INTRODUCTION
Global warming is the phenomenon of increasing average temperature on the planet, which is emerging as a serious problem. According to a prediction, heat stress (HS) caused by global warming will lead to financial losses in the U.S. pig industry [1]. Heat waves cause tropical climate expansion, and environmental changes in commercial farms will alter the nutritional metabolism of pigs [2].
Pigs are vulnerable to HS due to the absence of a functional sweat gland and a thick layer of subcutaneous adipose tissue, which interferes with radiant heat loss [3,4]. Moreover, the severity of the HS damage to growing and finishing pigs is greater than that of young pigs because of their different thermal neutral (TN) zones according to body size [5]. Heat-stressed pigs attempt to dissipate heat by circulating blood flow from their body core to the periphery [5]. In this process, a decrease in the blood flow in the gastrointestinal tract leads to hypoxia, which causes a decrease in intestinal integrity and an increase in endotoxin permeability [6,7]. In addition, voluntary feed intake of heat-stressed pigs was also decreased to promote body heat loss by reducing heat production [8]. The shortage of nourishment induced by decreased feed intake and alterations in the blood circulatory system leads to shifts in metabolism and metabolite levels [2,9,10].
Several studies have shown the possibility of using metabolites as a biomarker to identify heat-stressed pigs using biological samples such as the blood and saliva of growing and finishing pigs [10–12]. The saliva sample is a more ideal material as a noninvasive and stress-free diagnostic tool in terms of the advantage of its collection as well as animal welfare [13,14]. However, more information about the effects of HS on salivary metabolites in pigs of a different gender is needed to find the biomarkers for developing the diagnostic tool for HS. Hence, the objectives of this study were to better understand sex-specific HS-mediated metabolic changes and to investigate metabolites from saliva samples that are related to HS, to evaluate them as potential biomarkers.
MATERIALS AND METHODS
The present study was conducted following the ethical guidelines laid down by the Institutional Animal Care and Use Committee of the National Institute of Animal Science (No. NIAS-2017-245). A total of 6 growing pigs (Duroc; 3 boars and 3 gilts) of age 3 months, with an average body weight of 56 ± 2.62 kg were used in this study. Animals were fed a diet based on corn and soybean meal, and the diet was formulated to meet or exceed the nutritional requirements suggested by the Korean feeding standard for swine [15] (Table 1). The pigs were given free access to water and 2 kg of feed twice daily (09:00 and 16:00). Feed and water intake were measured by a water meter (Daesung, Seoul, Korea) daily during the experimental period and water loss was also collected to calculate daily feed and water intake.
1) The values supplied per kilogram of premix feed concentrations: vit A 5,000,000 IU; vit D3 1,000,000 IU; vit E 1,000 mg; vit B1 150 mg; vit B2 300 mg; vit B12 1,500 mg; niacin amide 1,500 mg; DL-calcium pantothenate 1,000 mg; folic acid 200 mg; vit H 10 mg; choline chloride 2,000 mg; Mn 3,800 mg; Zn 1,500 mg; Fe 4,000 mg; Cu 500 mg; I 250 mg; Co 100 mg; Mg 200 mg.
The experiment was carried out in an environmental chamber equipped with controls for temperature and humidity and animals were kept in individual metabolism crates. The experimental temperature was determined by the temperature-humidity index (THI) categories used as an indicator of HS [16]. The classifications of the index for HS are as follows: Normal (THI < 23.33), Alert (23.33 ≤ THI < 26.11), Danger (26.11 ≤ THI < 28.88), and Emergency (THI ≥ 28.88). The THI was calculated based on the formula using temperature (T) and relative humidity (RH) [17]:
The pigs were kept at 25°C and 60% RH (Normal, THI = 22.69) for 5 days during the adaptation period as a control group (TN), and saliva was collected at the end of the adaptation period. After the adaptation period, the pigs were kept at 30°C and 60% RH (Danger, THI = 26.59) for 24 h to demonstrate the heat-stressed pig group (HS30), and at the end, saliva was collected for the metabolomics analysis. After the first heat treatment, the pigs were allowed to recover at 25°C and 60% RH for 5 days. After this break, the pigs were kept at 33°C and 60% RH (Emergency, THI = 28.93) for 24 h to demonstrate the higher heat-stressed pig group (HS33). Similarly, the saliva was collected for metabolomic analysis at the end of the second heat treatment. The collection of saliva samples was performed using a cotton swab fixed to the end of the rod and pushed it into the pig’s mouth carefully. Thereafter, the swab was taken out and placed into a tube (Sarstedt AG & Co., Numbrecht, Germany) to collect the saliva samples after centrifugation (1,660×g at 4°C for 15 min).
