INTRODUCTION
In response to the continuous global population growth, the Food and Agriculture Organization (FAO) of the United Nations (UN) has emphasized the need to develop novel and sustainable protein sources [1,2]. Edible insects require much smaller breeding areas and release significantly lower carbon emissions than livestock, and their high feed efficiency would meet the protein requirements to ensure human food security [3]. Moreover, edible insects have been evaluated as meat protein substitutes and are rich in fat, minerals, and vitamins, with excellent nutritional and functional properties [4].
The nutritional qualities of dietary proteins are often determined by the total nutrient content as well as digestion and absorption rates in the gastrointestinal tract. The exoskeletons of adult insects consist of chitin-bound sclerotized proteins and glycoproteins [3,5]. The digestive enzyme accessibility and microbial solubility of chitin are low [5]. Marono et al. [6] reported the negative effects of chitin on in vitro protein digestibility in insects and Manditsera et al. [7] observed that protein digestion in adult insects is inhibited due to the high contents of chitin and sclerotized proteins. Thus, the larval stage, with its low chitin and sclerotized protein contents and the absence of the exoskeleton is the main raw material for protein products [8]. Bosch et al. [4] reported that the protein digestibility of Tenebrio molitor and Alphitobius diaperinus larvae was approximately 91%. Manditsera et al. [7] reported that insect protein digestibility was approximately 5% lower than that of whey protein, indicating excellent digestibility.
Although edible insects exhibit excellent nutritional qualities and protein digestibility, consumers are often be bothered by their appearance. Kim et al. [9] surveyed consumer perceptions of entomophagy, showing that approximately 32% of the respondents indicated they would refuse to eat edible insects in because insects were “disgusting”. To overcome this perception, edible insects can be processed by several methods, including grinding and extraction, to mask their appearance when added to food. As insects are generally considered to be rich in fat, protein extraction often involves defatting using organic solvents [10]. Although these processing methods can improve the sensory qualities or functional properties, including flavor, color, and taste of edible insects, protein digestibility can be decreased due to protein denaturation or aggregation during processing [11].
Edible insects that have been temporarily recognized as food ingredients in South Korea include Allomyrina dichotoma and Protaetia brevitarsis larvae. P. brevitarsis larvae are well-known protein sources with excellent physiological qualities and processing abilities [2]. Kim et al. [12] reported the excellent essential amino acid composition and protein quality of P. brevitarsis larvae, while Lee et al. [13] reported excellent antioxidant activity. However, to the best of our knowledge, no report has addressed the protein digestibility of P. brevitarsis larvae.
Therefore, the protein digestibility and quality properties of whole and defatted powder of P. brevitarsis larvae were investigated in the in vitro protein digestion model and it was compared with a representative animal protein, beef loin.
MATERIALS AND METHODS
Beef sirloin (USDA choice grade) frozen at −20°C was purchased from a local market and frozen fasted P. brevitarsis larvae were obtained from Farm bang in Korea and stored at −70°C after freeze-drying. Samples were lyophilized using a freeze-drier (Bondiro, Ilshin, Seoul, Korea) and then pulverized using a model FPM250 grinder (Kenwood, Havant, UK). For defatting P. brevitarsis larvae, 30 g of ground larvae was added to 600 mL of 70% ethanol and homogenized at 13,000 rpm for 1 min in a model T25 basic apparatus (IKA GmbH & Co. KG, Staufen im Breisgau, Germany). The homogenate was centrifuged at 6,710×g for 30 min, and the supernatant was removed. After 600 mL of 70% ethanol was added to the pellet, the mixture was homogenized and centrifuged again under the same conditions. This process was repeated (total of three times), and the final precipitate was freeze-dried. The freeze-dried beef flour (BF), insect flour (IF), and ethanol-defatted insect flour (IE) were stored at −20°C before analysis.
The proximate moisture, crude protein, crude fat, and crude ash compositions of the powder samples were estimated according to AOAC methods [14].
Total aerobic bacteria, Escherichia coli, and coliform bacterial counts in the powders were determined using previously described methods [15]. Briefly, 3 g of powder and 27 mL of sterile saline solution were mixed using a stomacher for 2 min. The mixture was sequentially diluted 10-fold using sterile saline. After incubation in 0.1 mL of diluent on a solid plate count agar (Difco Laboratories, Detroit, MI, USA) at 37°C for 48 h, the total aerobic bacteria were counted. E. coli and coliforms were enumerated after dispensing 1 mL of the diluent in a coliform count plate (3M Health Care, St. Paul, MN, USA) and estimated after incubation at 37°C for 24 h. The number of microorganisms is expressed as log CFU/g.
