Background
High temperatures are adverse for pig production because pigs have a low transpiration rate that prevents the animals from regulating their internal temperature and maintaining it within the limits that allow satisfactory rates of production. Pigs use thermoregulation to maintain their body temperature within their thermal neutral zone (18°C to 20°C). Thus, if the environmental temperature exceeds 25°C, pigs are subject to heat stress [1].
Moreover, gestation and lactation are both critical stages in which sows are more susceptible to heat stress. Several studies have reported that sows subjected to temperatures above their thermal neutral zone (≥ 25°C–35°C) under controlled conditions showed negative effects on reproductive performance, including decreased litter size and an increase in the time interval between weaning and the next litter. Furthermore, the restoration normal body temperature of the sows is irregular [2–4].
In addition, it has been observed that heat stress caused by temperatures above 30°C, can reduce the milk yield of sows [5,6], and in some components of milk and colostrum such as fatty acids and immunoglobulins can be diminished [7,8]. This decrease in the fatty acid content can affect the primary source of energy (i.e., the colostrum) during the first hours of life; also, the piglet acquires its immunity passively through colostrum, which is the primary source of protection against pathogens [9].
Furthermore, high temperatures can also affect the concentrations of vitamin A and vitamin E, among other nutrients. Vitamin E is the main antioxidant that protects cells against the free radicals that can generate oxidative stress. Vitamin A also works as an antioxidant, but its main effect is on the development of the embryo and foetus during the reproductive stage. During gestation and lactation, there is an increase in metabolic loads that cause significant systemic oxidative stress, which is related to the decrease in the availability of antioxidants such as vitamins A and E [10]. It has also been shown that stress plays a role in the physiology of infertility [11].
There are reports where the effect of heat stress on the parameters mentioned above was evaluated under conditions of controlled temperatures that were no higher than 35°C. However, it is important to evaluate how reproductive performance, the serum levels of vitamins A and E and the concentration of immunoglobulins during the gestation and lactation of sows and in their litters are affected under commercial husbandry in regions where environmental temperatures reach 40°C–50°C [hot environments with high relative humidity (HR)], which are considered challenging environmental conditions.
Materials and Methods
All procedures involving animal handling were performed within the official Mexican guidelines for animal care (NOM-EM-051-ZOO-1995) [12]. The humane care of animals was ensured during immobilization.
The experiment was conducted on a commercial farm where there were no special provisions to control the environmental conditions of the experimental animals. The pig farm was located in the southern portion of the state of Sonora, Mexico (27° 04’ 51” N, 109° 26’ 43” W). The sample analyses were conducted at the Research Centre for Food and Development (CIAD).
A total of 14 crossbred sows (Large White × Landrace) were used with parities ranging from 1 to 7. It is important to note that this wide range of parity occurred because the experiment was conducted under commercial conditions. The sows were evaluated at two different times. The sows selected had their gestation period during the winter-spring season and were evaluated upon completion of gestation during the summer season. The experiment lasted until weaning (21±3 days).
During gestation, the sows were given a diet of 3340 MCal / kg of metabolizable energy per day based on the recommendations of the NRC [13]. Due to farm management practices and seasonal effects, the diets were formulated with different energy contents for the summer and winter seasons (Table 1). During the lactation period, the sows were fed 4 kg of the lactation diet during the first seven days and 7 kg of feed during the remaining days of lactation. The feed was given once a day prior to parturition and twice a day after parturition. Water was available ad libitum during pregnancy and lactation.
During gestation, the sows were housed in an open sided area, in individual crates. The sides of gestation area could be covered with curtains to block out the sun and when the temperature fell. Foogers and forced ventilation were used to control temperature and humidity in the area particularly in summer. On day 110 of gestation each sow was moved to a farrowing house. Each farrowing house has a cooling system, a heat exchanger with recirculated water to control the temperature during summer.
The parameters recorded for each litter immediately postpartum were the number of live and stillborn piglets and mummies; the number of piglets alive and dead at weaning was also documented. The weight of individual piglets was recorded at birth and on the day of weaning (21 d of age).
Milk yield was calculated using an equation reported by Ferreira et al. [14], which includes the weight gain of the piglets (weight at 21 of age – weight at birth), the number of piglets per litter and the number of days of lactation:
where PWGP = piglet weight gain during the period. The value 4.27 is a constant that assumes that for each kilogram of litter weight gain, the sow produces 4.27 litres of milk [14].