The saliva samples were used for 1H-nuclear magnetic resonance (NMR) spectroscopy analysis; 150 μL of saliva sample was mixed with 5 μL of deuterium oxide (D2O) containing 20 mM of sodium-3-trimethylsilyl propionate-2,2,3,3-d4 (TSP; Sigma Aldrich, St. Louis, MO, USA) and placed in 4-mm NMR nanotubes. NMR spectra for saliva samples were obtained by high-resolution magic angle spinning (HR-MAS) NMR using an Agilent NMR spectrometer (Agilent Technologies, Palo Alto, CA, USA) with a 4 mm gHX NanoProbe. The spinning rate was 2,050 Hz. A Carr-Purcell-Meiboom-Gill pulse sequence was used to remove signals generated by water and macromolecules in the saliva samples. The1H-NMR spectra were measured with an acquisition time of 1.704 s, 1 s of relaxation delay, and 10 min and 20 s of total acquisition time. Chenomx NMR suite 7.1 (Chenoms, Edmonton, AB, Canada) software was used for the assignment of spectra and quantification of metabolites. The TSP-d4 peak (0.0 ppm) was used for calibrating the chemical shifts as a reference.
Multivariate statistical analysis was performed to calculate the amount of metabolites in the samples. The spectra were binned into 0.001 ppm binning size and the binned spectra were normalized to the total aliphatic spectral area and aligned with the icoshift algorithm of MATLAB R2013b (Mathworks, Natick, MA, USA). The data were imported into SIMCA (SIMCA version 14, Umetrics, Umea, Sweden) software for additional analysis. Principal component analysis (PCA) and orthogonal partial least square discriminant analysis (OPLS-DA) were performed to visualize differences between experimental groups.
Variable importance in the projection (VIP) was obtained from the OPLS-DA model, and VIP values > 1 were considered relevant for differences between groups. Pearson’s correlation coefficients were calculated between the metabolites (VIP values > 1). Analyses of metabolites were performed using Microsoft Excel. The statistical significance (p < 0.05) of the apparent difference in metabolite concentrations was evaluated by one-way ANOVA (Prism 5.01, San Diego, CA, USA).
RESULTS
The distribution of all the saliva samples of boars and gilts was represented by the PCA score plots (Fig. 1). The separation from the TN to the HS33 group in boars was obvious (Fig. 1A). However, the PCA results did not show a distinct separation between TN and HS30 (Fig. 1A). Additionally, the results of the PCA exhibited distinct classification between the TN and HS33 groups in gilts (Fig. 1B).
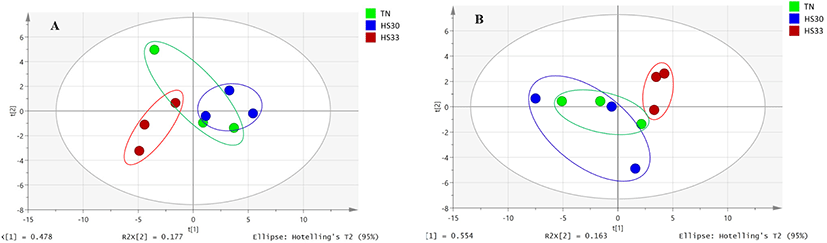
The OPLS-DA plots of the saliva samples of boars and gilts showed a clear separation between the TN and HS groups (Fig. 2). The parameters of the TN, HS30, and HS33 models for boar saliva samples (R2X (cum) = 0.872, R2Y (cum) = 0.48, and Q2 (cum) = 0.273) indicated that samples fit the established discriminant mathematic model (Fig. 2A). Similarly, the parameters of the TN, HS30, and HS33 models for gilts saliva samples (R2X (cum) = 0.808, R2Y (cum)= 0.889, and Q2 (cum) = 0.643) displayed a distinct separation among the three groups (Fig. 2B). The OPLS-DA models indicate that HS significantly affects the salivary metabolic profiles of growing pigs.
