INTRODUCTION
Carcass weight (CW) is an important variable that affects pork quality and profitability of finishing pig production. CW is closely related to slaughter weight (SW). The average SW in the United States continues to grow over the past 80 years. It was 125.6 kg in 2013 [1] which is higher than recommended SW of 110 kg in South Korea. SW in Korea is about 5 to 15 kg lower than in that in Western countries as a result of an increase in genetic selection of lean-type pigs worldwide over the past few decades. Such lower SW is often considered undesirable for Korean consumers who are tolerant of animal fat. Korean meat consumers, unlike Westerners, sometimes prefer fat-rich cut such as pork belly [2].
There have been many research reports on the effect of CW or SW increase on pork quality and sensory properties. However, results are inconsistent, including positive effect [3] and negative effect [4–6]. Alternatively, some studies have reported significant changes in pork quality and sensory properties with increasing SW [7,8], while others have reported no effect of SW increase [9]. Such discrepancy about the effect of SW increase among studies might be due to differences in genetics, gender and (or) age of pigs used in various experiments [10–12]. In addition, changes in pork quality with increasing CW or SW might be due to changes in muscle fiber composition and muscle metabolic traits. When live weight increased from 95 to 130 kg, a greater total number of type I muscle fiber and their size in the longissimus muscle of pigs have been reported [7,13]. In addition, better sensory properties of pork from barrows compared to gilts could be due to greater intramuscular fat (IMF) content [14–16] and lower muscle glycolytic potential [17]. Therefore, the aim of this study was to evaluate effects of CW increase on meat quality and sensory properties in relation to IMF content and ultimate pH (pHu) of pork from barrows and gilts.
MATERIALS AND METHODS
A total of 96 pork carcasses from LYD (Landrace × Large White × Duroc) crossbred barrows (n = 48) and gilts (n = 48) were selected at a commercial slaughter house. All pigs used in the experiment were raised on the same farm with the same feed and specification methods. Each gender group had commercial CW (≤ 90 kg, CCW), heavy CW (91–100 kg, HCW) and very heavy CW (> 100 kg, VHCW) (16 pigs for each CW group). Loin cuts were excised at 24 hr postmortem and transported to the laboratory to measure meat color, water-holding capacity (WHC), tenderness, fat content and sensory properties.
Backfat thickness was measured at the central part of the loin cut (the last rib area). Steaks with thickness of 3 cm were made in the direction from the center to the back for measuring the following in order: 1) meat color, muscle pH, and IMF content, 2) drip loss (DL) and released water (RW), 3) cooking loss (CL) and Warner-Bratzler shear force (WBSF), and 4) sensory properties with a panel test.
The pH of pork loin muscle was measured using a pH meter (MP 230, Mettler Toledo, Greifensee, Switzerland) after mixing approximately 3.0 g of sample with 27 mL of deionized water and homogenized (T25 ULTRA-TURAX, IKA, Königswinter, Germany) for 30 seconds. The surface color of pork steaks was measured using the Minolta Chromameter (CR -300, Minolta, Tyoko, Japan). Before measurement, the instrument was standardized with a white plate (Y = 93.5, X = 0.3132, y = 0.3198).
DL (%) was measured using a plastic box method [18]. A meat sample of a standardized sized (2 cm diameter × 2 cm thickness) was hung in a plastic box (18 × 15 × 10 cm) and left in a 4°C refrigerator for 24 hrs. The weight loss during suspension was measured and expressed as a percentage loss based on the weight of the initial sample. CL (%) was determined by weight loss caused by constant heat treatment on meat samples. Sample (2 cm diameter × 2 cm thickness) contained in a plastic bag was treated with heat in a 75°C water bath for 30 minutes and then the weight loss based on the initial weight was expressed as a percentage. RW (%) was measured by the method of Joo [19]. Briefly, a meat sample (3.0±0.05 g) was placed between two thin plastic films and pressed with a load of 2.5 kg, then the weight of the water drained from the original sample weight was calculated as a percentage.
WBSF (kg/cm2) was measured using an Instron Machine (Universal Testing Model 3343, Instron, Norwood, MA, USA). After measuring CL, 6 shear force samples (0.5 cm × 4.0 cm) per loin stake were prepared using a stainless core. Instron with a V-shaped shear blade cut at right angles to the direction of the muscle fiber. The crosshead speed of the instrumental was set at 100 mm/min and the maximum scale load was 50 kg.
