INTRODUCTION
Calves are supplied with nutrients from milk and grain starter, and this helps in the development of the skeleton, muscles and the accumulation fats during their growth stage. In particular, the number of adipocytes increases during growth [1], and the rumen and papilla which are essential for digestion and absorption of nutrients are also remarkably developed at this stage [2]. Therefore, understanding the physiological mechanism of calf growth and researching related fields is important for improving the ability and quality of Hanwoo calves. Unfortunately, in the case of Hanwoo cattle, most studies have concentrated on improving intramuscular fat with a focus on the finishing phase, while only a few studies have focused on calves.
As the genome information sequence of livestock is completed and molecular genetics and genomic technology is rapidly developing, the interaction of genes with nutrients is being revealed [3]. The formation of muscle fibers and adipocytes is directly and indirectly influenced by the expression and regulation of various genes [1]. Major gene groups are used as markers to assess an individual’s ability or to improve traits. Gene expression is influenced by genetic and environmental factors, and is differentially expressed in the growth period [4]. Understanding the expression patterns of myogenic and adipogenic genes in the growing stages of calves and distinguishing the main genes is necessary for improving the productivity of Hanwoo.
Therefore, this study was conducted to provide as basic data for improves productivity, such as improving the quality of beef and shortening the fattening period through compare of mRNA expression levels of myogenic-adipogenic makers in the skeletal muscle, adipocytes formation, body weight, rumen weight, and papillary length on Hanwoo calves at newborn and 6 months of age.
MATERIALS AND METHODS
The management of the Hanwoo calves used for the study and all experimental procedures were examined and permitted by the Institutional Animal Care and Use Committee of the National Institute of Animal Science (No. 2017-119).
Three newborn calves (NC, average body weight: 24.7 ± 0.6 kg), and three 5 to 6 months old calves (SC, average body weight : 105.7 ± 3.2 kg) were used for the study. SC was weaned at 3 months of age, and concentrate and timothy were fed until slaughter. Chemical compositions of the concentrate and timothy used in this study are shown in Table 1.
Item | Concentrate (%) | Timothy (%) |
---|---|---|
Dry matter | 88.76 | 91.38 |
Crude protein | 17.39 | 6.58 |
Ether extract | 3.26 | 2.92 |
Crude ash | 6.10 | 4.55 |
Crude fiber | 7.86 | 30.47 |
Calcium | 0.89 | 0.22 |
Phosphorous | 0.64 | 0.22 |
After calves slaughter, samples were collected from both sides after removal of the skin and subcutaneous fat at the site of the longissimus dorsi (LD) and semimembranosus (SM). At the same time, the rumen was separated from the calves, and the rumen contents were removed, and followed by weighing and sample collection. Tissue samples (LD, SM and rumen) were each placed in a sterile tube and were immediately frozen in liquid nitrogen, and it was stored at −80°C until the RNA isolation. Another portion of the samples were fixed in formalin (10%) for histological analysis.
Samples from the LD and SM were transferred into 2 mL screw tubes, and 1 mL of TRIzol (Ambion, Carlsbad, CA, USA) and 2 beads were added. The samples were then homogenized for 2 min using a homogenizer (TissueLyser 2, Qiagen, Hilden, Germany), and later transferred into new microtubes. 100 μL of chloroform was subsequently poured into the microtubes and mixed using vortex. Centrifugation was performed at 16,996×g for 15 min using a centrifuge (MICRO 17TR, Hanil, Namyangju, Korea) and the supernatants transferred into new microtubes. After transferring the supernatant into new micro tubes, equal amount of isopropyl alcohol was added, and centrifugation was performed again for 10 min at 16,996×g and the supernatants were removed. Thereafter, 1 mL of 75% ethanol was added and centrifuged at 10,623×g for 5 min, the resulting supernatants were removed, and the samples were dried. RNA of the dried samples was dissolved by adding diethyl pyrocarbonate (DEPC) - treated water. cDNA was synthesized using cDNA synthesis kits (Rever Tra Ace® qPCR RT Master Mix with gDNA Remover, TOYOBO, Osaka, Japan) after measuring the concentration using 2 μL of DEPC containing RNA.