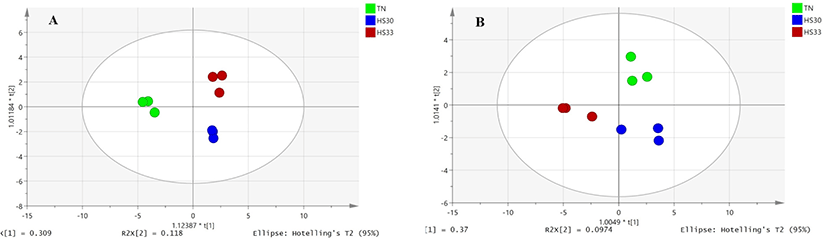
A total of sixteen and nine metabolites from the saliva samples of boars and gilts were considered relevant for group discrimination (VIP values > 1) and were identified in the TN and HS groups (Fig. 3). Among the sixteen metabolites with VIP values > 1 from the saliva samples of boars between the TN and HS groups, the pigs reared under HS conditions showed lower (p < 0.05) concentrations of glutamate, leucine, phosphocholine, and creatine than the TN group (Fig. 4). The concentrations of alanine, lactate, and glucose tended to decrease (p < 0.10), and that of formate tended to increase (p < 0.10) in the HS group compared to the TN group (Fig. 4). In contrast, among the nine metabolites with VIP values of > 1 from the saliva samples of gilts between the TN and HS groups, the concentrations of four metabolites (glutamate, leucine, dimethylamine, and creatine phosphate) were higher (p < 0.05) in the HS groups than in the TN group (Fig. 5). There was a tendency (p < 0.10) towards an increase in the concentrations of acetone, valine, proline, and succinate between the TN and HS groups (Fig. 5).
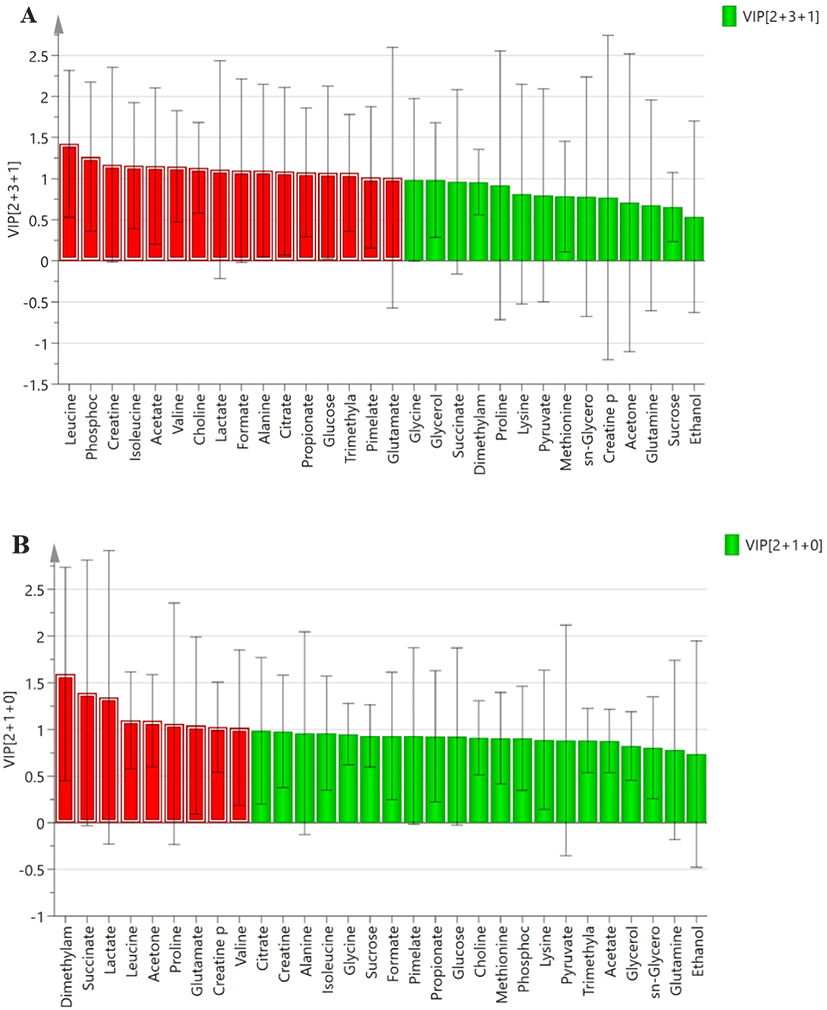
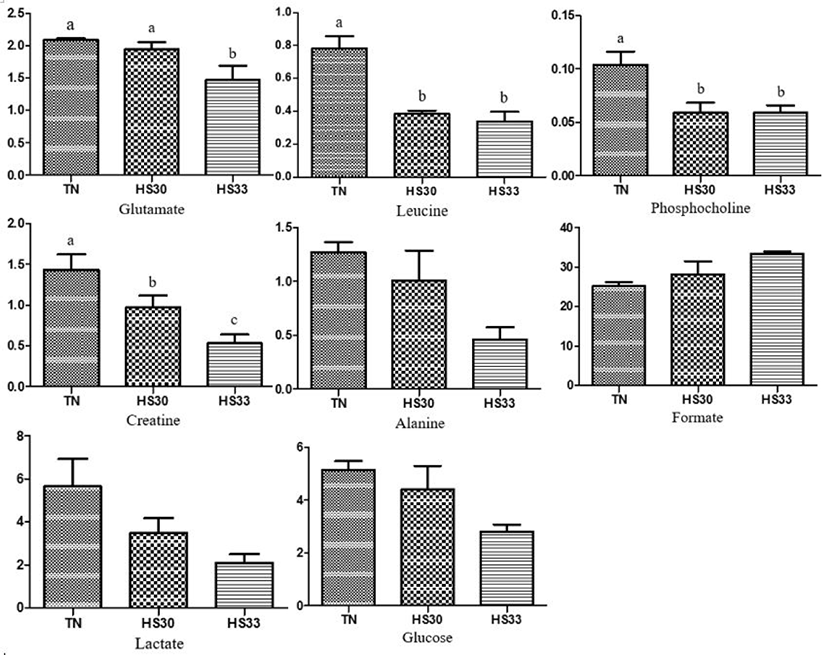
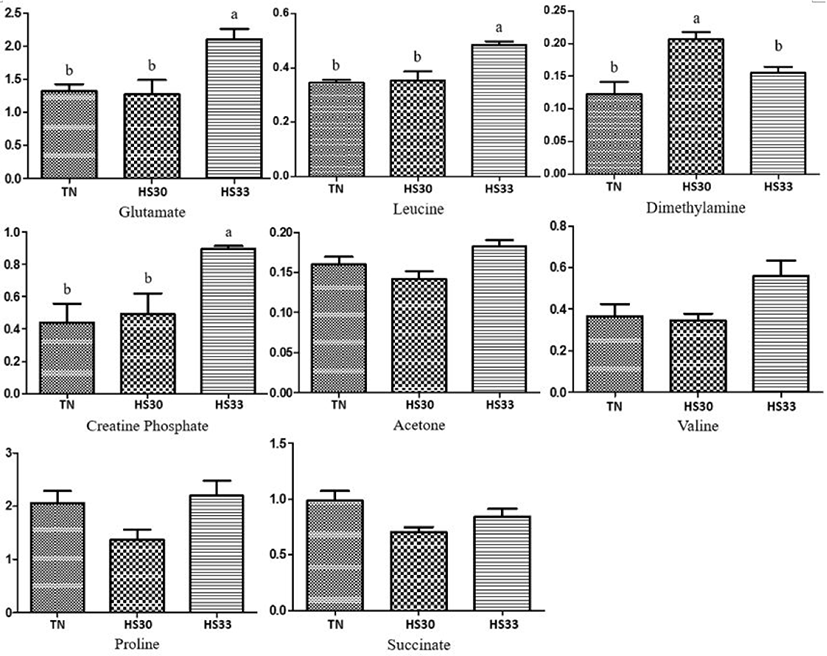
Tables 2 and 3 indicate the correlation coefficients between the metabolites in the saliva samples of boars and gilts, respectively. The values range from 1.00 (perfect positive correlation) to −1.00 (perfect negative correlation). In the case of boars, acetate concentration was negatively correlated with propionate (p < 0.05). Alanine concentration was negatively correlated with formate (p < 