The digestive fluid composition was determined using a previously described method [16]. After the protein concentration was adjusted to 48.5 mg/mL with distilled water, the samples were heated at 80°C in a water bath for 30 min to simulate cooking. After cooling, the samples were homogenized at 13,000 rpm for 1 min (T25 basic). The in vitro digestion of the powder homogenates was performed using a previously described method [17]. After supplementing the homogenate (4 mL containing 194 mg protein) with 10 mL of gastric fluid, the mixture was stirred for 2 h in a shaking water bath at 37°C. To simulate small intestinal digestion, 10 mL of duodenal fluid and 5 mL of bile solution were added to the chyme and stirred under the same conditions used for the gastric phase. The digesta was immediately frozen at −70°C to terminate the digestion reaction and stored at that temperature until further analysis. The digestion was performed three times (in three batches) on different days for statistical analysis.
The powder and digestive fluid samples were homogenized after distilled water was added to achieve a final protein content of 149.4 mg/mL (T25 basic). The final volume of the homogenate was adjusted to 10 mL using distilled water. The homogenate and digested samples were mixed in a 2× sample buffer (125 mM Tris-HCl, 20% glycerol, 2% SDS, 2% mercaptoethanol, and 0.02% bromophenol blue) at a 1:1 (w/w) ratio, and then heated at 95°C in a heating block. For the 12.5% SDS-PAGE, 10 μL of the sample (74.7 μg protein) and 5 μL of pre-stained protein ladder (3454A, TaKaRa Bio, Shiga, Japan) were loaded and run at 20 mA for 240 min in running buffer containing 0.1% SDS, 25 mM Tris, and 192 mM glycine using pagerRun (AE-6531 mPAGE, ATTO, Tokyo, Japan). The protein bands were stained using a mixed solution of Coomassie brilliant blue and 10% acetic acid and subsequently scanned using a model GS-710 apparatus (Bio-Rad Laboratories, Hercules, CA, USA).
To measure the α-amino group content of the powder, 10 mL of 10.4% trichloroacetic acid was added to 0.4 g of the powder, followed by homogenization at 13,000 rpm for 1 min (T25 basic). The mixture was centrifuged at 2,063×g for 20 min in a model 1580 R centrifuge (LABOGENE, Lynge, Denmark) and the supernatant was filtered using No. 4 filter paper (Whatman, Maidstone, UK). The hydrolysis rate of the digestion reaction was determined using 1 g of powder mixed with 6 mL of 6 N HCl. Complete digestion was achieved by heating at 100°C for 24 h [18].
The α-amino group contents were measured by reacting the samples with o-phthal-dialdehyde (OPA) [19]. The OPA reagent was freshly prepared 20 min before use with a mixture containing 25 mL of 100 mM sodium tetraborate, 2.5 mL of 20% SDS, 40 mg/mL of OPA in methanol, and 100 μL of beta-mercaptoethanol. The solution volume was adjusted to 50 mL with distilled water. For the experiment, 150 μL of the sample and 1 mL of OPA reagent were reacted for 2 min at room temperature in a model DU®530 spectrophotometer (Beckman Instruments, Brea, CA, USA) to determine the absorbance at 340 nm. Glycine was used to establish a standard curve and the protein content was measured using the Kjeldahl method [14]. The α-amino group contents were calculated and reported as μM NH2/g protein. The digestive hydrolysis rate (%) was calculated using the following formula:
The examinations were performed in triplicate (three batches) and the data were statistically analyzed using mixed models by a randomized complete block design (each batch corresponding to a block). The least square means and standard error of the least square means are presented, and Tukey’s multiple range test was used to distinguish significant differences (p < 0.05). SAS version 9.3 software (SAS Institute, Cary, NC, USA) was used to perform the statistical analyses.
RESULTS AND DISCUSSION
Several studies have investigated the nutritional composition of insect species [3,20]. Edible insects are high-quality protein sources rich in fats and minerals [21]. The crude protein content was significantly higher in IF (66.65%) and IE (69.28%) than in BF (59.53%; Table 1, p < 0.05), in agreement with previous studies showing the high protein content of P. brevitarsis larvae [22,23]. The protein content of the insect samples increased after defatting (p > 0.05), as observed previously [12], likely due to the fat removal resulting in an increased crude protein content in IE. The crude ash content of IF was significantly higher than that of BF (p < 0.05), but no significant difference was observed between those of IE and BF (p > 0.05).