Real-time PCR analysis was performed using 7500 Fast Real-Times PCR system (Applied Biosystems, Foster City, CA, USA), and the myogenic markers used were myogenin (MyoG), myosin heavy chain 1 (MYH 1) and myosin heavy chain 2A (MYH 2A), while adipogenic markers were CCAAT/enhancer binding protein β (C/EBPβ), peroxisome proliferator activated receptor γ (PPARγ) and stearoyl-CoA desaturase (SCD). The primer sets used are shown in Table 2. 5.5 μL of distilled water and 2 μL of cDNA were injected on the plate, 1 μL of forward and reverse primers and 0.5 μL of probe were added, respectively. Finally, after adding 10 μL of Master Mix, the analysis was performed. The reaction conditions were as follows: 2 min at 50°C, 10 min at 95°C on the holding stage, and 15 s at 95°C, followed by reaction at 60°C for 1 min on the cycling stage. With this process as one cycle, a total of 40 cycles were performed.
Tissue samples were rinsing and immersed in 10%, 20%, and 30% sucrose solutions. The immersed tissues were embedded in an OCT compound and rapidly frozen. After freezing, each sample was sliced to a thickness of about 10 µm using a freeze slicer, and the sliced tissues were attached to silane coated slide glasses.
Tissue slides was washed with phosphate buffer saline (PBS). For the permeabilization, PBS-containing either 0.1% Triton X-100 (Sigma, Sant Louise, MO, USA) solution was added to the slides, and incubated at room temperature for 30 min. After incubation at room temperature for 30 min, 10% Albumin from bovine serum (BSA, Sigma, Sant Louise, MO, USA) + 22.52 mg/mL glycine in PBS + 0.1% Tween 20 (PBST) blocking solution was added. After removing the blocking solution, PBST solution of myosin light chain 2 (MLC2) primary antibody (Abcam, Cambridge, UK) diluted to 1/2,000 was subsequently added, and incubated for 1 h in a dark environment at room temperature, and washed with high salt PBS. The diluted (1/200) secondary antibody solution (Abcam, Cambridge, UK) was incubated in the dark at room temperature, and then wash with high salt PBS. 400 μL of body fit solution was added and incubated for 1 h in a dark environment at room temperature. Then wash with high salt PBS. Hoechst was diluted to 1/1,000, dispensed, incubated for 1 min in a dark environment, and washed with PBS. Finally, glycerin was added and the slides were covered with cover glass, and were observed with an optical microscope (ECLIPSE Ti-U, Nikon, Tokyo, Japan)
A generalized linear model (GLM) was used for the analysis and mean comparison using SAS 9.4 version, and the fixed effect (birth, 6 months of age) was assessed for significance at 5% confidence level by t-test.
Where, Yij = individual observations, µ = overall mean, monthi = age of calves (newborn and 6 months of age), and eij = random error.
RESULTS
The results of body weight, rumen weight and papilla on Hanwoo calves at newborn and 6 months of age are shown in Table 3 and Fig. 1. Body weight and rumen weight were significantly increased in SC compared to NC (p < 0.01), and the growth rate of rumen was higher than that of body weight. The ratio of rumen to body weight was approximaely 2.5-fold higher in SC than NC (p < 0.01), and papilla length was about 10-fold longer in SC than NC.
Item | NC | SC | SEM | p-value |
---|---|---|---|---|
Body weight (kg) | 24.7b | 105.7a | 1.09 | 0.01 |
Rumen weight (kg) | 0.51b | 5.52a | 0.12 | 0.01 |
R/B rate (%) | 2.05b | 5.22a | 0.12 | 0.01 |
Papilla length (mm) | 0.33b | 3.43a | 0.06 | 0.01 |
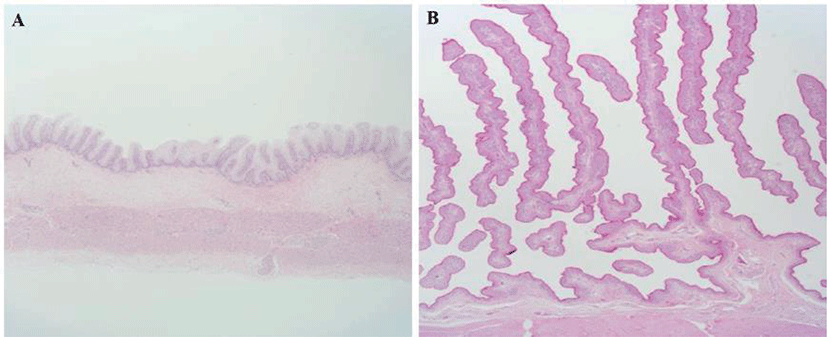
The results of immunofluorescence staining on skeletal muscle in Hanwoo calves at newborn and 6 months of age are shown in Fig. 2. Adipocytes were noticed in the LD and SM tissues regardless of the calves growth stage, and were mainly formed around blood vessels. In addition, adipocytes were more easily visualized in SC than in NC, and the area ratio of adipocytes in LD and SM tissue also showed higher results in SC than NC.