IMF content was determined using the Folch et al. method with some modification [20]. Briefly, a homogenized meat sample of 3 g was added with 30 ml of Folch solution I (chloroform : methanol = 2 : 1, v/v) and the lipids were extracted. The solution filtered by Whatman no.1 filter paper was stirred with 0.88% NaCl and separated into two layers in the cylinder. The final volume of the lower layer was recorded after washing the cylinder wall with 10 ml of Folch solution II (chloroform : methanol : H2O = 3 : 47 : 50). The upper layer (methanol and water layer) was removed using an aspirator and 10 mL of the lower layer (chloroform containing lipid extracts) was taken into a dish to dry at 50°C. The weight of the dish was measured before and after drying. Fat content was computed based on weight difference of the dish.
Sensory evaluation was performed according to the modified Spectrum TM method for descriptive analysis [21] by eight trained panelists with more than 50 hrs of experience in pork assessment. Panelists evaluated samples for flavor, juiciness, tenderness, and palatability using a 9-point hedonic scale (1 point, “extremely dislike” to 9 point, “extremely like”).
This experiment had a completely randomized design with a 2 (gender; barrow vs gilt) × 3 (CW; CCW vs HCW vs VHCW) factorial arrangement of treatments. All data of meat quality measurements and sensory evaluation were analyzed using general linear model procedure of Statistical Analysis System (SAS) ver. 9.3 [22] and a model containing gender, CW, and interaction of these terms. Significant differences were declared at p < 0.05. Pearson Correlation coefficient was obtained to describe the relationship between CW and meat quality traits and sensory properties using SAS PROC.
RESULTS AND DISCUSSION
As shown in Fig. 1, backfat thickness and IMF % increased as CW increased in both gilts and barrows. Backfat thickness and IMF % of VHCW were significantly higher than those of CCW (p < 0.05). There was no significant difference in IMF % or backfat thickness between gilts and borrows. This increase of backfat thickness with increasing CW is similar to results of previous studies [1]. From previous 25 studies, when marketing weight of a pig was increased by 10 kg, the backfat thickness was increased by an average of 1.8 mm [1]. In the present study, as CW increased by approximately 20 kg, backfat thickness was increased by 6.0 mm for gilt and 6.2 mm for barrow. Results of relatively thicker backfat thickness and greater IMF content in barrows than in gilt were similar to results of our previous studies [2, 23–25].
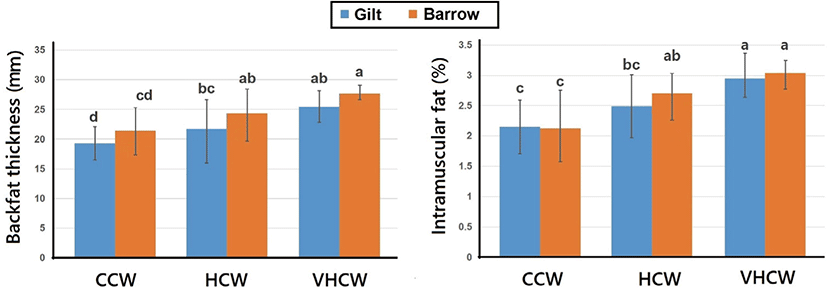
The pHu was not affected by either CW or gender (Table 1). Many studies have reported that pH does not change when SW is increased [26,27]. However, some studies have reported a slight decrease [28] or increase in pH [3,29] when SW is increased. No significant difference in pHu among CW groups suggests that the increase in CW will not result in an increase in PSE (pale, soft, and exudative) meat incidence due to a slow cooling rate for large carcass.
Among meat quality measurements, only ra* and WBSF showed significant (p < 0.05) differences among CW groups (Table 1). There was no significant difference in L* or b* values among CW groups. The result that L* value was not changed with increasing CW was the same as our previous studies [2,24,25] and others [28,30]. However, Virgili et al. [11] have found a 0.01 unit reduction in L* value with every 10 kg increase in SW. Regarding a* value, increases in a* value with increasing CW were observed for both gilts and barrows, consistent with results of Đurkin et al. [30] and Latorre et al. [26], but different from other studies showing no significant differences in a* value with increasing CW [11,28]. Overall, with increasing CW or SW, results of L* and a* values were different between reports. Because meat color is the number one factor affecting consumers’ decisions when purchasing meat, further studies are needed to determine changes in meat color due to CW increase.
