INTRODUCTION
Salt and phosphate are key additives that play an important role in the quality of meat products. However, excessive additives intake adversely affects human health. Because of these potential risks, consumers want to have a food reduced ingredients. Thus, consumers want foods that have reduced or eliminated additives, so-called “clean label” foods, because additives are considered unsafe and potentially harmful by consumers [1]. Due to greater scientific literacy and popular sources of media information, consumers are well aware of the relationship between sodium and diseases, in particular hypertension. This leads to increasing demand for low salt products in many countries. Salt gives meat products salty flavor which is due to Na+ with Cl− modifying the perception [2,3]. It also solubilizes the myofibrillar protein in meat products and improves meat product gelation, hydration, water-binding capacity, cooking loss (CL) and texture [3]. The addition of phosphate leads to increased electronegativity and the electrostatic repulsion of protein interaction. Therefore, protein dispersed in water has greater solubility, and emulsifying and foaming ability when phosphate is present [4]. It can also increase pH of meat products, dissociate actin and myosin and bind to the meat protein [5,6]. Among the phosphate compound, sodium tripolyphosphate (STPP) has been widely using in the meat industry because of its safety, low cost and simple operation [4,7]. The salt effect is enhanced by phosphate, because NaCl and phosphate act synergistically in the depolymerisation of thick filaments [6]. In addition, many studies have reported that the combination of salt and phosphate contributes to quality improvements (i.e., binding property, water retention, emulsion stability, sensory property) of meat products [8,9]. To develop a clean label meat product, we evaluated the optimal combination to reduce additives without significantly impairing the quality according to the amount of salt and phosphate added. Therefore, this study was performed to evaluate quality of chicken breast sausage containing various combinations of salt and STPP.
MATERIALS AND METHODS
Raw chicken breasts were purchased from the local market and stored at −70°C in freezer until used. Frozen chicken breasts were thawed overnight at 4°C. After thawing, the meat was ground by meat chopper (M-12S, Hankook Fujee Machinery, Gyeonggi, Korea) and used for the manufacture of chicken model sausages.
Table 1 shows the formulation of model chicken sausages with varying salt levels (T1–T5). Model sausages were processed with 0.3%, 0.8%, 1.3%, 1.8%, and 2.3% salt, but actual salt content was 0.5%, 1.0%, 1.5%, 2.0% and 2.5%, since cure blend included the salt (~0.2%). Ground chicken breast was mixed with ice water and non-meat ingredients (salt, STPP, sodium erythorbate and cure blend) using a food mixer (HMC-401, Hanil Electric, Seoul, Korea) for 3.5 min. Approximately, 40 g of sausage batter was placed into 50 mL conical tubes (SPL Life Science, Gyeonggi, Korea) and centrifuged at 1,660×g for 2 min using a centrifuge (VS-5500, Vision Science, Seoul, Korea) then sausages were cooked at 75°C for 30 min. When cooking was done, the cooked sausages were placed in an ice box for 30 min, and then stored at 4°C until utilized.
pH of sausages was measured on five times using pH-meter (MP-120, Mettler-Toledo, Greifeense, Switzerland). The inner surface of sausages was used to measure their color values. Lightness (CIE L*), redness (CIE a*) and yellowness (CIE b*) were evaluated on six times using the color reader (CR-10, Minolta, Tokyo, Japan).
Water-holding capacity was performed by modifications base on the method described in Jauregui [10]. Sausage samples cubes (1.5 g) were prepared, and then wrapped with three pieces of Whatman #3 filter paper. After samples and filter paper pieces were weighed, they were centrifuged at 1,660×g for 15 min, and then weights were measured again. The expressible moisture (EM) was calculated by the equation. EM (%) = (filter paper weight after centrifuge − original filter paper weight) / original sample weight × 100. The CL was calculated by weight difference after cooking. CL (g) = sample weight (40 g) before cooking − sample weight (g) after cooking.
Ten pillar shaped samples were prepared using a puncturing tool. Sample’s height was 13 mm and diameter was 12.5 mm. An Instron Universal Testing Machine (Model 3344 testing device, Norwood, MA, USA) was utilized to analyze the textural quality of chicken breast sausage. The sample height was reduced to 75% of the original height after samples were compressed two times sequentially. A compression probe was utilized with a 500 N load cell and the crosshead speed was 300 mm/min. Hardness (gf), springiness (mm), gumminess, chewiness, and cohesiveness were measured following the method of Bourne [11].
Table 2 indicate the formulation of model chicken breast sausages with various combination of salt and STPP. Model sausages were made with 0%, 0.8%, or 1.8% salt and with or without 0.3% STPP and mixing was performed using a food mixer (HMC-401, Hanil Elecric, Seoul, Korea) for 3.5 min. The following measurements were the same as in Experiment 1.
pH and color measurement were performed as in Experiment 1. Proximate analysis was measured by AOAC method [12]. Moisture was measured by dry-oven method, crude fat content by Soxhlet extraction method, and crude protein by Kjeldahl method.