0.05) and positively correlated with glucose and pimelate (p < 0.01, p < 0.05). Choline concentration was positively correlated with lactate and propionate (p < 0.05,p < 0.05). There was a positive correlation between citrate and isoleucine (p < 0.05). Glucose and pimelate levels were inversely correlated with formate (p < 0.05 and p < 0.01, respectively). Both glutamate and pimelate were positively correlated with glucose (p < 0.05), and valine concentration was positively correlated with leucine and phosphocholine (p < 0.05). In the case of gilts, there was a positive correlation between creatine phosphate and leucine, glutamate and valine (p < 0.05). Dimethylamine concentration was negatively correlated with lactate levels (p < 0.01).
DISCUSSION
In the present study, NMR spectroscopy was used to quantify metabolites from porcine saliva samples. NMR has the advantage of being able to precisely identify specific liquid samples such as serum and urine [14]. It has been reported that the NMR analysis of saliva and urine from gilts could be used for the identification of puberty using potential biomarkers [18]. However, metabolic materials from the urine are primarily affected by food and the environment, resulting in heterogeneity compared with salivary metabolites, which are more homogenous [19]. Therefore, the use of salivary metabolites as a biomarker instead of any other biofluid (e.g., plasma, serum, and urine) can be a suitable method to manage heat-stressed pigs. The management of environmental conditions for pigs is important for better growth performance. In particular, optimal ambient temperature is necessary for the maintenance of normal