Backfat was measured at the beginning of the experiment and at weaning using ultrasound PigLog105 (SFK-Technology Ud., Herlev, Denmark); the measurement was taken at the last rib, 65 mm from the dorsal line. The data were expressed in inches.
Blood samples were collected from the sows and from the piglets for the determination of vitamins A and E, IgG and IgA in serum. Sampling for piglet serum at day 0 occurred twelve hours after colostrum intake and on day 21 (at weaning). Samples of colostrum and milk were obtained for the quantification of vitamins A and E, and immunoglobulins IgG and IgA. Fig. 1 shows the sample collection scheme for sows and piglets sampled for colostrum, milk and blood.
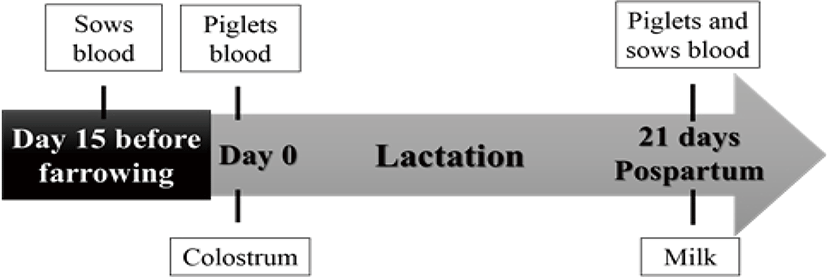
Different extraction techniques were used to quantify the vitamin E and vitamin A in serum, colostrum, and feed. For serum, the extraction of vitamins A and E followed the method described by Hess et al. [15]. For colostrum and in milk, the method used was described by Hidiroglou [16]. After the saponification of the feed, the extraction was conducted as described by Onibi et al. [17]. The quantification of vitamin E and vitamin A were conducted using an HPLC system equipped with a diode array detector (1260 Infinity, Agilent Technologies, Santa Clara, CA, USA) with a C18 Agilent Microsorb (100-3 C18, 100 × 4.6 mm) column. The mobile phase was isocratic [methanol: water (98:2, v/v)] at a wavelength of 325 nm and 290 nm for vitamin A and vitamin E, respectively. Purified standards (Sigma-Aldrich, St. Louis, MO, USA) were used to calculate vitamin A and vitamin E concentrations, which were expressed by volume (µg/mL).
IgG and IgA in the serum of piglets and in the colostrum and milk from sows were quantified by a sandwich enzyme-linked immunosorbent assay (ELISA). Kit Pig IgG ELISA (E101-104, Bethyl Laboratories, Montgomery, TX) and an ELISA Kit Pig IgA (E101-102, Bethyl Laboratories, Montgomery, TX), were used with slight modifications. The absorbance was measured at 450 nm using a Model 680 microplate reader (Bio-Rad Laboratories, Tokyo JP).
We used the temperature and humidity index (THI) as an indirect measure to determine whether the sows were subject to heat stress. This index uses environmental temperature and HR as an indicator of heat stress [1]. This index was used during gestation for both the summer and winter seasons. The environmental temperatures and HR were recorded using a HOBO data logger, Model H-8 (Bourne, MA, USA), installed in the gestation area, which is an open area. The THI values were calculated using the following equation [18].
where:
T: temperature (°C)
HR: relative humidity (%)
The relationship between THI and heat stress was as follows: if the score derived from the equation was 67 to 70, there was moderate heat stress; from 71 to 76 was considered severe heat stress and a value greater than or equal to 77 was considered extreme heat stress (ThermoTool, Group CCPA, Janze, France).
A longitudinal study was conducted using the same pregnant sows from winter to summer. An analysis of variance was performed using general linear models procedure (GLM ANOVA) with the season as a fixed effect, parity and milk production as covariates, to determinate the effect by season, for initial and final weight in the piglets, litter size (birth and weaning), live born piglets, dead piglets at weaning. For milk yield, the model include the parity and initial back fat thickness as a covariate. For vitamins and immunoglobulins content in colostrum, milk and serum the GLM ANOVA was used, including season as fixed effect and baseline levels as covariates. Data of stillborn and mummies were evaluated using proportion test to estimate the effect of season. The results were considered significant if the level of probability of the Type I error was less than or equal to 0.05. Data analyses were performed in the statistical package NCSS version 2007 (Number Cruncher Statistical System for Windows, Kaysville, UT, USA).