As shown in Table 1, CW increase has no effect on WHC measurement. There was no significant (p > 0.05) difference in DL %, CL %, or RW % among CW groups. The pHu was not affected by gender either, different from our previous result showing DL was greater in gilts than in barrow [2]. Although the reason for such difference between studies was unclear, DL % in the present study was generally lower than DL % of PSE pork which was over 5.0 %. This suggests that the incidence of PSE occurring at over 5.0 % of DL [31] is not likely to be influenced by gender or CW. Moreover, effects of increasing SW on DL % are inconsistent among in many studies [4,11,28,30]. In the present study, insignificant differences for all WHC measurements might be due to no difference in pHu among CW groups. This is because WHC is readily affected by either pH decline rate or pHu.
Results of the present study showed that when CW increased, pork loin became tougher for both gilts and barrows (Table 1). There were significant (p < 0.05) differences in WBSF among CW groups. However, as with a* value, there was no significant difference in WBSF between gender groups (p > 0.05). WBSF results with increasing CW or SW are conflicting in many studies. There is no significant difference in WBSF of pigs weighing 70, 80, 90, and 100 kg or 116, 124, and 133 kg [26]. On the contrary, a slight reduction of 0.08 kg per 10 kg marketing weight increase in WBSF has observed [4]. A quadratic effect of body weight on tenderness has also reported. Pigs weighing 140 and 160 kg have greater WBSF values than pigs weighing 120, 150, and 170 kg [30]. Despite these conflicting results, our results clearly showed that when the weight of pork carcass was increased, so did the shear force of muscle. This result suggests that as pig weight increases, the tenderness decreases due to an increase (a* value increase) in red muscle fiber in loin muscle.
Differences in sensory properties with increasing CW in gilts and barrow are shown in Table 2. Flavor score was significantly increased while tenderness score was significantly decreased (p < 0.05) with increasing CW. There were no significant difference in juiciness and palatability score among either CW or gender groups (p > 0.05). These results were different from the observation by Huff-Lonergan et al. [32]. They observed significant and positive responses of juiciness and off-flavor presence with increasing CW. Cisneros et al. [4] have observed decreased tenderness and juiciness by 0.1 and 0.4%, respectively, for every 10 kg increase in market weight from 100 to 160 kg. However, Park and Lee [28] have observed no significant difference in flavor profile of cooked pork when marketing weight is increased from 116 to 133 kg.
A significant correlation between CW and sensory properties (except juiciness) was observed (Table 3). CW had a positive correlation with flavor (r = 0.59), while it had a negative correlation with tenderness (r = −0.70). The negative correlation between CW and tenderness was similar to results of Martin et al. [33]. They observed a slightly positive but significant relationship between increasing CW and shear force. Both juiciness and palatability showed stronger correlations with backfat thickness (r = 0.31 and 0.33, respectively) than with CW. IMF content also showed strong correlations with all sensory properties. These results indicated that an increase in fat content resulting from CW increase had a positive effect on sensory properties. pHu, L* and DL showed strong correlations with juiciness and palatability, while a* showed significant correlations with all sensory properties. WBSF showed a strong negative correlation with tenderness, while it showed no significant correlation with palatability. These results imply that as CW increase, flavor improves while tenderness deteriorates. Thus, the palatability is offset without changing too much.
CONCLUSIONS
Backfat thickness and IMF content of pork loin were increased with increasing CW. a* value increased and shear force decreased with increasing CW, but pHu, lightness L* value and all WHC measurements did not changed. As CW increase, sensory flavor score increased while tenderness score decreased. Consequently, CW has a positive correlation with flavor but negative correlation with tenderness. The increase in IMF content resulting from CW increase improves flavor, juiciness and palatability, although tenderness deteriorates with increasing CW. Therefore, an increase in CW is expected to improve the taste of pork.