body conditions, and the ideal TN zone for growing pigs is below 25°C [20]. In the present study, growing pigs in the TN group were raised at 25°C and those in the HS33 group resulted in a decrease in daily feed intake and a tendency to increase in daily water intake (our published data) [21]. Reduced feed intake and loss of appetite minimize metabolic heat production. Moreover, reduction in body weight occurs in acute heat-stressed pigs 24 h after heat treatment; they undergo catabolic metabolism and dehydration, resulting in reduced feed efficiency, fat deposition, and protein degradation [2,6,22].
Acute HS primarily leads to a temporary nutrient restriction to minimize metabolic heat production, an alteration in intestinal function, and an increase in intestinal permeability caused by vasoconstriction in the gastrointestinal tract [7,23]. Moreover, these symptoms cause an increase in glucose absorption by upregulation of glucose-related transporters to preferentially protect the intestinal epithelial cells (IECs) [24]. The absorbed glucose is used for the generation of adenosine triphosphate (ATP) as an energy source for IEC protection [25]. In the literature, continuous feed and energy depletion by HS, resulting in negative energy balance (NEB) due to insufficient fuel for energy supply [12]. In the NEB state, protein catabolism is increased, meaning that an alternative energy fuel is needed [26]. Furthermore, Lu et al. [22] reported that chronic HS decreased the proportion of chicken breast meat and increased leg meat for energy production. It seems that breast meat is affected owing to its relatively higher protein rate than leg meat.