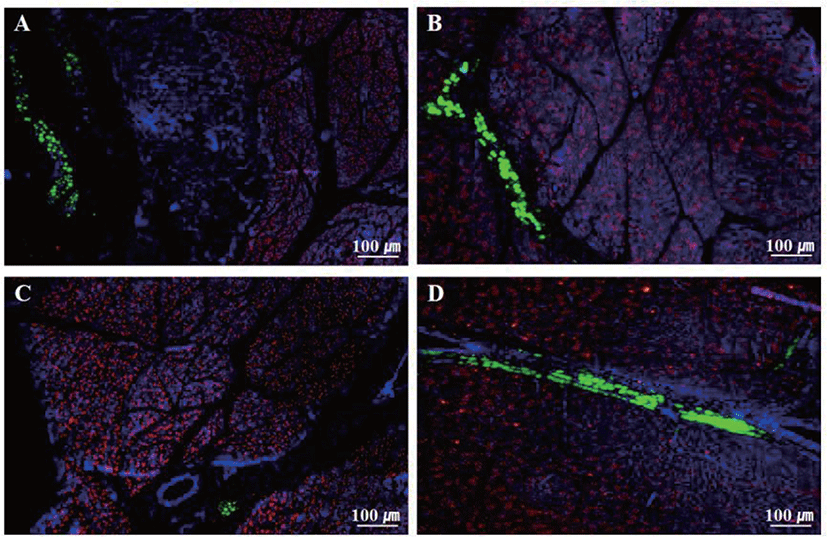
mRNA expression of myogenic-adipogenic makers on skeletal muscle in Hanwoo calves at newborn and 6 months of age are shown in Table 4. mRNA expression of myogenin (p < 0.01), MYH 1 (p < 0.02) and MYH 2A (p < 0.01) in LD was found to increase with calves growth. Also at the SM, mRNA expression was higher in SC compared to NC (p < 0.01). In addition, it was discovered that both NC and SC had higher levels of mRNA expression in the SM than in the LD.
In LD tissues, the mRNA expression of SCD (p < 0.04) and PPARγ (p < 0.03) was significantly higher in SC than NC. However the mRNA expression of C/EBPβ was slightly increased. In SM tissues, mRNA expression levels of SCD (p < 0.02) and C/EBPβ (p < 0.01) were higher in SC than NC, and mRNA expression levels of PPARγ also increased, but there was no significant difference.
DISCUSSION
The rumen is the largest digestive organ in ruminants, it is responsible for several physiologically important functions, including digestion, absorption, transport, and metabolism of short-chain fatty acids [5,6]. The rumen of the newborn calf occupies approximately 20% of the total stomach volume, the rumen development is completed at 14 to 16 weeks of age, and the composition of the rumen microbial community is established during the early growth periods [7]. Lyford [8] reported that the rumen to total stomach ratio of newborn calf is around 35% (95 g), but rapidly develops to 68% (2,040 g) at 17 weeks of age, and then maintains a similar rate until adulthood. In addition, the major microbial populations found in mature ruminants are already present in the rumen at 1–3 days of age [9,10]. It has been reported that it takes approximately 3 to 4 weeks to stabilize the inter-microbial community system after the initial microbiota is formed [11,12]. In general, rumen development can be largely divided into increase of rumen mass and papilla growth. Once the calf begins to consume solid feed and rumen fermentation is established, it undergoes both physical and metabolic development [13]. Physical stimulation by feed could increase rumen weight and muscle development [14], but volatile fatty acids are necassary for papilla development [15]. Soomro et al. [16] reported that feeding butyrate at 0.3/kg BW significantly increased the length and thickness of the rumen papilla, and Zhang et al. [17] reported that feeding 5% calcium salt propionic acid significantly increased papilla length and mRNA expression of GPR41, GPR43, and CCND1. For the simultaneous development of the rumen size (volume and muscle) and papilla, it is necessary to feed the calf with an appropriate proportion of concentrate and hay. Žitnan et al. [18] reported that feeding concentrate to young calves stimulated the development of the rumen epithelium (papilla), whereas feed with large particle size or high fiber are the main stimulant for rumen muscularization and volume. In the present study, the increase in rumen weight and improvement of papilla was higher than the overall weight gain in relation to the growth stages (newborn - 6 months) of the Hanwoo calves (Table 3 and Fig. 1). This suggests that similar to previous studies, rapid development of rumen masses and papilla occurs at the calf stage in Hanwoo cattle.