Results
The THI for the winter and summer seasons is shown in Fig. 2. The months from November to March were considered winter, and the lowest THI was recorded in January (Fig. 2a). The average temperature was 17.52 ± 6.70°C, and the average HR was 62.59 ± 21.80%. The THI calculated during these months is shown in Fig. 2a; the values obtained during this time showed that the sows were not subject to heat stress, because the monthly average THI of each month during pregnancy was below 67. In addition, Fig. 2b shows the THI of the coldest and hottest hour of the day during the month of January, which was the coldest month during this season (57. 86 ± 1.53 THI, on average).
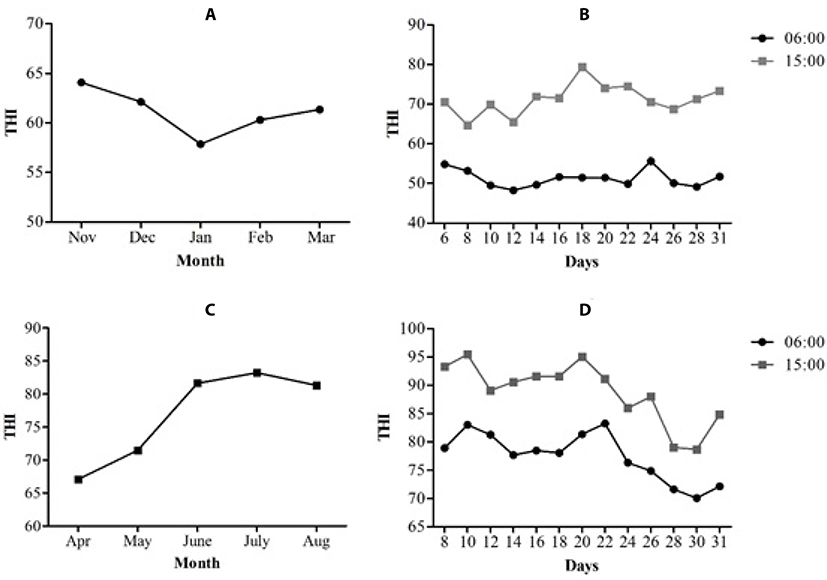
During the months that corresponded to the summer season, from April to August, the average temperature was 33.7 4.90°C, and the average HR was 52.47 ± 15.80%. At the end of April, gestation began and the observed THI was 67 ± 1.43, which is not considered heat stress, whereas May had a THI of 71.47 ± 3.26, which indicated severe heat stress. During the months of June, July and August the THI values were greater than 80 ± 7.50; this indicates extreme heat stress (Fig. 2c). The month of July showed the highest THI of the summer (83.21 ± 3.92). The THI was also calculated for the coldest hour of the day (6:00; THI 78 ± 3.45, on average) and the warmest hour (15:00; THI 89 ± 4.80) for the month of July, both of which were classified as extreme heat stress (Fig. 2d). On the farm, owners use foggers in gestation area and cooling system in farrowing house in the summer season as a strategy to mitigate high temperatures. However, the humidity contributes to some extent to the stress that occurs during this time.
Table 2 shows the parameters of reproductive performance for each season. Although the winter season had a higher number of piglets per litter compared with the summer season, when the proportions of these reproductive parameters were analysed, no statistical difference was found between the seasons (p ≥ 0.05). The proportion of live-born piglets was 93.8% and 91.9% in summer and winter, respectively (p ≥ 0.05).
In addition, Table 2 shows the weights of the piglets at birth and at weaning in the two seasons. No significant differences were found between summer and winter (p ≥ 0.05). The weights recorded were within the range of average weights based on previous studies [19]. Those authors conducted a study of the survival of newborn piglets and suggested that piglets with a birth weight of < 1.22 kg were of low weight and those with a birth weight of 1.42 kg were average, which is similar to the results obtained for both seasons in this study.
There were no differences in milk yield between winter and summer (p ≥ 0.05) see Table 2. However, in summer, there was a decrease in milk yield of 0.84 kg/day, which is physiologically important. It is important to note that this tendency for a decreased milk yield would have been more significant if the groups had included a larger number of sows in the experiment; unfortunately, our experiment was conducted under commercial conditions, and this was the number of sows available. Nevertheless, these results show a clear decrease in milk production during the summer.
The body condition of the sows was evaluated by measuring the dorsal fat thickness. Fig. 3 shows the dorsal fat thickness of the sows before at day 100 of gestation (initial) and at 21 days postpartum (final) for both seasons, and no differences (p ≥ 0.05) were found between seasons; the initial and final values of the sows were also within the normal range (0.67–0.78 in) as reported Coffey et al. [20].