Treatment | Moisture | Crude protein | Crude fat | Crude ash |
---|---|---|---|---|
IE | 3.17A | 69.28A | 24.48B | 3.08B |
IF | 1.36AB | 66.65A | 26.81B | 5.18A |
BF | 0.00B | 59.53B | 37.42A | 3.16B |
SEM1) | 0.692 | 2.797 | 1.230 | 0.403 |
The crude fat content was highest in BF, and no significant differences were observed between IE and IF. Previous studies have reported decreased crude fat content after defatting of edible insects with organic solvents, but this was not observed herein. The differences in the crude fat content are likely due to the different larval defatting methods used. Kim et al. [12] and Purschke et al. [24] defatted for > 1 h after adding hexane to the insect powder. Zhao et al. [25] defatted mealworms by immersion in 99.5% ethanol overnight. However, 70% ethanol was used herein as the defatting solvent for a short time, which may be the reason for the lack of a significant decrease in fat content of P. brevitarsis larvae during defatting.
Although the physicochemical and functional properties of edible insect proteins have been evaluated, limited studies have been conducted regarding their microbiological properties [21]. Herein, the counts of total aerobic bacteria, E. coli, and coliform bacteria were determined to monitor changes in the potential microbiological properties before and after defatting with ethanol (Table 2). The total aerobic bacteria of IF was significantly higher than that of BF (8.71 vs. 1.52 Log CFU/g; p < 0.05), while E. coli and coliform bacteria were not observed in the BF, but were present in IF (0.81 and 5.71 Log CFU/g, respectively; p < 0.05). Insects are bred in close contact with soil or sawdust in containers contaminating the surface and body of insects with microbes during breeding [21,26]. In addition, contamination of edible insects by spores present in the feed has been reported [21], which may explain the increased the number of total aerobic bacteria, E. coli, and coliform bacteria in the IF samples compared to BF samples.
Treatment | Total aerobic bacteria | Escherichia coli | Coliform |
---|---|---|---|
IE | 5.37B | NDB | NDB |
IF | 8.71A | 0.81A | 5.71A |
BF | 1.52C | NDB | NDB |
SEM1) | 0.129 | 0.138 | 0.015 |
Total aerobic bacteria in IE was 5.37 Log CFU/g, significantly lower than that in the IF group (p < 0.05), while E. coli and coliform bacteria were not detected in IE. Previous studies have also reported the antibacterial effect of the 70% ethanol defatting procedure. Crippen and Sheffield [27] showed that the outer layer of the mealworm was removed and microorganism number reduced by 60% when treated with 70% ethanol. The main mechanisms underlying the antibacterial effects of ethanol involve protein denaturation and aggregation. The hydroxyl group (−OH) in ethanol binds to the proteins of the microorganisms by forming the hydrogen bonds resulting in the damage to their structure and functionality [28]. Thus, the sterilizing effect of ethanol is due to the inhibition of microbial enzymes and protein degradation. Ingram [29] reported that ethanol treatment induced cross-linkage of peptidoglycans and degraded E. coli. Because as fat can be dissolved by ethanol, the waxy layer constituting the larval surface might be solubilized and ethanol penetration increased [27].
Ethanol has been reported to exhibit low toxicity as a fat extraction solvent and applying 70% ethanol in the extraction process of insect powder is expected to reduce the number of aerobic bacteria [30,31]. However, to use edible insects as food ingredients, standard specifications, or sterilization methods must be systematically established for safety purposes.
Free amino acids and small three to four residue peptides are soluble in 10% trichloroacetic acid (TCA) [16]. The α-amino group content of the 10% TCA soluble fraction was measured to compare the content of small amino groups in the insect and beef samples (Table 3). The α-amino group content of IF was 152.88 μM/g, significantly higher than that of BF (27.53 μM/g; p < 0.05). Thus, P. brevitarsis larvae flour contains larger amounts of small peptides and free amino acids compared to BF. Bioactive peptides exhibit antioxidant, antihypertensive, and mineral-binding activities [32] and several studies have confirmed the presence of low molecular weight bioactive peptides in edible insects including P. brevitarsis larvae [32–35]. Previous studies have reported the presence of innate proteases in insect bodies [36,37]. In live insects, innate proteases participate in the defense system, while Bang et al. [36] and Chang et al. [37] reported that serine proteases are present in the larvae and maggot of P. brevitarsis. Autolysis can be caused by the degradation of skin and muscle proteins by endogenous proteases [18]. Moreover, Tonheim et al. [38] reported that autolysis of proteins in the insect can occur even during extraction. Therefore, the high content of the α-amino group in IF may be due to autolysis caused by innate proteases.