Scanning electron microscopy was performed to determine the three-dimensional structures of each sausage. Cube shaped samples (3 × 3 × 3 mm3) were prepared. Samples were fixed using 2.5% glutaraldehyde solution which was dissolved in 0.1 M sodium phosphate buffer (pH 7) overnight at 4°C. The samples were washed one time using 0.1 M sodium phosphate buffer (pH 7) and immersed in phosphate buffer containing osmium tetroxide for 5 hrs. Thereafter, the samples were washed three times for 10 min with phosphate buffer. Dehydration was performed at increasing ethanol concentrations (50%, 60%, 70%, 80%, 90%, and 100%) at 10 min intervals. Finally, acetone was added for 10 min intervals. The dried samples were gold-coated utilizing a model 108 auto sputter coater (Cressington Scientific Instruments, Watford, UK) and three-dimensional structure was observed by LV-SEM (JSM-6610LV, JEOL, Tokyo, Japan).
RESULTS AND DISCUSSION
As shown in Table 3, pH values were not different among the treatments (p > 0.05). Different salt concentrations didn’t affect the pH of chicken breast sausages (p > 0.05). Lee and Chin [13] reported that low-fat pork sausages with different levels of salt (0.75%, 1.0%, 1.25%, and 1.5%) didn’t differ in pH. Also, restructured pork ham containing salt from 0.5% to 1.5% did not change pH [14]. Aaslyng, Vestergaard and Koch [15] found salt did not affect the pH, regardless of the kind of meat products such as sausage, bacon, ham and salami. In terms of color values, lightness (CIE L*) decreased with increasing salt content (p < 0.05). TRT1 (0.3% salt) had the highest redness (CIE a*) and yellowness (CIE b*) values (p < 0.05). As the salt level increased, the color values tended to decrease. Trout [16] reported sodium chloride in cooked ground beef muscle caused an increase in the denatured myoglobin (%) at the normal cooking temperature of meat and as sodium chloride level increased, denatured myoglobin (%) also increased linearly [16]. Therefore, Trout [16] concluded that addition of salt reduced the pink color of cooked meat products. According to Jeong’s study [17], the CIE L* and b* values with various NaCl levels showed similar trends to our results. He also reported that the addition of salt (except ≥ 2%) might be able to increase CIE a* of cooked chicken breast. However, redness of salt at 0.8% in chicken sausage didn’t differ from 0.3%.
As shown in Table 4, EM (%) was increased with decreasing of salt levels and addition of salt at 1.3% (TRT3) was similar to the higher levels of salt (TRT4, 1.8%; TRT 5, 2.3% salt) (p < 0.05). In fact, TRT 5 (2.3% salt) was the lowest. The results of CL showed similar trends to the EM (Table 4). TRT 4 and 5 had the lowest amount of drip during cooking processing (p < 0.05). According to previous research, increased salt levels of chicken breast gels increased the water-holding capacity, and decreased CL [18]. Another study found the cooking yield of cooked ground chicken breasts tended to increase with increased NaCl level [17]. Lee and Chin [14] previously reported that CL increased in reduced-salt restructured ham. Salt caused the extraction of salt-soluble protein (myofibrillar protein) which is important in emulsifiers and stabilizers in meat products and help to bind meat proteins. In the higher salt level, chloride ions bind to the protein filaments. Therefore, negative charge of filaments increases and causes electrostatic repulsion among the myofibrillar proteins [3,19]. Thus, the adsorption of chloride ions with myosin caused swelling of myofibrils and increased water-holding capacity [3]. In a previous study, the addition of 1% salt reduced chicken muscle shrinkage greatly due to increased water-holding capacity, an effect that depended on phosphate [20].
Table 5 shows that all textural properties of chicken breast sausage except for springiness were affected by various salt levels (p < 0.05). TRT 5 (2.3% salt) showed the highest hardness score (p < 0.05), and TRT 3 (1.3% salt) was similar to TRT 5 on hardness, gumminess and chewiness (p < 0.05). In cohesiveness, TRT 1 (0.3% salt) showed the lowest result, but the others didn’t differ. Tobin et al. [21] reported that salt level affected not only color, sensory and CL, but also texture of frankfurters. And lower salt levels caused increased CL, resulted in lower hardness, springiness, cohesiveness, chewiness and resilience in another study [21]. Similar trends were also observed in the results of Li et al. [18].
As shown in Table 6, pH showed no differences among the formulations without STPP, but addition of 0.3% STPP (TRT 7, 9, 11) caused pH value to increase, regardless of salt addiiton. A previous study reported that STPP increased the pH of chicken patties, but sodium didn’t affect pH if STPP was not incorporated [22]. However, in presence of STPP, sausages with 1.8% sodium (TRT 11) decreased pH slightly as compared to those without salt treatment (TRT 7). In other study, regardless of salt content, addition of 0.4% STPP did not change the pH in raw comminuted beef [23]. Thus, various salt/STPP combination could have interactive effects on pH, depending on their contents.