Considerable effort has been made to improve meat quality by increasing intramuscular adipogenesis. Most of these efforts focus on nutritional management in the finishing phase. On the other hand, studies on “Fetal programming [19]” and “Metabolic imprinting [20]” have been conducted to improve intramuscular fat through nutritional management during maternal (pregnancy) or at the calf stage. One of the important objectives of this study was to increase initial adipogenesis. Adipogenesis starts at mid gestation and persists after the birth of the calves. However, after approximately 250 days of age, it is more effective to increase the size of intramuscular adipocytes than to increase the number of intramuscular adipocytes due to the depletion of pluripotent cells [1]. In this study, the numbers of adipocytes in the LD and SM, and the mRNA expression of the adipogenic genes were higher in the SC than in NC, suggesting that in Hanwoo cattle, adipogenesis increases with the during growth of the calves, as reported in previous studies. Therefore, special supplements (energy, protein, vitamins, etc.) during this period could be an important key to effectively improving intramuscular fat. In studies of other cattle breeds [21–23], grain and corn-based feeds during early weaning or early calf stages has been reported to increase intramuscular fat. This suggests that high energy supply to early calf is one of the major factors in adipogenesis.
Various genes are expressed during calf growth, and some specific genes affect muscle and fat development. MyoG is known to be involved in the stimulation of muscle growth by inducing myogenesis, and contributes in the formation muscle cells [24]. In addition, it has been reported to be directly involved in muscle growth (muscle hypertrophy), and affects carcass weight [25,26]. Myosin heavy chain is involved in muscle contraction and force generation [27], and there are four isoforms in the mammalian skeletal muscle [28]. Among these, MYH 1 has low adenosine triphosphatase (ATPase) activity, slow contraction, and is necessary for the maintenance of posture, and is often found in red muscles [28]. MYH 2A is abundant in muscles that are continuously in use, and metabolic activities are high in this muscle due to oxidation and glycolysis [29,30]. In the present study, the increase in mRNA expression of MyoG, MYH 1, and MYH 2A in SC than NC is considered to be due to the muscle fiber hypertrophy resulting from growth, and increase in muscle activity. In addition, the reason for the higher expression of mRNA in SM than LD could be attributed to its role in supporting the body and its involvement in mobility.
On the other hand, adipocytes play an important role in energy regulation and homeostasis, and the differentiation of matured adipocyte is known to be regulated by transcription factors such as C/EBP family and PPARγ [31]. In particular, C/EBPβ not only regulates the expression and activity of PPARγ, but it has also has been reported to be involved in the synthesis of PPARγ ligand [32]. Differentiation of adipocytes is also caused by stem cells present in the muscle [33], and proliferation, differentiation and size of adipocytes in muscles are closely related to marbling score [33,34]. Mitochondria use energy and fatty acids to form acetyl-coA, which is used as a precursor for intramuscular fat synthesis [4]. Acetyl-coA synthesizes long chain fatty acids (saturated fatty) through the actions of various enzymes, and finally SCD converts saturated fatty acids into monounsaturated fatty acids to enable the accumulation of fat [35]. In the present study, we found that the mRNA expression level of SCD was increased in both LD and SM in relation to the calves growth stage, indicating fat accumulation was actively progressing. In addition, although it was inconsistent in both LD and SM, it was found that adipogenesis continued during calves stage because the mRNA expression of C/EBPβ and PPARγ tended to significantly increase with calves growth. Etoh et al. [36] reported that PPARγ and SCD expression was continuously increased in LD of 45 day, 4- and 10-month-old calves, and C/EBPα showed little difference between 45 days and 4 months, and it tended to increase at 10 months of age. It was concluded that the difference in feed quality during the early growth stage of calves has a substantial effect on the expression of genes involved in adipogenesis and fatty acid synthesis.
CONCLUSION
In the results of this study, Hanwoo calves were found to become significantly increased rumen weight and papiila length for 6 months after birth, and increased adipocytes formation and mRNA expression of myogenic-adipogenic makers in skeletal muscle. Thus, the calves period suggests that it is an important step in the development of the rumen for digestion and absorption and the myogenesis and adipogenesis. In addition, it is necessary to investigate additional data during the growing and fattening period, and further, studies such as the adjustment of nutritional levels, functional substances, and exploration of genetic markers to promote growth, rumen development, and adipocytes formation during the calves period are needed.