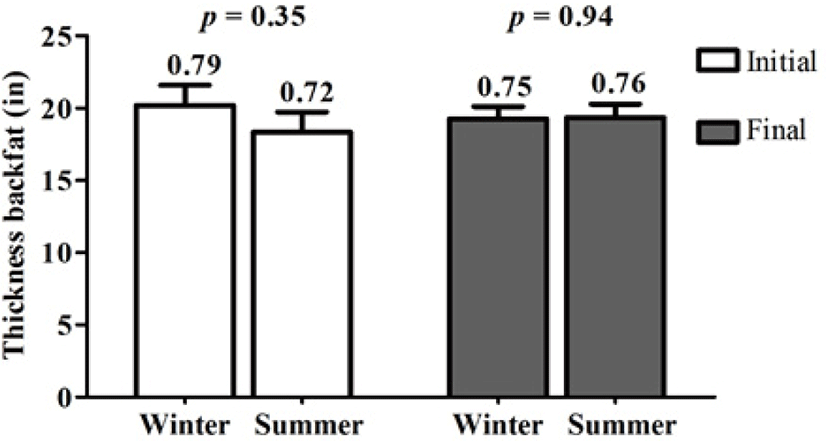
Table 1 shows the vitamin A and E content in the diets of pregnant and lactating sows for both seasons. The vitamin E content of the feed for these stages was below the recommendation of 44 mg/kg of feed, whereas the vitamin A content was approximately equivalent to the recommended value, i.e., 1.2 mg/kg of feed during gestation and 0.6 mg/kg of feed during lactation [21].
The vitamin E and vitamin A content in the serum of sows at day 100 of gestation and at 21 days postpartum is shown in Table 3. The vitamin E content in sow serum was lower in summer than in winter at 100 days of gestation (p < 0.001); however, at day 21 post-partum there was no difference between the seasons. In contrast, the vitamin A content was lower (p < 0.001) in summer than in winter at 21 days postpartum, and no differences were found between winter and summer at day 100 of gestation. Table 3 also shows the vitamin E and A content in colostrum and milk. There was no effect of season for colostrum (p ≥ 0.05). In contrast, milk had an increased vitamin E content in winter compared with summer (p < 0.001). There were no significant differences in vitamin A content between seasons for either colostrum or milk (Table 3).
The vitamin E and vitamin A content of piglet serum is shown in Table 4. The concentrations of vitamins A and E in the serum of newborn piglets 12 h after consuming colostrum were significantly higher in summer than in winter. At 21 days after birth, there were no differences in the contents of either vitamin between seasons.
The immunoglobulin (IgG and IgA) values in colostrum and milk by season are shown in Table 5; there was no effect (p > 0.05) of season for IgG and IgA concentrations. However, in milk, there was a tendency (p = 0.07) towards a decreased IgA content in the summer compared with winter. The IgG content in piglet serum at day 0 (at birth, 12 h after consuming colostrum) and at 21 days of age was not different between seasons. However, the IgA content in piglet serum was lower in summer (p < 0.001) compared with winter at day 0 and at day 21. In addition, there was an effect of parity on immunoglobulin values; the difference is because this study was conducted under commercial conditions and was adapted to farm management, thus the parity effect could not be controlled. We found that parity had an effect (p < 0.001) on colostrum IgG concentration (Table 5). First parity sows had lower (p < 0.001) colostrum IgG concentrations when compared with sows with 2 or more parities. Moreover, parity had an effect (p < 0.01) on the IgA content of milk.
Discussion
In the two groups of pregnant sows studied under conditions of commercial confinement during different seasons (winter and summer), we found that sows were subject to moderate to severe heat stress in summer but not in winter, based on the THI indexes obtained.
Under changing climate conditions, the occurrence of high temperatures and their intensification in arid regions could affect some production parameters in pigs, primarily during the reproductive stage under commercial conditions, even if the animals were adapted and housed in installations designed to ameliorate these extreme temperatures [22]. Moreover, the genetic variability both within and among pig populations has an effect on heat tolerance. Gourdine et al. [23] reported that Large White sows have some thermoregulatory traits that allow them to tolerate heat stress and mitigate its effects on health.
During gestation, high temperatures and high HR promote the impairment of some health parameters, and; thus, a lack of constant monitoring and management of environmental factors such as temperature and humidity could be reflected in production losses, which translate into economic losses.