In color values, lightness (CIE L*) was not different (p > 0.05), but redness (CIE a*) and yellowness (CIE b*) values tended to decrease with the addition of salt and STPP (p < 0.05). In a previous study, salt and phosphate affected the color of cooked pork meat batters [24]. According to the results of proximate analysis (Table 7), moisture content (%) increased with increased levels of salt and STPP (p < 0.05), however, no differences in fat and protein contents (%) were observed (p > 0.05). The reason why moisture was different among treatments was partially due to the increased CL (Table 8). In previous study [25], NaCl/STPP marination resulted in quality improvement of moisture content. And, it also improved water holding capacity and CL [25]. The reduced CL might decrease the moisture content of the sausage, indicating that salt and STPP increased the moisture content of the final meat product. As salt level increased, CL and EM tended to decrease, and the combination of the salt and STPP was even further lower than salt addition alone (Table 8) (p < 0.05). According to the previous study, low salt content (<1.0% and 1.5%) of turkey frankfurters had lower smokehouse yield, as compared to those with high salt (> 2.0%) without STPP [26]. However, regardless of salt levels, addition of phosphate reduced the difference in yield [26]. Villamonte et al. [24] reported that the addition of salt increased the water-holding capacity and decreased CL of the cooked pork meat batters at 3% salt, and polyphosphate reduced the CL without affecting water-holding capacity. Our results showed that salt and STPP in combination affected EM and CL, even though salt level was low. Furthermore, the addition of phosphate resulted in reduced CL and increased water-binding properties [27].
In TPA (Table 9), hardness and springiness tended to increase with increasing salt and STPP levels (p < 0.05). These results of gumminess, chewiness and cohesiveness showed that 1.8% salt and 0.3% STPP combination had the best properties (p < 0.05). In other study of low-fat (1.2%) and single-step high-pressure processed chicken breast sausages, addition of STPP caused a decrease in hardness and chewiness [28]. However, our study turned out to be contrary to these results. Sausage containing 0.3% STPP didn’t decrease the textual properties. Li et al. [18] reported that increasing salt level from 1% to 2% increased TPA and gel strength of chicken meat gels. In addition, 1.5% salt in combination with 0.3% STPP had higher TPA values than those with 1.5% salt alone [29].
Fig. 1 shows the three dimensional microstructure of sausage with various salt and STPP combinations. Regardless of the addition of STPP, the increased levels of salt made the surface flatter and reduced surface pores. Sausages containing 1.8% salt and 0.3% STPP had the flattest surface and the most compact structure. In results of Li et al. [18], there were difference of microstructure according to salt level as it increased from 1% to 2%. One % salt meat gel had a plate-like and disorganized structure with a lot of aggregates and 2% salt formed structure with small pore among the protein strands. However, our results showed that the addition of STPP affected structure to make it plate-like and small pore structures appeared above 0.8% salt. Another study reported that muscle junctions at higher salt and phosphate levels showed a high level of junction alignment and binding properties [30]. During the massaging process, the absence of salt or polyphosphate caused muscle fibers to break, and fragments were observed [31].
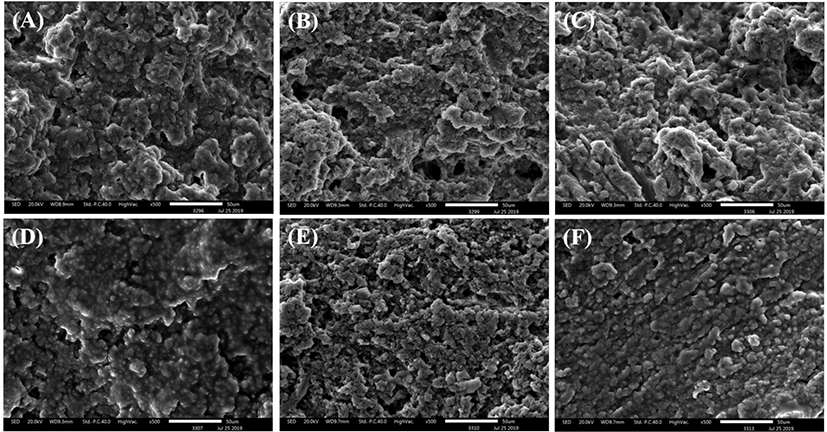
In conclusion, addition of salt and phosphate affects the functionality, textural and physicochemical properties of chicken breast sausages. Although salt or STPP affects the quality of meat products, they are considered to have mutually dependent effects, because these effects based on the difference of concentration and combination of two ingredients. Therefore, meat products should have minimum level of additives during processing, and attempts should be made to reduce salt content (%) with the addition of STPP, resulting in similar characteristics to those with regular-salt meat products. Therefore, STPP addition along with salt will be replaced with partial amounts of salt contents in the manufacture of low-salt meat products.