It has been shown that differently gendered pigs have a different mechanism under acute HS due to the conflicting results of several metabolites, and a schematic overview of some changed metabolic pathways is shown (Fig. 6A). It has also been reported that the gender of pigs might affect the HS response [27,28]. In the present study, the glutamate and leucine levels in the saliva samples from the male pigs decreased and the concentrations of glucose and alanine tended to decrease by HS. It may be speculated that the NEB state occurred. However, the glutamate and leucine levels in the saliva samples from the female pigs increased and the concentration of valine tended to be higher in HS33 than TN. Even though the TN zone depends on body size, the pigs used in our study had similar body weights [5]. Therefore, the contrasting result of the metabolites associated with the tricarboxylic acid cycle could be ascribed to a gender effect because uncastrated pigs (boar) were used in this study. It was reported that an increase in cortisol and protein catabolism for glucose production are consequence of nutrient depletion by releasing glucocorticoids from the adrenal cortex [29]. This hypothalamic-pituitary-adrenal (HPA) axis is activated under stressful conditions; however, female sex hormones could attenuate the stress response and slow down the cortisol feedback by reducing the reactivity of the HPA axis [30]. Moreover, different body compositions (lean meat and subcutaneous fat) according to sex may also affect the heat response. Boars generally have a thicker subcutaneous fat layer than gilts [31–33]. However, the thick subcutaneous fat layer, which has an insulation effect, disturbs radiant heat loss for heat dissipation [4,34]. These sex-specific differences in the hormonal mechanism and body composition could alter the HS response of male and female pigs. In other words, it appears that the NEB triggered by the HS was less severe in the female pigs compared to the male pigs, which would lead to contrasting results for the concentrations of glutamate and leucine.
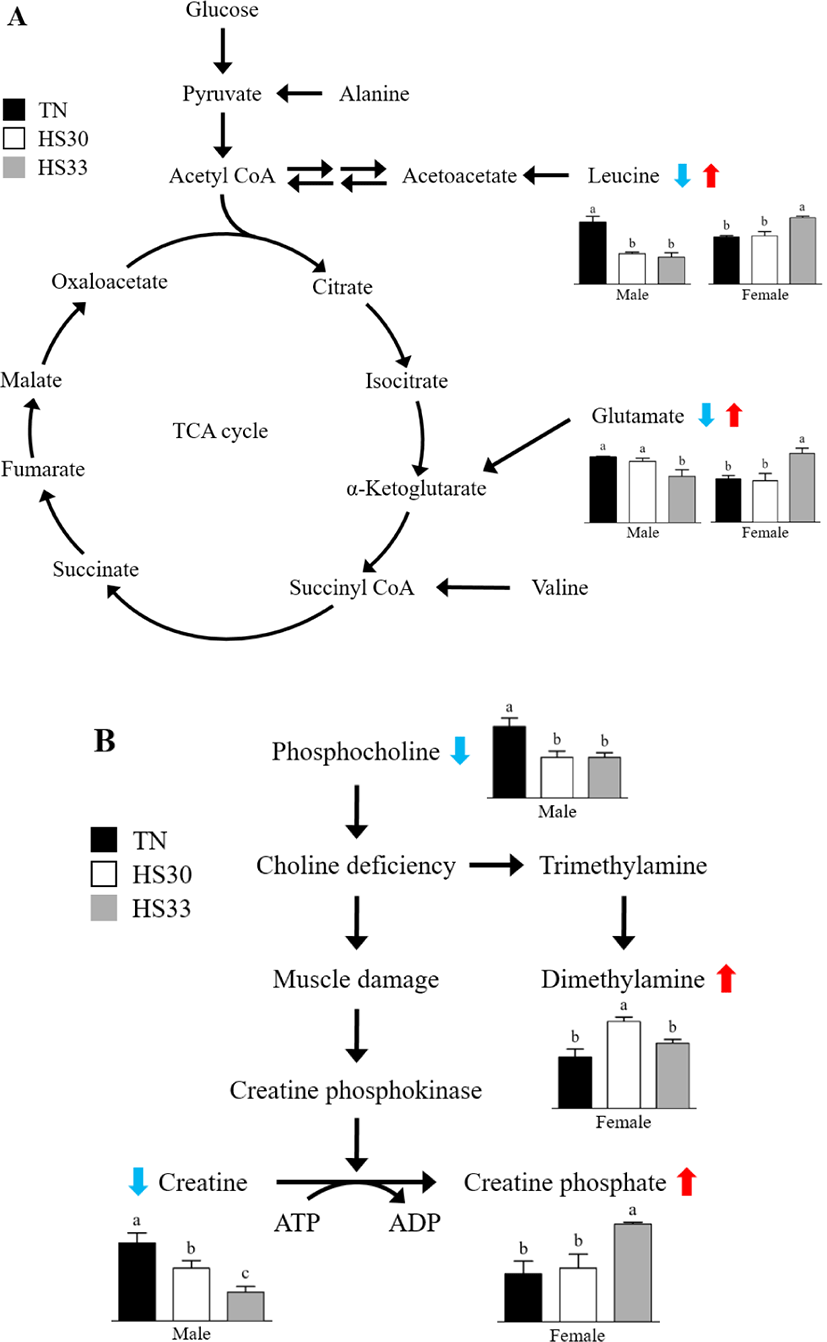
Acute HS also changed the metabolites related to protein metabolism in all gender; besides, a muscular reaction by the HS was an inevitable event for male and female pigs. The decreased feed intake and increased energy expenditure cause weight loss by the NEB, resulting in muscle damage [35,36]. A schematic representation of some changed metabolic pathways is shown in Fig. 6B. In the present study, creatine was decreased in male pigs, whereas creatine phosphate was increased in female pigs. Even though the results of these metabolites were found in different sexes, it appears