Despite the non-significance on the parameters of reproductive performance between seasons, our data showed a lower number of live-born piglets (135 vs 157) and weaned pigs (123 vs 141) in summer than in winter, which implies an impact on production and economic losses in the summer season. Moreover, a greater number of piglets were born dead or as mummies in the summer than in the winter season, as well as, a smaller number of piglets at weaning in the summer season (p > 0.05). However, from the standpoint of production, this decrease in weaned animals is important, reflecting economic losses at the production level; for example, in the United States, the mortality of sows and growing pigs leads to an annual loss of $300 million [24]. We estimate the economic losses in the farm, extrapolating the number of piglets weaned from the 14 sows to the total (400) in both seasons, and the losses in summer season would reach 514 piglets representing 85,000 US dollars approximately. This estimation value was calculated based on the hogs average price for slaughter, 1.49 US dollar per kilogram live weight, considering 110 kg of weigh per animal to slaughter [25]. Most of the reports, regarding the effect of heat stress involve temperatures from 30°C–35°C and low HR, or they are related to animals that have not been adapted to a wide range of temperatures. In a review by Bianca [26], the author mentions that warm temperatures (34°C) favour the development of the piglet during lactation; however, our results showed that at warmer temperatures, an increase in mortality (9.2% in summer vs 8.1% in winter) was observed.
With regard to milk production, although the values were not significantly different between winter and summer, summer production was lower. From a physiological standpoint, this decrease could be important because there was a loss of 0.84 kg/day of milk. These results are consistent with those reported by Silva et al. [5], who observed that when lactating sows were exposed to heat stress (29.4°C), they produced 1 kg less of milk per day than those at 20°C. Silva et al. [27] found a similar effect when sows were placed in an environment similar to their thermoneutral zone (a cold floor at 17°C). Sows without this cooling system had a milk production of 8.05 kg / day compared with 10.20 kg / day for sows with this cooling system.
Additionally, the studies conducted by Silva et al. [27] and Williams et al. [28] also reported a decrease in milk production under conditions of high controlled temperatures, and they suggested that this is related to a thinner layer of back fat before parturition. Although there were no significant differences in the present study, lower milk production will undoubtedly result in decreased energy consumption by the piglet and will probably result in a loss of body weight. The lower the weight of the piglets at weaning, the more difficult it will be to achieve the final desired weight of the pig, which will require a greater investment in both time and feed for the piglet to reach the weight required for slaughter and sale.
In contrast to our results, the marked differences in reproductive performance parameters such as milk yield, live weight of piglets and mortality reported in other studies when the animals were above their thermoneutral zone occurred because those studies were conducted under controlled environmental temperatures to which the animals were not well adapted. In contrast, our experiment under commercial husbandry conditions did not control environmental temperatures, and the animals were adapted to higher temperatures.
The vitamin E content was lower in the summer diet than in the winter diet, and it was lower than the level recommended by the NRC [21], which recommends an increase to at least 50 mg / kg feed in combination with higher quality fatty acids in the summer; this value is recommended because during this time, greater oxidation of fatty acids and vitamin E occurs, although the feed typically contains antioxidants to prevent oxidation.
Our results showed a lower concentration of vitamins E and A in the serum and milk of sows during the summer; however, these data are within the reported range of animals exposed to temperatures below those in our experiment, with the exception of those reported by Pinelli-Saavedra [29], who worked under conditions similar to our experiment (above 40°C in the summer season). These low values of vitamin E in serum and milk could be attributed to heat stress in the sows, because heat stress can produce oxidative stress [10] and the reduction in vitamin E levels in the serum could then be reflected in the vitamin E content of the milk.
The values of vitamin A and vitamin E in colostrum, milk and serum observed in our study are similar to those reported by other authors [29–33].
The high vitamin A and vitamin E content in piglet serum at birth during the summer was unexpected. We hypothesized that because their thermoneutral zone was 34°C, newborn piglets subjected to warm temperatures would rapidly acquire mobility, find the nipples of their dam and begin to consume the initial colostrum secretions, which contain a high concentration of nutrients, including vitamins A and E. In contrast, when the ambient temperature drops, the piglets pile up together to retain heat; they exhibit chills and require more time for colostrum intake [1,22,26]. Despite these seasonal differences in behaviour observed at birth, seasonal differences in vitamins levels were not observed at weaning. In the case of vitamin E, this could be because the milk offered by the dams was higher in vitamin E during the winter. Moreover, it is clear that colostrum and milk strongly influence the vitamin E content in piglet serum at weaning. With regard to vitamin A, no differences were found between seasons and the environmental conditions also helped. The values of vitamins A and E in piglet serum for both seasons and the sampling times were consistent with those reported by Pinelli-Saavedra [29], Håkansson et al. [31], Debier et al. [32] and Sivertsen et al. [34].