Treatment | α-amino group content (μM/g) |
---|---|
IE | 34.65B |
IF | 152.88A |
BF | 27.53C |
SEM1) | 1.314 |
IE showed decreased α-amino group content (34.65 μM/g), significantly lower than that of IF (p < 0.05). The hydroxyl radicals present in 70% ethanol can bind to insect proteins and induce protein denaturation and aggregation [28]. Liu and Xiong [39] reported decreased α-amino group content following the oxidation and denaturation of meat proteins. Ethanol use for fat extraction may reduce the α-amino group content via protein polymerization and aggregation.
These trends were also observed by SDS-PAGE (Fig. 1). The BF bands were evenly distributed at all molecular weights and a high intensity band was observed migrating with a molecular mass exceeding 200 kDa, likely a myosin heavy chain [39]. However, most insect bands migrated with a molecular mass of < 45 kDa. According to Kim et al. [12], bands under 20 kDa in insect samples are likely anti-freeze and hemolymph proteins with molecular weights below approximately 13 kDa. Herein, it was confirmed that the molecular weight distribution of the proteins present in the P. brevitarsis larvae flour and BF differed significantly.
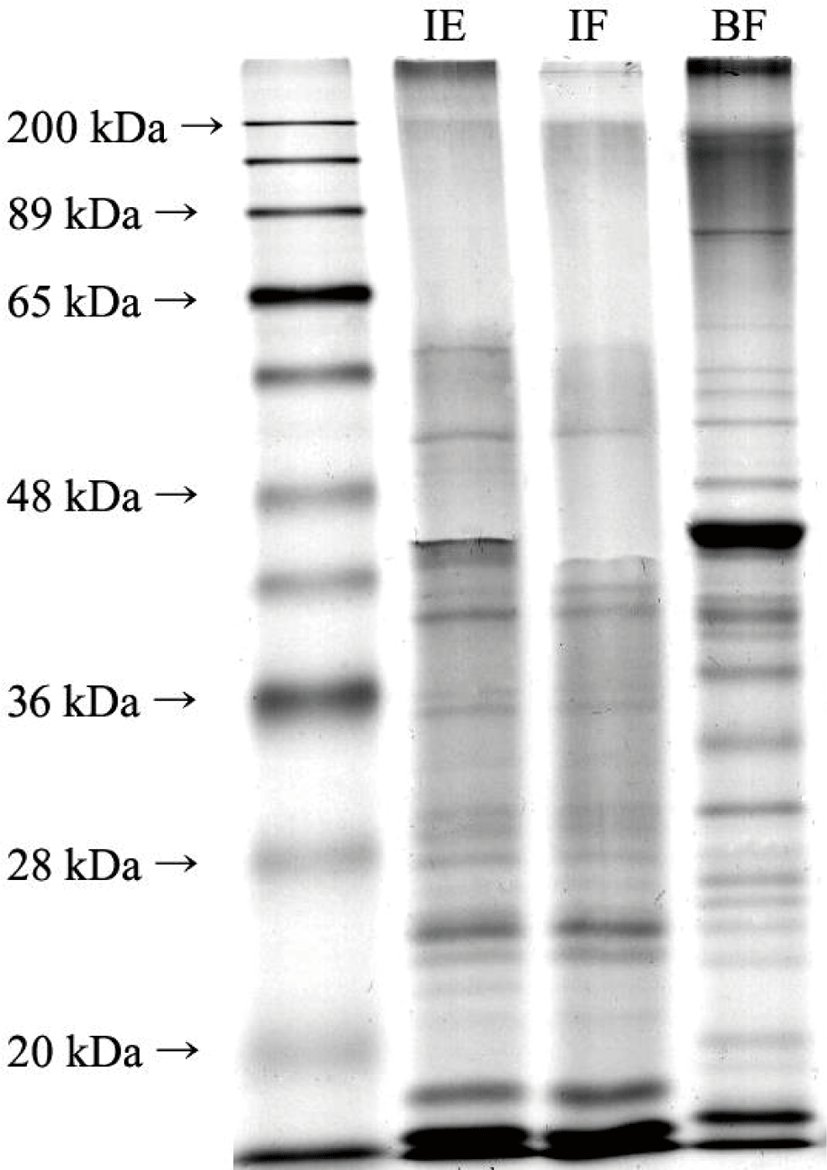
Differences in band distribution and intensity were observed before (IF) and after (IE) defatting. IE displayed bands over 200 kDa, while IF did not. In addition, a band near 60 kDa was faintly visible in the IF, while the same band was present at a relatively high intensity in IE. These changes in band intensities and distribution could be due to the denaturation or aggregation of proteins during the ethanol defatting process.