that creatine, which can attenuate the damage and enhance muscle gain, was converted to creatine phosphate to protect from protein degradation in muscle using ATP and creatine phosphokinase [37,38]. However, chronic HS increased the creatine level by mobilization of creatine phosphate in the muscle tissue for energy supply by nutrient depletion [39,40]. Additionally, elevated levels of creatine phosphokinase were induced by a decrease in phosphocholine level, choline deficiency, and muscle damage [37,41]. Moreover, choline deficiency may have contributed to the difference in dimethylamine (DMA), which is formed from trimethylamine (TMA) and choline in female pigs [42,43]. It has been reported that dietary choline increased TMA and DMA in rats, and a choline-free diet also increased the excretion of TMA and DMA due to persistent excretion of methylamine in some rat tissues [42–44]. However, further research using the pig model is needed to elucidate the observed significant difference in the DMA in female pigs by the HS.
Concentrations of volatile fatty acids (VFAs) such as acetate, propionate, and butyrate are related to reduced feed intake by HS. Especially, Bedford et al. [45] reported that the absorption and production of the VFAs are associated with controlling both satiety and hunger. In the present study, it can be speculated that reduced feed intake by HS decreased production and increased absorption of both acetate and propionate. Because it was previously reported that infusion of propionate decreased feed intake by affecting satiety [46]. There was also a result of interconversion between acetate and propionate by the HS [45]. However, in the result of correlations of identified metabolites in pigs, there was a negative correlation between the concentrations of acetate and propionate. This result might be due to the conversion of propionate to acetate by decreased feed intake under HS condition was stronger than that of acetate to propionate [45].
In conclusion, HS induced alterations in several metabolites, and the response to HS showed different metabolic changes caused by the gender effect. The reason for this is not clear; however, sex-specific characteristics, such as different-sex hormonal mechanisms and body composition, may lead to different results of the metabolites in this study. Besides, in an effort to protect muscle damage from acute HS, alteration in protein-related metabolites is required for regulatory adaptation to acute HS. However, further research is necessary to elucidate the effect of sex differences on the response to HS. It is anticipated that this study will facilitate the development of a diagnostic tool to manage heat-stressed pigs.