Passive immunity from the sow’s mammary gland secretions is transferred to piglets through colostrum and milk and is an important form of protection to improve the survival of progeny. In this study, one of the aims was to evaluate piglet serum immunoglobulin content under commercial husbandry conditions. Therefore, our study was adapted to the farm management, and we included a mixed population of primiparous and multiparous sows. We found an effect of parity on colostrum IgG and on IgA in milk. Both immunoglobulins had higher values in multiparous than in primiparous sows. The IgG content of colostrum obtained in our study is consistent with the values reported by Cabrera et al. [35], who found that parity had a significant (p < 0.001) effect on colostrum IgG concentration and was 5% higher in multiparous sows than in primiparous counterparts. With regard to the effect of parity on IgA in colostrum and milk, our data are consistent with those reported by Leblois et al. [36], who reported that parity had an effect on the IgA content in milk but not in colostrum.
However, there is little information related to the effect of hot environmental conditions on the immunoglobulin content in colostrum, milk and piglet serum; this is a critical stage of immunological development when piglets are dependent upon maternal antibodies to fight potential infectious agents. Our results showed a significant decrease in the level of IgA in colostrum and milk (p < 0.05), and although there was not a significant seasonal difference, there was a decrease in IgG in colostrum during the summer (35.42 vs 36.30 mg/mL). One possible explanation is that the physiological effects of heat stress increase the production of reactive oxygen species (ROS), which increases the overall load in the body [37]. Immune cells are susceptible to damage by ROS, thus immunoglobulin production by plasma cells could be impaired.
Regarding the effect of season on immunoglobulins in piglet serum, we observed that IgA values were significantly lower in summer compared with winter, and although the same pattern was observed, the effect was not significant for IgG. IgA antibodies are important at the intestinal barrier as an immunoexclusion mechanism of antigens and help to protect against infectious agents. A possible explanation for the decrease in both immunoglobulins could be due to the reduction in colostrum intake, which reduced the concentration of colostrum-derived antibodies in the newborn piglets. Additionally, Machado-Neto et al. [8], reported that when sows were exposed to heat a few days before and during parturition, their piglets showed a significant decrease in plasma immunoglobulins. This detrimental effect might be caused directly by the inhibition of absorption of immunoglobulins by cortisol, but it is also possible that the piglets born from heat-stressed sows consumed less colostrum [38].
Another aspect to consider is that there may be significant variation in the concentration of immunoglobulins among different animals from the same litter, because the concentration of immunoglobulins depends on factors such as the amount of colostrum available, the level of consumption by the piglet and the speed with which they consume the initial secretions of colostrum [9,39]. In addition, a reduction in the vitamin A serum concentration in postpartum sows might explain the lower IgA concentration in milk and in the serum of weaned piglets, when passive immunity, as well as the piglet’s own antibody production, is important, because retinoic acid (a vitamin A metabolite) is involved in mucosal IgA production [40].
Regarding the limitations of this study as it was conducted in a commercial farm, we had to adapt to management of the farm, thus, we could not control the range of parity, the number of sows used and the environmental temperatures and HR only the strategies used in the farm to ameliorate the adverse effects of severe high temperatures. Despite of this, we found a negative effect of hot weather in some parameters such as vitamin E and vitamin A contents in sows serum and milk and Ig A in piglets.
Conclusion
Despite the pregnant sows experienced heat stress in extremely hot weather according to the THI values obtained, our experiment under commercial husbandry conditions showed that the animals seem to be adapted to higher temperatures due to the strategies used from the farm to ameliorate the adverse effects of hot weather. However, we found that some parameters measured were affected in the summer season. We found the hot weather had a negative effect on the serum levels of vitamin E and vitamin A in sows at the gestation stage, and it affected their milk content. Moreover, the concentrations of IgA in piglet serum at birth and weaning were decreased in summer; this could compromise intestinal health and make them susceptible to infectious diseases.
Regarding reproductive performance there was no significant effect of summer, but an economic impact can be demonstrated at the production level because there was a smaller number of live piglets per litter, both at birth and at weaning, and a decrease in milk production, which may have contributed to the lower number of weaned piglets in summer.
The results of this study are therefore relevant to practical pig production in hot climates and may guide the search for future strategies that mitigate the impact of thermal stress on nutritional and immune status and on pig production performance.