The SDS-PAGE results and α-amino group contents of the larvae flour indicated that P. brevitarsis larvae contain more low molecular weight peptides and amino acids compared to BF. Thus, defatting with ethanol likely induces larval protein denaturation.
Protein digestibility is often used to evaluate protein quality and the digestibility of animal proteins is excellent [16,40]. Thus, it is necessary to evaluate insect protein digestibility if they are to replace animal proteins in the human diet. The in vitro protein digestibility of IF was 54.91%, similar to that of BF (50.58%; Table 4). Kinyuru et al. [11] reported that the in vitro protein digestibility of insects is similar to that of meat and whey proteins. However, Jonas-Levi and Martinez [41] suggested that insect protein digestibility is overestimated by glycoproteins bound to chitin, which cannot be digested in vivo. Because adult insects have sclerotized proteins and chitin in their exoskeleton, concerns have been raised regarding their digestion in the human digestive tract. Therefore, as Finke [8] reported that larvae can be easily digested because of their soft body and low chitin content, larvae were used to as a protein source with the potential to replace meat proteins. The in vitro protein digestibility of P. brevitarsis larvae was considered to be excellent, similar to that of beef. The protein digestion rate can be increased by processing through the structural changes of proteins and inactivation of anti-nutritional factors that bind to proteins, inhibiting digestion [42]. Janssen et al. [43] observed that oxidation-related denaturation and aggregation of proteins during processing decreased protein digestibility by reducing their accessibility towards digestive enzymes. Megido et al. [42] reported that in vitro protein digestibility increased during the boiling and baking of mealworms in an oven. However, Kinyuru et al. [11] reported decreased in vitro protein digestibility during the baking and drying of grasshoppers. These differences likely originate from the various degrees of protein denaturation during processing. Herein, aggregation occurred in the larvae protein after defatting, as indicated by the results of α-amino group content and SDS-PAGE. However, the digestibility of the larva after defatting did not significantly differ (Table 4). Chamba et al. [44] confirmed that the surface hydrophobicity of soybean protein extract increased after defatting with hexane. Most proteins have a hydrophobic core consisting of internal non-polar amino acids, while their exterior is composed of polar amino acids [45]. External factors can alter surface hydrophobicity and digestive accessibility of the proteins. As the ordered structures of proteins loosened to form an irregular shape due to physiochemical damage during processing, aliphatic amino acids, including leucine, isoleucine, and valine, are exposed to the surface, and hydrogen bonds are collapsed [46]. Protein aggregation occurred in IE, but some buried hydrophobic groups may have been exposed to the outside during defatting by ethanol. Moreover, pepsin exhibits substrate specificity for aromatic amino acids, including phenylalanine, tyrosine, and tryptophan [47]. If defatting with 70% ethanol induced an increase in surface hydrophobicity in IE, the digestive accessibility of pepsin to phenylalanine and tryptophan may have increased [48]. Therefore, no significant differences in in vitro protein digestibility were observed between IE and IF, as protein aggregation and unfolding occurred at the same time in the insect proteins.
Treatment | Protein digestibility |
---|---|
IE | 46.58 |
IF | 54.91 |
BF | 50.58 |
SEM1) | 2.518 |
The SDS-PAGE results for the in vitro gastrointestinal digesta indicated excellent in vitro protein digestibility of P. brevitarsis larvae and beef loin (Fig. 2). All bands in the sample (Fig. 1) disappeared after digestion (Fig. 2), where only bands below 36 kDa were recognizable and were present only at extremely low intensities. This indicated that the proteins in the P. brevitarsis larvae and beef loin were cleaved by digestive enzymes during in vitro digestion and could not be observed in the 12.5% gel. In addition, a 20% SDS-PAGE was run to examine smaller molecular weight bands, and a few recognizable bands were evident in the gel (data not shown).
