed that dietary turmeric influences gut microbial fermentation and improves gut health by enhancing beneficial bacteria, SCFAs, and gut morphology. However, in the current study, we used whole turmeric root powder as a dietary supplement. Further studies that use turmeric extract or curcumin alone are needed to clarify the microbial alteration effects in the intestine of pigs.