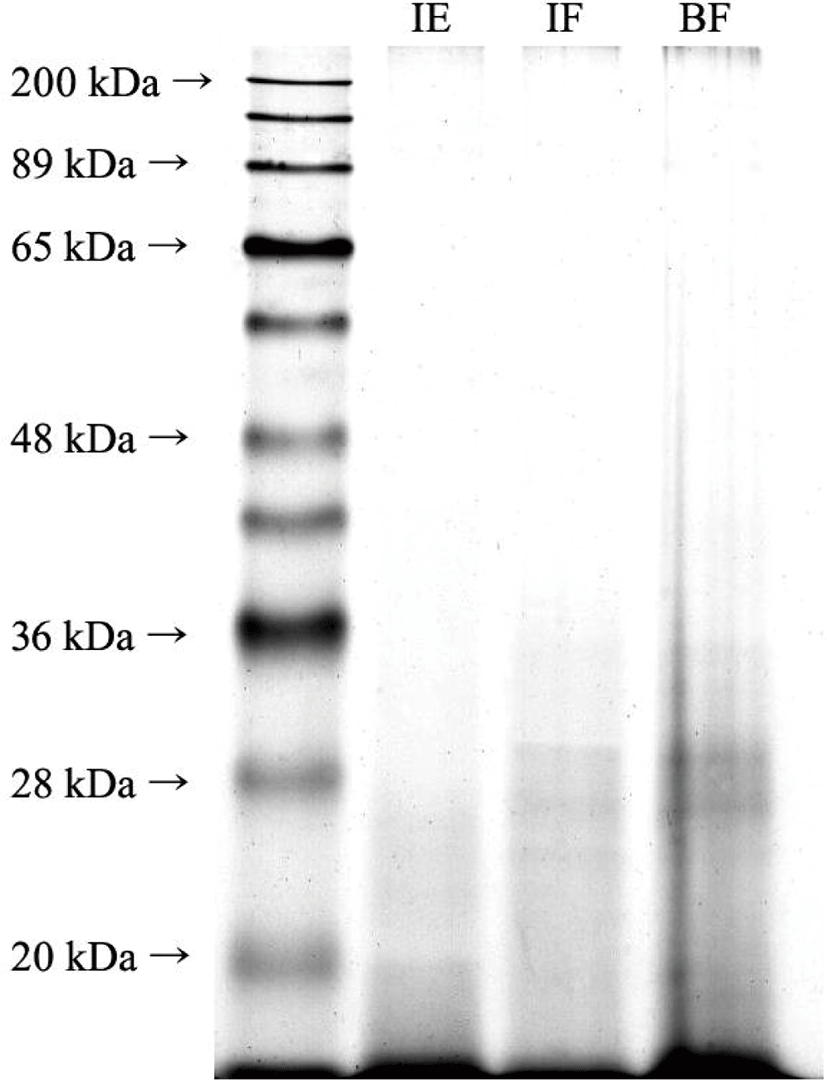
The observed proteolysis was lower than that reported previously by Kinyuru et al. [11], with the in vitro protein digestibility of grasshopper of > 80%. Bosch et al. [4] demonstrated that the in vitro digestibility of T. molitor and A. diaperinus exceeded 91%, but Janssen et al. [43] reported that the in vitro digestibility of T. molitor, A. diaperinus, and Hermetia illucens was 10%–30%. Manditsera et al. [7] confirmed that the in vitro digestibility of Eulepida mashona and Henicus whellani was 30.6% and 29.7%, respectively. These differences can be attributed to the design of the in vitro digestion model, method of evaluating protein digestibility, types of edible insects examined, and the breeding stage [7]. Therefore, the absolute value of the in vitro protein digestibility obtained herein differed from those previously reported.
In conclusion, the proximate composition of the samples indicated a high crude protein content in P. brevitarsis larvae. Defatting insect samples with 70% ethanol reduced the total aerobic bacteria, E. coli, and coliform counts. Small molecular weight amino acids and peptides were more evident in the insect samples compared to the beef samples. Although defatting IF with 70% ethanol induced a decrease in the α-amino group content in IE, no significant differences in in vitro protein digestibility were observed among the three sample treatments. Therefore, larvae of P. brevitarsis exhibited excellent protein digestibility and crude protein content, showing great potential as a substitute for beef protein. Furthermore, defatting the larvae of P. brevitarsis with 70% ethanol increased microbial safety without impairing the in vitro protein digestibility.